the Creative Commons Attribution 4.0 License.
the Creative Commons Attribution 4.0 License.
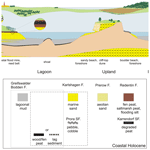
The lithostratigraphic formations of the coastal Holocene in NE Germany – a synthesis
Lampe, R.: The lithostratigraphic formations of the coastal Holocene in NE Germany – a synthesis, E&G Quaternary Sci. J., 71, 249–265, https://doi.org/10.5194/egqsj-71-249-2022, 2022.
Based on extensive investigations along the coast and in the coastal waters of NE Germany, a lithostratigraphic classification of the Holocene coastal deposits is presented. Their characteristics, i.e. the lithofacies, reflect the spatial change in hydrodynamics, sediment supply, salinity, bioproduction, etc. in the accumulation space. The displacement of the facies associated with the sea-level rise of the Baltic Sea led to the formation of regularly occurring vertical depositional sequences. From these regular profiles, four lithostratigraphic formations and two subformations of the coastal deposits can be delineated as approximately homogeneous sedimentary bodies, which are described in detail, defined in terms of their spatial extent and classified with regard to the time of accumulation.
Auf der Grundlage umfangreicher Untersuchungen entlang der Küste und in den inneren Küstengewässern NE-Deutschlands wird eine lithostratigraphische Gliederung der holozänen Küstenablagerungen vorgestellt. Deren Merkmale, d.h. die Lithofazies, spiegeln den räumlichen Wandel der Hydrodynamik, Sedimentzufuhr, Salinität, Bioproduktion, etc. im Akkumulationsraum wider. Die mit dem Meeresspiegelanstieg der Ostsee einhergehende Verlagerung der Fazies führte zur Herausbildung regelhaft auftretender vertikaler Depositionssequenzen. Aus diesen Regelprofilen lassen sich als annähernd homogene Sedimentkörper vier lithostratigraphische Formationen und zwei Subformationen des Küstenholozäns ausgliedern, die detailliert beschrieben, in ihrer räumlichen Ausdehnung definiert und deren Akkumulation zeitlich eingestuft werden.
- Article
(13824 KB) -
Supplement
(4351 KB) - BibTeX
- EndNote
Lithostratigraphy is the branch of stratigraphy that correlates rock units exclusively on the basis of rock properties (lithofacies) and their changes in space (within a sedimentation area) and time (within the vertical profile). Any fossil content is taken into account as a rock property. According to the stratigraphic principle, the lithofacies of coastal sediments reflect environmental conditions such as hydrodynamics, salinity and bioproductivity in the accumulation areas and trace their temporal changes and spatial displacements due to shoreline shifts, which can be caused by an unbalanced sediment budget but mainly by rising or falling sea levels (Kraft, 1978).
The basic unit in the lithostratigraphic hierarchy is the formation, which is defined by its lithology, its internal heterogeneity/anisotropy and facies-change-related boundaries. Since the properties of formations are identifiable with field methods and because they can be traced in the landscape without any additional stratigraphic information, they build the primary mapping units in geological surveys (Ad-hoc-Arbeitsgruppe Geologie, 2002). For land-use planning, construction, mineral resource exploitation, water management, and coastal and landscape conservation, it is important to know the spatial distribution of geological and geotechnical properties in the sub-ground. Maps of formations reflect this information and mirror the distribution of the parameters needed for planning purposes (Weerts et al., 2005).
According to Remane et al. (2005), a formation represents a lithologically sufficiently homogeneous rock unit that can be mapped on the ground surface or in the subsurface and represented at a scale of at least 1:10 000. Terrain morphological criteria can also be taken into account. The boundaries of the formation are determined by a distinct change in lithological characteristics, which are usually diachronic due to the spatial displacement of the sedimentation areas. Formations can be grouped together on account of common lithological features. It is also possible to separate subformations due to their specific characteristics.
This paper deals with the lithostratigraphic formations and subformations of the coastal Holocene along the SW Baltic shoreline in Mecklenburg-Vorpommern. Although the term “coastal Holocene” is commonly used, no explicit definition exists. Therefore, the following definition is here proposed: the coastal Holocene comprises the subaqueous and subaerial accumulations whose formation is determined by littoral processes and brackish-marine environments. In the subaqueous part of the coast, those depositions are to be considered which accumulated above a critical water depth where wind waves cause sediment transport due to bottom contact. In the subaerial part, all accumulations count as coastal Holocene which formed directly under the influence of seawater or under environmental conditions which are characteristic for the proximity to the sea (wind regime, salt and sediment inputs, flooding).
On the coast of Mecklenburg-Vorpommern, the critical water depth is about 10 m (Ministerium für Landwirtschaft, Umwelt und Verbraucherschutz Mecklenburg-Vorpommern, 2009). Since the Holocene global sea-level rise reached the Baltic Sea basin around 8800 years ago, the water level has risen by about 25 m (Niedermeyer et al., 2011). Therefore, coastal Holocene sediments are also to be expected beyond the current 10 m isobath. Although comprehensive seabed data are available (Bundesamt für Seeschifffahrt und Hydrographie, 2012, 2016), they only reflect the conditions close to the ground surface. Since the sediments and their bounding surfaces in the deeper sub-ground remain widely unknown, such data are not sufficient for the definition of formations. The explanations presented in this paper are therefore limited to the near-coastal area, which is comparatively better explored (Fig. 1).
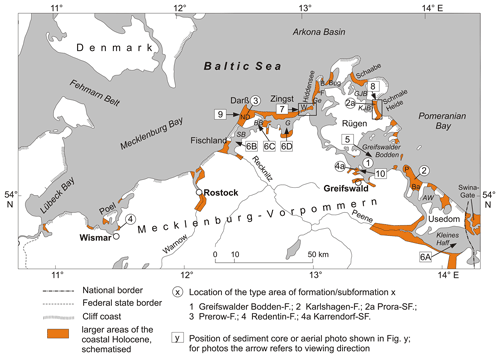
Figure 1Occurrences of larger coherent areas of the coastal Holocene in Mecklenburg-Vorpommern (schematised), locations of the type areas of the formations and subformations, and the sediment cores and photos used for their description. SB: Saaler Bodden; BB: Bodstedter Bodden; G: Grabow; GJB: Großer Jasmunder Bodden; KJB: Kleiner Jasmunder Bodden; AW: Achterwasser; ND: Neudarß; W: Werder islands and sandflat; Ge: Gellen and Gellenschaar; F: Fährinsel; B: Bessin and Bessinsche Schaar; P: Peenemünder Haken; Ba: Banneminer Niederung.
In the past, studies to subdivide the German coastal Holocene lithostratigraphically were made only for the North Sea coast of Lower Saxony (Brand et al., 1965; Sindowski, 1968; Barckhausen et al., 1977; Streif, 1979). The underlying principles, however, are hardly transferable to the North Frisian coast (Ahrendt, 2006) and not applicable to the Baltic Sea coast due to different sediment layering. Further, the studies mentioned do not include any aeolian accumulations, which are important coastal landforms along the SW Baltic region (Niedermeyer et al., 2011). For the coast of Mecklenburg-Vorpommern a spatial representation of lithologically defined units was given by the Quaternary lithofacies map 1:50 000 LKQ50 (Cepek, 1999) produced in the German Democratic Republic (GDR). As the map series was designed for the entire national territory, the coastal Holocene as such is not shown separately but is integrated into the Holocene horizon map and shown with signatures as marine brackish, telmatic or aeolian accumulation and differentiated as gravel, sand, silt and clay, marine or limnic mud, fen peat, or aeolian sand.
Currently, the descriptions of lithostratigraphic units used for geological maps in Germany are collected in a unified way. This posed the challenge to subdivide the coastal Holocene into formal units that correspond to the criteria of the lithostratigraphic lexicon LithoLex. LithoLex – a joint project of the German Stratigraphic Commission and the Federal Institute for Geosciences and Natural Resources (DSK and BGR, 2021) – was initiated in 1999 as a collection that records all defined lithostratigraphic units of Germany. Since 2006 LithoLex has established a dynamic database which is publicly accessible via the internet. It complements the Stratigraphic Table of Germany 2016 (STD 2016) and should provide descriptions of all units mentioned there, and these are also the mapping units of the geological maps of the state geological surveys.
The formations of the coastal Holocene on the SW Baltic coast were defined and evaluated by the Subcommission on Quaternary Stratigraphy for the first time in 2014. However, the standard forms used in LithoLex allow only short descriptions which need to be amplified and explained separately.
Therefore, the aim of the paper is
-
to summarise the available lithological information by defining homogeneous sedimentary units (formations), describing their general lithological properties, their occurrence, boundaries, mean thickness and spatial interrelations;
-
to relate the accumulation of these units to specific phases of the regional Baltic sea-level development and to show their genetic and chronostratigraphic correlations;
-
to contribute an exemplary solution within the efforts for a comprehensive and uniform definition of lithostratigraphic units in Germany (LithoLex) as a basis for future geological maps.
The definitions and descriptions of the coastal formations of the SW Baltic region rely mainly on data from investigations which were conducted at the University of Greifswald during the past decades, among them unpublished project reports and diploma and PhD theses. They document more than 600 descriptions of on- and offshore sampled sediment cores (sampled onshore mostly with motor-hammer-driven core devices and offshore with shipborne vibrocorer or gravity corers) and related lithological investigations (colour, grain size distribution by sieving or with a laser particle sizer, content of organic matter as loss on ignition (LOI) at 550 ∘C, carbonate according to Scheibler or as the difference of LOI at 1100 ∘C and LOI at 550 ∘C, trace elements using X-ray fluorescence spectroscopy (XRF), or inductively coupled plasma (ICP), as well as macroscopically identifiable remains of organisms), which were used to derive the general lithology of the coastal sediment bodies and to gain cross sections of the coastal landforms. In some cases ground penetrating radar (GPR) or sediment echo sounder records provide supplementary information regarding the spatial layering. Additionally, for many samples or cores radiocarbon or optically stimulated luminescence (OSL) datings and analyses of pollen, diatoms, molluscs or macro-remains are available which provide information about the deposition period, environmental conditions, accumulation rates and human impact. Almost all of these investigations were conducted to reconstruct the sea-level development and/or the evolution of the coastal landscape.
From the lithological and topographical information contained in these data, supplemented by the data documented in the drilling data repository of the State Geological Survey of Mecklenburg-Vorpommern, the general layering structure of the coastal morphogenetic units, such as strandplain, coastal mire, barrier, lagoonal infill, etc., is known, and from this a useful classification into lithostratigraphical formations can be derived. The characteristic of a formation is essentially determined by its position in the accumulation space relative to the shoreline. This position might be temporarily shifted due to sea-level or sediment budget variations, and thus, the formations as pure lithostratigraphic units can be linked to chronostratigraphy to some extent.
With few exceptions, the morphogenetic framework for the development of today's coastal landscape was formed during the Weichselian Glacial. The sediment and relief legacy of this glaciation determined the spatial distribution of the elevations and depressions of the ground surface, as well as the type and volume of the sediment that built up the later coastal landforms and is responsible for the development of regionally predominant coastal types (Hurtig, 1957). After the deglaciation of northern Germany about 14 500 years ago, the present coastal area remained under terrestrial-limnic conditions until the Atlantic (Kolp, 1976; Verse et al., 1998; Schumacher and Bayerl, 1999). The precursors of the Baltic Sea – the Baltic Ice Lake, the Yoldia Sea and the Ancylus Lake – did not exceed a level of −18 m NHN (standard elevation zero) according to present knowledge (Lemke, 1998; Lampe et al., 2005); only sediments of local waters have survived. Therefore, in today's coastal area, deposits of the coastal Holocene are always separated from those of the terrestrial-limnic Late Pleistocene or Early Holocene by an erosional unconformity.
The development of the coastal landscape started with the Littorina transgression, i.e. the time when the postglacially rising ocean flooded the connecting sounds between the Baltic Sea and the Kattegat and entered the Baltic Sea basin (Fig. 2). There are differing opinions about the exact time. Rößler et al. (2011) give an age of 8 ka b2k (all ages are converted to millennia before 2000 CE (ka b2k) and rounded) for the onset of marine sedimentation in the Mecklenburg Bay and 7.2 ka b2k for the Arkona Basin, while Andrén et al. (2000) found an age of 7.8 ka b2k for the Bornholm Basin. For today's coastal areas, however, most researchers describe marine sediments from as early as 8.8–8.0 ka b2k (e.g. Kliewe and Janke, 1982; Berglund et al., 2005; Lampe et al., 2010; Rosentau et al., 2021).
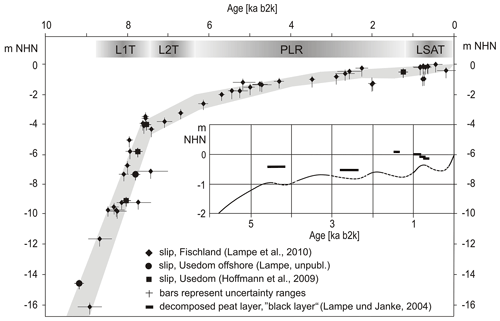
Figure 2Relative sea-level curve for the Fischland and northern Usedom coastal sections (see Fig. 1). L1T/L2T: Littorina-1/Littorina-2 Transgression; PLR: Post-Littorina Retardation; LSAT: Late Sub-Atlantic Transgression; for explanation see text. Due to different glacio-isostatic movements, the curve is flatter north of Fischland and Usedom and steeper to the south (see Lampe et al., 2010). For the period after 6 ka b2k, a curve derived from degraded peat layers in the coastal mires is additionally given, which better reflects the small-scale sea-level fluctuations (after Lampe and Janke, 2004; modified). Dashed curve sections mark periods of sea-level fall as deduced from degraded peat layers. The amplitude of the lowerings is not known. For a detailed description of the sea-level index points (slip) see the database in Rosentau et al. (2021).
The further development of sea level is also interpreted somewhat differently depending on the investigator. In general, however, it can be divided into four phases, which are characterised by different rates of rise and the formation of characteristic coastal landscape units (Niedermeyer et al., 2011). Depending on the regional context and the dating methods used, the information on the time intervals of the phases can vary by several hundred years; the following information follows Lampe and Lampe (2021).
During the Littorina-1 Transgression (L1T; Fig. 2), ca. 8.8–7.5 ka b2k, a very rapid water-level rise occurred at a rate of 6–9 mm a−1 on average; for more precise data, the regionally varying isostatic movements of the coastal sections must be taken into account (Dietrich and Liebsch, 2000). The abrasion of the inundated land areas remained relatively low, it can rather be assumed that the landscape drowned (cf. Schwarzer et al., 2019). In the depressions of the present-day lagoons (Bodden), which were protected by surrounding Pleistocene uplands, mud accumulated rich in shell hash. Over the Littorina-2 Transgression (L2T), ca. 7.5–6.3 ka b2k with a gradual transition to the next phase, the rate of rise decreased to about 1–2 mm a−1. This was associated regionally with an increase in abrasion and locally with the filling of the near-shore submarine areas with shallow-water sands. Towards the end of the phase, the first stable coastal landforms emerged. The Post-Littorina Retardation (PLR), ca. 6.3–1.2 ka b2k, was characterised by a largely stable sea level, but several decimetre-scale fluctuations were found (Fig. 2). The phases of falling or at least stagnating sea level coincide with known climate variations such as the Homeric Minimum (ca. 2.9–2.6 ka b2k; Martin-Puertas et al., 2012; Mayewski et al., 2004) and the Dark Age Cold Period (ca. 1.6–1.2 ka b2k; Helama et al., 2017). The mean rate of rise was about 0.2–0.4 mm a−1. During this phase, coastal equilibration was largely completed, with the aforementioned water-level fluctuations appearing as intervals of varying shoreline progradation or retreat. The fourth phase began at about 1.2 ka b2k, when the rate of rise increased again with the onset of the Late Sub-Atlantic Transgression (LSAT). Due to the interruption during the Little Ice Age (LIA), which in Central Europe is mostly dated to 0.55–0.15 ka b2k, the mean rate of rise for the entire period was 0.5–0.7 mm a−1 but reached 1.0–1.5 mm a−1 before and after the LIA (Fig. 2). Initially, the phase was characterised by increased abrasion on the cliffs and an increase in shoreline progradation along the coastal barriers. At the same time, extensive mires developed on the back-barrier sand flats and on other low-exposure sections of the coast. After the end of the LIA, erosive tendencies generally intensified. Currently about 70 % of the formerly prograding coastline has a negative sediment balance (Schwarzer, 2003). An accelerated sea-level rise will exacerbate this trend in the future.
Lithofacies differentiation is mainly determined by the position of depositional areas in relation to sea level and shoreline, hydrodynamic exposure, and sediment availability. As a result of the transgression- or regression-related shifts in the facies spaces, characteristic vertical sequences of lithofacial units developed in the course of the coastal evolution which can be defined as standard profiles.
For example, with the inundation of the pre-Littorina relief gravelly or blocky residual sediments formed on elevated till deposits, which were covered by marine-brackish shallow-water sand and later by dunes or cover peat (Fig. 4: Barrier). In contrast, basal organic sediments indicating ponding accumulated in the depressions of the relief shortly before the arrival of the transgressing sea (Kliewe and Janke, 1978). After inundation, they were covered by thin strata of shallow-water sand (which can also be missing and is therefore not shown in Figs. 3 and 4) and lagoonal mud after a sufficient water depth was reached (Fig. 4: Lagoon). Where elongating spits evolved, the mud was overlain by shallow-water and beach sand or pebbles, on which dunes of different generations could develop as the shoreline started to prograde (Fig. 4: Barrier).
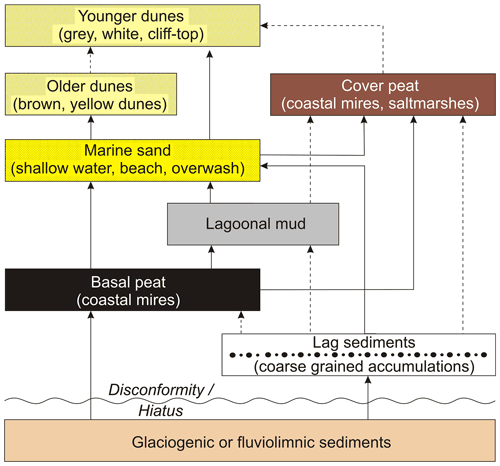
Figure 3Sediment and facies sequences in the coastal Holocene of Mecklenburg-Vorpommern, empirically proven by sediment cores. The sequences do not have to be completely developed but can end at each facies change. Transitions marked with broken lines occur only rarely. Note that the vertical sequence of facies units is not necessarily equivalent to the temporal sequence.
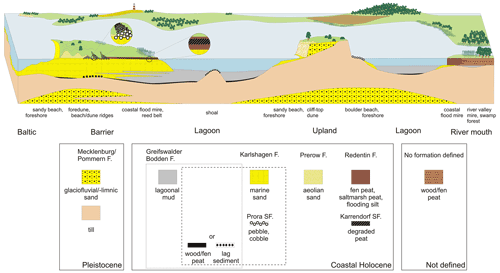
Figure 4Schematised cross section (not to scale) through the SW Baltic coast illustrating the main coastal landforms, their typical vertical sediment sequences (including the formations and subformations as described in this synthesis), and the lateral sediment interlocking, also with sediments, not belonging to the coastal Holocene. Note that not all possible depositional sequences (see Fig. 3) are shown.
The depositional sequences (standard profiles) empirically proven by sediment cores are marked by arrows in Fig. 3. More specifically, however, the depicted sequences do not have to be fully developed but can end before any facies change. They form the framework in which the lithofacial units (formations) are to be defined. Since for practical reasons the number of formations should be kept as low as necessary (Piller et al., 2003), the thin basal peats and the residual sediments are not kept as separate formations but are added to the subsequent facies unit. In addition, the dunes of different generations are combined into one unit. This results in four formations:
-
basal peat or lag sediment and brackish lagoonal mud (Greifswalder Bodden Formation),
-
basal peat or lag sediment and marine-brackish shallow-water and beach sediment (sand, gravel, boulder) (Karlshagen Formation),
-
aeolian sand (Prerow Formation),
-
cover peat and sediments of the coastal mires (Redentin Formation).
4.1 Greifswalder Bodden Formation (GBF)
Lithologically, the GBF is predominantly characterised by lagoonal mud (Schlick). Although Möbus (2000) designated the formation as Mudde-Sequenz, it is recommended to use the term Schlick to emphasise the distinction from Mudde as a limnic sediment (Ad-hoc-Arbeitsgruppe Boden, 2005). Different approaches to the definition of lagoonal mud can be found in Kolp (1966), Lindner (1972), Schlungbaum (1979), Leipe (1986), and Lampe and Meyer (1995). In the revision of the “Definitions of Geogenetic Term of the Geological Mapping Guide” (AG Geologie, 2021), mud is described in general terms, GeolKAID 323, and again under the term lagoonal deposits, GeolKAID 29, in a narrower sense to denote fine-grained basin sediments.
The muddy sediments of the GBF consist mainly of silt (40 %–95 % dry weight) with variable admixtures of fine sand and to a lesser extent also clay, as well as organic matter between 5 % and 30 % dry weight. Within the individual lagoons, the composition is relatively uniform due to intensive wind-driven resuspension. In contrast, there are clear differences when comparing different lagoons, which are mainly due to hydrographic conditions such as water exchange with the Baltic Sea, river input, land use in the catchment area, bioproductivity and resuspension conditions (Lampe and Meyer, 1995). Lagoons having a high water exchange with the Baltic Sea (outer lagoons: Greifswalder Bodden, Großer Jasmunder Bodden, Grabow; Fig. 1) show lower concentrations of organic matter and higher proportions of fine sand.
Below a brownish oxidised surface layer a few millimetres thick (which may also be absent at times of oxygen deficit), the colour of the mud is black or olive-black due to the formation of iron–sulfur compounds. Characteristic is the intensive smell of H2S. The FeSx × nH2O content may reach more than 7.5 % dry weight (Neumann et al., 2005). The formation can be traced into the lower sections of the coastal rivers where it interlocks with peat and gyttja of the river floodplains. The Greifswalder Bodden as the largest water body of the Western Pomeranian lagoons has been best studied sedimentologically and stratigraphically (Kolp, 1976; Leipe, 1986; Verse, 2001) and represents the type area of the formation.
The lower boundary of the formation represents the transgression surface of the Littorina Sea (Verse et al., 1998) during L1T (Fig. 2). Its age depends on the time of flooding, and it varies in its character depending on the exposure, sediment and morphology of the flooded area. As a result of the sea-level rise, at first the development of shallow paludification mires (Versumpfungsmoor) started in the coastal lowlands (this refers to the term Basistorf as defined by Lange and Menke, 1967; detailed descriptions can be found in Kliewe and Janke, 1978; Strahl, 1997; Verse et al., 1999). These basal peats (see Figs. 3 and 4) mark the upper limit of the sea level at the time of their growth, and, if reliably dated, they can be used as sea-level indicators (sea-level index points, slip; see Figs. 2 and S2 in the Supplement). For the indicative meaning of sea-level indicators, methodological limitations and uncertainties the reader is referred to the literature, e.g. Dörfler et al. (2009), Hijma et al. (2015), and Khan et al. (2019).
The transgression contact representing the transition from the terrestrial to the marine environment is formed as a change from this peat to a sandy and peaty mud or as an interbedding of these sediments (Janke and Kliewe, 1990). As an erosive reworking horizon, it is usually less suitable for dating. In accordance with the landward shift of the facies, marine shallow-water sand follows on top (Fig. 7). Due to the fast sea-level rise and the resulting rapid increase in the landward distance of the accumulation area, the sandy deposits generally have a low thickness and may even be missing. In hydrodynamically more exposed areas, an abrupt sediment change can be observed, where superficially eroded glaciogenic or fluviolimnic sediments of the Pleistocene or Early Holocene are followed by the typical lagoonal mud. Such a transition corresponds to an erosive ravinement surface (Verse et al., 1998) and – if till is the underlying sediment – might be accompanied by the formation of coarse-grained lag sediments (Figs. 3, 4 and S3). According to the little available 14C data, the hiatus associated with the change from terrestrial-telmatic to marine-brackish conditions can cover a period of several hundred to more than a thousand years (see also Kliewe and Janke, 1978).
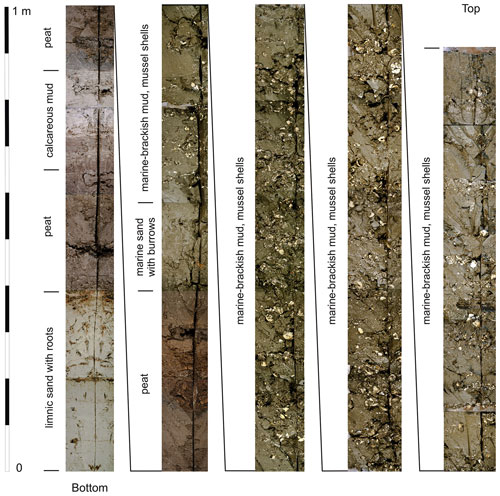
Figure 5Composite photo of the 5.9 m long sediment core GB95-2 from the northern Greifswalder Bodden, water depth 8.2 m; for the position see Fig. 1. Above late glacial/Early Holocene sediments (sand, peat, calcareous mud, peat), the lower boundary of the Greifswalder Bodden Formation is situated within the upper peat at the transition from a terrestrialisation mire (Verlandungsmoor) to a paludification mire (Versumpfungsmoor). Above marine sand in shallow-water facies (which is mostly of low thickness or missing) lagoonal mud (Schlick) with numerous shells (molluscan shell breccia, sensu Kolp, 1976) follows, forming storm surge layers (tempestites) towards the top. Due to the sampling method, the uppermost ca. 0.3 m of the core is missing (photo: Reinhard Lampe, 1995).
In the central areas of the basins, lagoonal mud accumulation began with a thin layer that still contains little amounts of shell hash of marine species. Above this, a several-decimetre-thick section with a high concentration of mollusc shells is generally observed. The detritus of mainly Cerastoderma, Mytilus and Hydrobia shells can reach up to 50 % of the sediment mass (molluscan shell breccia, sensu Kolp, 1976). This shell accumulation marks the phase of maximum inundation, greatest land distance and lowest sediment input and is an expression of a sediment deficit in which relatively little clastic material reached the accumulation area (Figs. 5, S3). The extent to which a trophic favouring of the zoobenthos took place during this period has not yet been investigated. The massive shell hash accumulation in the Greifswalder Bodden began around 7.1 ka b2k (Poz#2-91310) and ended around 5 ka b2k (Poz#2-91309, possible reservoir effect not included in either case). In the Oder Lagoon, Borówka et al. (2005) rank the marine-influenced section between 7.1 ka b2k (Gd-16027) and ca. 5 ka b2k by estimate. Age estimates can also be derived from pollen analyses of cores from other lagoons. They show that in the sediment columns, the pollen zones VII (late Atlantic) and especially VIII (Sub-Boreal, according to Franz Firbas) are characterised by low mass accumulation rates (Schumacher et al., 1998). Temporally, this largely coincides with the PLR (Fig. 2).
A noticeable growth of the spit and barrier system started about 5 kyr ago and increasingly separated the lagoons from the Baltic (Reimann et al., 2011; Lampe and Lampe, 2021). During this phase, which lasted until about 2 ka b2k, mud accumulation in the lagoons increased relative to the deposition of shell hash. Storm-wave-induced resuspension and subsequent re-sedimentation of the uppermost sediment layer led to the separation of mud and hash and thus to the formation of numerous tempestite layers (Verse, 2001; Fig. S4).
For the period 2.1–0.75 ka b2k, another strong increase in the mass accumulation rate is documented for the lagoons. The onset of the LSAT (Fig. 2) and the related shoreline erosion, an increased separation from the Baltic Sea, and advancing deforestation in the catchment area can be assumed as causes, but the relative significance remains unclear (Schumacher et al., 1998). As the dating of the period is based on pollen data, the above timing does not necessarily represent the interval of increased accumulation; it merely gives the limits within which the rate was calculated.
The mollusc fauna reconstructed from the shell hash suggests several salinity changes. Although marine-brackish conditions developed rapidly with the flooding, the salinity maximum appears to be offset by several hundred years due to the backwater of the rivers. Characteristically, large and thick shells of mainly Cerastoderma glaucum, Scrobicularia plana and Mytilus edulis appear then. Littorina littorea – as it prefers to colonise harder ground – is rarely found in the mud probably due to redeposition.
With the ongoing isolation of the lagoons from the sea and the increasing importance of river discharge in the water exchange, the species spectrum adapted to the respective salinity. In the lagoon Kleines Haff, the accumulation of mud containing shells of marine species in appreciable quantities ended at about 4 ka b2k, in the Saaler Bodden at about 2 ka b2k and in the Großer Jasmunder Bodden (for locations see Fig. 1) at about 1 ka b2k (Schumacher et al., 1998; ages are derived from pollen data and can be several hundred years higher than calibrated 14C ages of corresponding samples, uncorrected for a reservoir effect). In contrast, due to the intensive water exchange with the Baltic, the salinity development in the Greifswalder Bodden rather reflects that in the Pomeranian Bay (Fig. 1). So far, two special regional events can be inferred from diatom analyses: the local maximum salinity with 12–14 PSU lasted until about 4 ka b2k, then decreased to 10–12 PSU and dropped to the current level of 7–8 PSU between 1.6 and 1.3 ka b2k (Janke, unpublished). Increased salinity over shorter periods in recent times (e.g. between 1.8 and 0.8 ka b2k in the Kleiner Jasmunder Bodden; Fig. 1, Lampe et al., 2002) is possibly related to temporary modifications in the water exchange with the Baltic Sea. The last occurrence of the species Scrobicularia plana, typical for Littorina-age sediments and indicating salinities ≥8 PSU (Frenzel, 2006) or ≥10 PSU (Jaeckel, 1952), is dated to 1.8 ka b2k in the Greifswalder Bodden (Poz#2-91305, not including a reservoir effect). In the coastal section of the river Ryck, which flows into the Greifswalder Bodden (Fig. 1), S. plana is recorded up to the middle of pollen zone IXa (Janke, unpublished), which corresponds to an age of ca. 2.0–1.8 ka.
The youngest mud strata, about 300–500 years old, are nearly free of mollusc remains, which indicates a considerable deterioration of the living conditions. The occurrence of Mya arenaria in the lagoons with a higher salinity is restricted to sandier sediments in the transition to the Karlshagen Formation. An age of ≤0.5 ka is usually assumed for this species being introduced from North America. Its first occurrence in the Greifswalder Bodden was dated to about 0.7±0.07 ka b2k by Behrends et al. (2005) based on amino acid racemisation. However, probably due to temperature effects, this age may be too high. As another neozoon, Dreissena polymorpha has populated the inner lagoons with the lowest salinity (Saaler Bodden, Kleines Haff) since about 200 years ago (Gruszka, 1999).
Particularly characteristic is the increase in concentration of organic matter, phosphorus and the metals Zn, Pb and Cu in the uppermost decimetres of the sediment column, which, according to Leipe et al. (1998), began with the industrial and agricultural development in the catchment around 1850 CE (cf. Borówka et al., 2005). Although this increase varies in the individual lagoons depending on the river water input, it is detectable in all of them (Fig. 6). In the highly eutrophic waters of the Kleines Haff and the Saaler Bodden (Fig. 6a, b), which belong to the inner lagoons and are both strongly influenced by the rivers Oder and Recknitz, chalk contents of about 20 % and 10 % on average, respectively, are also characteristic and are based on summer calcite precipitation as a result of photosynthesis of the phytoplankton. This anthropogenically modified layer (Hensel et al., 2006) can comprise the uppermost 10 to >100 cm, depending on the sedimentation rate and redistribution intensity; an average of 20 cm is to be expected.
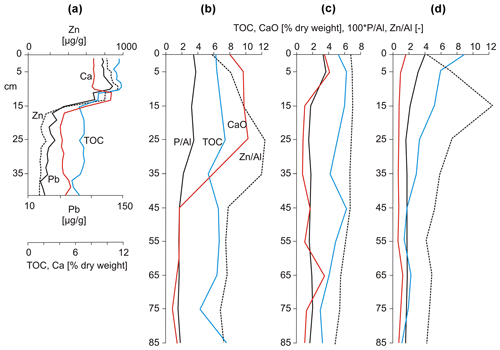
Figure 6Concentrations of selected elements in short sediment cores from the Kleines Haff (a, sample interval 1 cm, based on Leipe et al., 1998), the Saaler Bodden, Bodstedter Bodden and the Grabow (b, c, d; sample interval 10 cm, the P and Zn contents are normalised to the Al concentration; TOC = total organic carbon; based on Hensel et al., 2006). For the positions of the cores see Fig. 1.
The GBF forms the surface of the coastal Holocene in the mud-filled central areas of the lagoons. The mean thickness is probably about 3 m, and the maximum thickness known so far is 9 m (Kleiner Jasmunder Bodden). Only the older part of the formation is found under the coastal barriers. Here, due to the distance of the depocentre from the shoreline and the compaction due to the overburden, the characteristic black, mollusc-rich mud is mostly found below −8 m NHN. In sheltered areas or in the coastal sections of the rivers, the formation is somewhat more complete and may already be present at −1 to −3 m NHN (Janke and Kliewe, 1990). When covered by littoral shallow-water sand, the upper boundary of the GBF is indistinctly marked by an alternating bedding of sand and mud, which represents the transition to the Karlshagen Formation. The age of the upper boundary is diachronic and ranges from the beginning of the progradational coastal development to the present.
It has to be noted that the marine mud of the much deeper Baltic Sea basins differs significantly from the lagoonal mud in terms of grain size distribution, fossil content, concentration of organic matter and trace elements and is not represented by the GBF.
4.2 Karlshagen Formation (KF)
During the phase of rapid sea-level rise, a large part of the material eroded from the uplands reached the seaward edge of the coastal zone comparatively quickly. Thus, it was not available for long-shore transport or only available with a time lag. Under these conditions, neither an equilibrium profile normal to the coast could develop nor could a significant shoreline adjustment parallel to the coast take place. As a result, the moraine landscape with its uplands and depressions developed into an archipelago with deficient sedimentation in the inundated basins (see GBF). With the declining sea-level rise rate in the L2T (Fig. 2) the volume of coastal long-shore transport started to grow. The material eroded from the active cliffs and the still unbalanced foreshore areas was increasingly transported parallel to the coast and accumulated where the shoreline turned landward and the coastal long-shore transport led into deeper water (Hoffmann and Lampe, 2007; Lampe and Lampe, 2021). Wide, sandy shallow-water accumulations with subaerial spits growing into the basins developed (Figs. 7, S5 and S6). At the same time, the filling of the basins intensified due to increased supply of silty material transported beyond the shallow-water areas.
These shallow-water and beach sediments form the Karlshagen Formation (KF; Obere Sandfolge according to Möbus, 2000). They consist predominantly of grey, silty fine and medium sands in varying mixtures. Coarse sands, gravels and pebbles are, with exceptions, bound to (formerly) subaerial beach ridges and berms. In general, the transition from subaqueous to subaerially deposited sand is gradual. Grain coarsening tendencies towards the ground surface (coarsening upward) are regularly observed, as well as from the back-barrier to the front-barrier area (Kliewe, 1960; Hoffmann and Barnasch, 2005; Hoffmann et al., 2005). Channel and overwash formations lead to local variations (Fig. 7). The subaquatic sands contain small amounts of shell hash. Plant remains occur regularly. Occasionally, they are concentrated in platy detritus layers and should not be confused with thin peat strata, which would be recognisable from a rooting horizon below.
The diachronous lower boundary of the formation is marked by the transition to glaciogenic sediments of the Pleistocene or fluviolimnic sediments of the Early Holocene, which often show a fossil soil or a basal peat on top. If the KF is underlain by the GBF, the lower boundary is indistinct due to alternating bedding of sand and mud at the transition. So far, only a few absolute dates are available for the Banneminer Niederung/Usedom (Fig. 1), where the sands of the KF have a maximum OSL age of 6530±470 years (Lampe and Lampe, 2021). Thus, the age of the lower boundary extends from the end of the L2T to the present at sites where spits and related foreshore and sandflat sediments (e.g. Bessin with the Bessinsche Schaar/Hiddensee, Gellen/Hiddensee with Gellenschaar, Zingst with the Werder sandflat; Figs. 1 and 7) accumulate over non-marine late glacial/Early Holocene sediments or muds of the GBF or where strandplains prograde over marine sands of the Baltic Sea (Peenemünder Haken/Usedom, Neudarß; Fig. 1). The distinction from the latter, which are mostly marine redeposited sands of glaciofluvial or glaciolacustrine origin, is problematic because the grain size spectrum and fossil content are very similar.
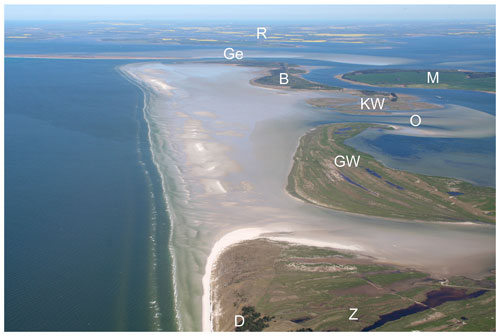
Figure 7Sandflat east of the Zingst peninsula (Z) with the islands Großer Werder (GW), Kleiner Werder (KW) and Bock (B) and in the background the southern tip of Hiddensee with Gellenschaar (Ge) and Rügen (R); M marks the mainland (see Fig. 1 for the position of the image section). The sandflat and the bases of the islands are made up of shallow-water sand up to 4 m thick and the islands of prograding beach ridges. Between them overwash deposits (O) are recognisable. All deposits belong to the Karlshagen Formation. Dunes (D) of the Prerow Formation are deposited on the seaward beach ridges of the Zingst peninsula (photo: Reinhard Lampe, 2007).
On the barriers of the Vorpommern coast, the average thickness of the KF is about 8 m; the maximum thickness observed so far amounts to 15 m. In the drilling data repository of the Geological Survey of Mecklenburg-Vorpommern even higher values are found, also in Kliewe (1960), but the marine origin of the sands described there seems uncertain. Lithofacially, the thin beach deposits in front of the cliffs, which border the barriers and represent their main sediment source, also belong to the KF (Fig. S7). The thickness of these sediments hardly exceeds 1–1.5 m on average.
As a special feature, the Prora Subformation (PSF) is differentiated. It is characterised by beach ridges consisting predominantly of pebbles. At the type location on the barrier Schmale Heide/Rügen, the beach ridges with a thickness of up to 4 m and a height of up to 2.5 m NHN consist of approximately 95 % flint pebbles and cobbles, which probably originated from a glaciofluvial concentration in a neighbouring shoal (Fig. 8). In the north of the barrier, they overlie a peat layer of the Redentin Formation, which yielded a 14C age of 4.4 ka b2k (Hoffmann, 2004), but for morphological reasons a somewhat older age must be assumed for the oldest ridges. Similar beach ridge formations, consisting mainly of flint, exist on Schaabe/Rügen and on the Fährinsel near Hiddensee (Fig. 1).
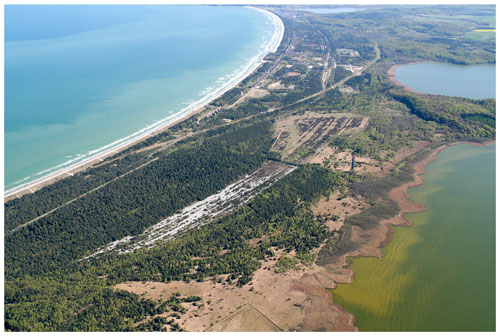
Figure 8Aerial view of the Schmale Heide/Rügen spit from the northwest, with the towns of Prora and Binz in the background (see Fig. 1 for the position of the image section). The marine and beach sands forming the spit, including the sands of the adjoining foreshore areas in the Baltic and the lagoon, belong to the Karlshagen Formation. The light-coloured beach ridges in the image centre consist mainly of flint pebbles (Prora Subformation). The dunes adjoining the Baltic Sea (to the left in the image), covered with forest, belong to the Prerow Formation. On the right two bays of the Kleiner Jasmunder Bodden are recognisable. The peat of their reed belts belongs to the Redentin Formation (photo: Reinhard Lampe, 2007).
For consistency reasons, all pebble and cobble beaches are combined in the PSF and together with the sandy beaches assigned to the KF. Likewise, the coastal sand migration zone, i.e. the foreshore with its sand bars, belongs to the KF (Figs. S8 and S9). The offshore residual sediment zone is to be regarded as the seaward boundary, where the shoreward sand transport separates from the seaward transport of finer material (Pratje, 1948; Kolp, 1966). However, such a residual sediment zone is clearly pronounced only where sediments with a wider grain size spectrum, e.g. till, are eroded and depleted of fine particles. In the case of a purely sandy foreshore, delineation is difficult. The upper boundary of the formation is either equal to the ground surface or coincides with the lower boundary of the dune sands of the Prerow Formation or the lower boundary of the coastal mires of the Redentin Formation.
4.3 Prerow Formation (PF)
The PF comprises exclusively aeolian sediments. Most of the sediments are dunes that rest on underlying beach ridges belonging to the KF (Figs. 3 and 4). They consist mainly of well to very well sorted, non-calcareous fine to medium sands. The transition from the beach ridges to the overlying drift sand is usually gradual, especially if the beach sand is well sorted. Otherwise, the absence of coarse sand, gravel and mollusc detritus is a useful criterion to distinguish them from the beach sediment.
Usually, all sandy beach ridges, which are no longer reshaped by annual storm floods, have a drift sand cover and are raised to a greater or lesser extent. Dunes form from them where sand is delivered from the beach for a sufficiently long time and is subsequently fixed by vegetation. With a prograding shoreline due to a positive sand balance or a falling sea level, a new beach ridge forms in front of the dune within a few decades. This increasingly focuses the sand input on a new foredune, whereby the older dune successively becomes inactive and finally covered by forest.
Keilhack (1912) gave a comprehensive description and first subdivision of the beach ridges and the coastal dunes on top of them, using the Świna Gate between the islands of Usedom and Wolin as an example (see also Reimann et al., 2011). According to the occurrence of typical soil horizons and their colour, he distinguished between brown dunes with iron–humus podzols (Bhs/Bs horizon; Fig. 9), yellow dunes with humus podzols (Bh/Bsh horizons) and white dunes (syrozems with O/Ai/Ah horizons) and concluded that their age decreased in this order. Janke (1971) separated the grey dunes with a distinct Ah horizon (regosols; see Ad-hoc-Arbeitsgruppe Boden, 2005) from the white dunes and placed them between the yellow and white dunes in terms of age.
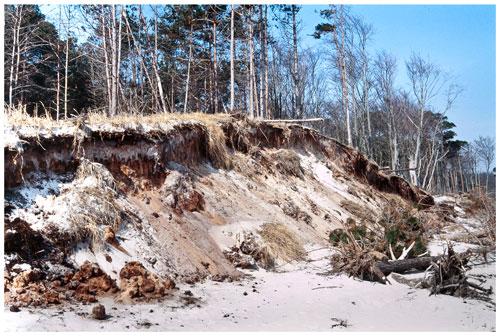
Figure 9Brown dune of the Prerow Formation with pronounced iron–humus podsol in Neudarß (for the position see Fig. 1; photo: Reinhard Lampe, 1995).
First attempts to date the dune generations are based on 14C data from swale peats (Prusinkiewicz and Noryskiewicz, 1966) between the dunes of the Świna Gate (see Fig. 1). The results, however, are to be regarded as minimum ages. Reliable absolute chronological data can only be obtained with the OSL method. So far, the oldest ages (6.6±0.4 ka b2k) within the KF have been found at the dunes of the Świna Gate (Reimann et al., 2011) and fall into the L2T phase with clearly decreasing water-level rise. Thereafter, the formation of dunes remains closely linked to the sediment budget of the coastal section. Rapid progradation of the shoreline is coupled with the formation of relatively numerous beach ridges with low dunes on top, while slow or no progradation results in fewer beach ridges covered by higher dunes (Lampe and Lampe, 2018). The grey dunes formed during the LIA. In contrast to the uniform, linear walls of the other dune generations, they have an irregular, hummocky and elevated relief with heights of up to 15 m. Fossil soils, lidar surveys and OSL dating show that grey dunes often dispersed, developing individual barchanoid forms and transgressed over older, lower linear dunes (Lampe and Lampe, 2021). Janke (1971) attributes the development of this dune type, which can be found on all SW Baltic strandplains, to the destruction of the vegetation cover by humans and to the special climatic conditions of the LIA. Today, they are mostly stabilised by forests, but in some cases they are still active (Fig. S10).
Cliff-top dunes also belong to the aeolian landforms of the coastal area. They form where cliffs predominantly consist of non-cohesive, sandy sediments, which are blown out by landward-directed winds and deposited behind the upper cliff edge (Fig. S11). Like the cliff itself, they erode and shift landward with the retreat of the cliff's edge. Thus, cliff-top dunes are subject to a constant recycling process and are always relatively young accumulations. Although the mechanism of development is different from that of strandplain dunes, the lithological differences, such as the smaller grain size and a chalk content of 2 %–3 % (Kliewe, 1973), are not striking. It seems therefore appropriate to include the cliff-top dunes in the PF. If necessary, their special position can be taken into account by defining a subformation.
The lower boundary of the PF at the transition to the underlying KF can be very gradual and indistinct but can be estimated by its position relative to the sea level. For the cliff-top dunes, the lower boundary is clearly marked by the soil of the sub-ground over which the dune migrated. The average thickness of the KF is difficult to determine due to the unstable relief but is probably around 2 m; the maximum thickness is around 15 m in the transgressive grey dunes of the LIA. In the case of the cliff-top dunes, a maximum thickness of 25 m (which can no longer be verified) was observed at Streckelsberg/Usedom (Wernicke, 1930); here, too, the mean thickness is probably in the range of a few metres. The upper limit of the formation is always equal to the ground surface.
4.4 Redentin Formation (RF)
In sheltered areas of the inner lagoons and in the lower reaches of the coastal rivers, siliciclastic sedimentation was not yet possible during the phases L1T and L2T of the sea-level rise. With the onset of inundation, lagoonal mud accumulated under marine influence (GBF), while gyttja predominantly deposited in fluvially affected sections. In places, the backwater into the river valleys temporarily led to the development of lake-like waters in the lower reaches, in which calcareous fine detritus gyttja accumulated, e.g. in the Recknitz valley (Succow, 2001). After the end of the rapid rise, Phragmites peat accumulation prevailed in the river valley mires (Flusstalmoore), in which the decreasing influence of seawater is accompanied by a decreasing sulfur content (Kliewe and Janke, 1978; Janke, 1978; Succow, 2001).
At the transition of the valleys into the lagoons, the river valley mires interlock with the coastal mires, which are usually only shallow and continuously influenced by brackish water. In small sheltered depressions their accumulation started at about 7 ka b2k, while the largest increase in area is linked to the LSAT, which began at about 1.2 ka b2k (Fig. 2). This new transgression led to a widespread rise of the groundwater table, to ponding in the lowlands surrounding the lagoons and to the development of coastal terrestrialisation mires (Küstenverlandungsmoore; Slobodda, 1989; Fig. S12). Unlike in inland reed beds, however, the growth zone of the Phragmites reed along the lagoon shores is confined in the vertical to a small interval of ±0.2 m around mean sea level (Krisch, 1978; Slobodda, 1989). Because of this close relationship, only a rising sea level can lead to an aggradation in the coastal mires dominated by Phragmites, while even a slight lowering may lead to desiccation, mineralisation and associated peat shrinkage. This results in a highly decomposed peat (Fen or Mulm according to the German classification), which is characterised by the absence of macroscopically recognisable plant remains and by a conspicuously dark colour (Schwarze Schicht; Fig. S13). Where thicker peat strata accumulated in sheltered depressions, two and in one case even three black layers within the uppermost 1.5 m have been detected (Lampe and Janke, 2004). Palynostratigraphically and chronostratigraphically, these layers were assigned to cooler climatic phases, which apparently were associated with sea-level recession or retardation (see Fig. 2). The conspicuous and typically formed uppermost black layer is designated as the Karrendorf Subformation (KSF; Fig. 10). The phenomenon probably corresponds to the Humus-Dwog horizon in the marsh soils of the North Sea coast (Behre, 1986; Scheder et al., 2018), but comparative studies are still lacking.
In some areas, a further type of peatland occurs in the form of coastal flood mires (Küstenüberflutungsmoore; Fig. S14). Phenologically it differs from the coastal terrestrialisation mires, which are characterised by reed beds, by the dominance of grassland communities, and morphologically by a higher position above the mean water level and sedimentologically by the occurrence of flooding silt (Überflutungsschlick), which is characterised by a higher content of mineral matter. Typically, the content of organic matter varies between 20 % and 30 % (Antorf), but locally up to 80 % has been observed. Phragmites largely recedes as peat-forming vegetation; radicells of rushes (above all Juncus gerardii) and grasses are predominantly observed as the main peat former, but sedges occur too. Jeschke and Lange (1992) described this sediment, which is characteristic of coastal mires used as pastures or meadows, as saltmarsh peat; on the geological map GK25 it is designated as flooding silt. The flooding silt layer reaches thicknesses of 2 to 4 dm and typically occurs above the KSF.
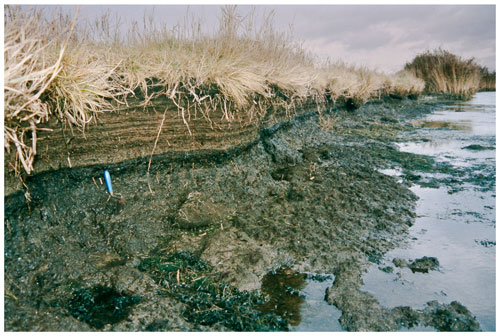
Figure 10Between underlying Phragmites peat and overlying saltmarsh peat, which together form the Redentin Formation, the Karrendorf Subformation is recognisable as a black layer of heavily degraded peat. Shore of the Karrendorfer Wiesen near Greifswald during low water (for the position see Fig. 1; photo: Reinhard Lampe, 2003).
According to Jeschke and Lange (1992), the development of the sequence black layer with covering saltmarsh peat (flooding silt) is the result of the coincidence of a sea-level fluctuation and a land-use change. Hence, the regression at the transition from the Medieval Climate Anomaly (MCA) to the LIA led to the development of the black layer as a result of the desiccation and oxidation of the peat that had developed during the previous phase of rising sea level. Subsequently, the use of the desiccated reed belt areas as mowing meadows and cattle pastures, which began in the 13th century, promoted the establishment of saltmarsh vegetation and prevented a potentially renewed growth of Phragmites reeds during the subsequent phase of sea-level rise. The compaction of the substrate by cattle trampling and the production of H2S promoted by the saltwater influence ensured that the grass roots were inhibited from decomposing. In combination with the more frequent flooding during the LIA and the resulting increase in clastic sediment input, this led to the saltmarsh growing up to 60 cm above the mean water level. The saltmarsh peat (flooding silt) accumulation should therefore be regarded as an anthropo-zoogenic formation.
Lampe and Janke (2004) have shown that flooding silt already occurred in earlier phases of coastal mire evolution. In such cases, too, it was the successor sediment of a black layer (see Fig. 2) but, like the latter, not as typically formed as the deposits built in the MCA and in the LIA. Mostly, it is merely silty peat. Information about the mean content of mineral matter is not meaningful for detection, as the values vary greatly depending on the (palaeo-)exposure. However, the deviation becomes clear in comparison to the under- and overlain strata, and this may be used as an indicator.
In areas not affected by dyking, drainage or land use change the flooding silt accumulated in the LIA is followed by a shallow saltmarsh peat as the uppermost layer, which has a much higher organic content than the saltmarsh peat below (Fig. S15). This transition to an increased accumulation of organic matter is probably caused both by a reduced sediment input from the lagoons and a decreased removal of above-ground biomass of the mire through mowing and grazing. The extent to which this also reflects an accelerated sea-level rise is currently still open.
The RF includes two types of peatlands: coastal terrestrialisation mires and coastal flood mires. Lithologically, they consist of moderately to highly decomposed reed, sedge and saltmarsh peats with a variable content of mineral matter (Antorf, Halbtorf or Volltorf), as well as moderately to strongly degraded peat (Fen or Mulm) and flooding silt (Überflutungsschlick), the latter both being restricted to the upper section of the depositional sequence. Towards the shore, increasingly silty and fine sandy admixtures occur in the peat. Especially in the immediate shoreline area, wide, shallow sandy embankments can sit on top of the mires and can be interpreted as beach ridges.
The coastal mires rarely reach a thickness of more than 1.5 m, in many areas considerably less. Due to drainage measures and the associated subsidence and oxidation, the mires are severely degraded, especially in the back-barrier areas. Only in the lower reaches of rivers and creeks have thicknesses of up to 4 m been observed locally (Niederes Fischland; Lampe et al., 2010; Redentin/Groß Strömkendorf; Lampe et al., 2005; coastal sections of the rivers Recknitz; Peene and Uecker, Janke, 1978; Endtmann, 2006; Michaelis and Joosten, 2009). In the back-barrier areas, the formation overlies the KF, which defines the lower boundary of the RF. On the mainland side of the lagoons, the RF is underlain by Pleistocene sands and till, mostly of the Mecklenburg Formation or Pommern Formation. The upper boundary is usually defined by the ground surface, but in rare cases older parts of the RF may be overlain by sediments of the KF and PF. In sheltered bays and river valleys, the RF interlocks with river valley mires (Fig. 4), which are difficult to differentiate. Apart from vegetation remains or foraminifera indicating the influence of brackish water, a higher sulfur content is a possible indicator for the coastal Holocene.
The four lithostratigraphic formations and two subformations described above represent all Holocene littoral deposits accumulated in the near-coastal SW Baltic realm since the region was reached by the Littorina transgression. The Greifswalder Bodden Formation started at first to accumulate, is dominated by fine-grained mud and includes underlying thin transgression contact deposits like basal peat or lag sediment. It mainly represents calm-water conditions, but tempestite layers mark occasional erosion and redeposition during storm floods.
The Karlshagen Formation started to accumulate not earlier than about 7 kyr ago when the rate of the sea-level rise declined. Sandy shallow-water accumulations with subaerial spits evolved and developed into prograding beach-ridge plains. The Prora Subformation as part of the Karlshagen Formation comprises all accumulations that are particularly coarse (pebble to boulder). All sediments reflect the effects of waves and currents.
Many beach ridges of the Karlshagen Formation and the Prora Subformation are covered by wind-driven sand. The Prerow Formation comprises exclusively these aeolian deposits, including the cliff-top dunes. The formation started to develop somewhat later than the Karlshagen Formation, as it needs dry, subaerial sand accumulations to form dunes.
The Redentin Formation is dominated by organic deposits as Phragmites peat or saltmarsh peat. In a few sheltered areas it started to accumulate somewhat after the Greifswalder Bodden Formation, which it may overlie. The formation has the widest distribution on the low-lying coastal belt of the mainland and on the back-barrier areas of the coastal barriers. Here, the accumulation started mainly about 1.2 kyr ago with the onset of the Late Sub-Atlantic Transgression. The Karrendorf Subformation is a layer of degraded peat, which developed shortly before the Little Ice Age due to a falling sea level. Except for the Karrrendorf Subformation, all formations are still in development.
The lithostratigraphic units are defined in a way that they are easy to recognise in the field from sediment cores and without any methods, which are not usually available in field surveys. However, it was not intended to test the suitability of the defined formations for mapping. Mapping is the task of the competent State Geological Survey, and thus, the usefulness and applicability can only be assessed within the survey's mapping practice. With the mapping experience gained, it might become necessary to revise and adjust the subdivision of the units and their definitions.
The formations as described above are well backed by the results of many investigations of the coastal barriers, the lagoons and the near-coastal offshore area of Mecklenburg-Vorpommern. Since the environmental conditions and the related facial characteristics increasingly diverge because of the growing extent of an accumulation area, the validity of a formation's definition is spatially limited. For neighbouring regions, the definitions can not be adopted without reservation and must be verified in each case. For instance, different remains of organisms or ages of accumulation must be expected even over shorter distances in the SW Baltic region, as the environmental conditions vary greatly. Also, lithofacially deviating characteristics are anticipated for lagoonal sediments and coastal mires elsewhere, which may require a more comprehensive characterisation of the existing formations in the future. Practicable solutions also need to be found to separate the set of coastal formations from inland formations, especially in areas of valleys and lowlands, and from marine formations of the open sea.
The drilling data repository of the State Geological Survey of Mecklenburg-Vorpommern can be accessed at https://www.umweltkarten.mv-regierung.de/atlas/script/index.php (last access: 22 November 2022; LUNG MV, 2022). All other data are available from the author upon reasonable request.
The supplement related to this article is available online at: https://doi.org/10.5194/egqsj-71-249-2022-supplement.
The author has declared that there are no competing interests.
Publisher’s note: Copernicus Publications remains neutral with regard to jurisdictional claims in published maps and institutional affiliations.
This article is mainly based on data collected since 1992 at the Chair of Physical Geography, University of Greifswald. The author would like to express his sincere thanks to all who have contributed. Therefore, the students and PhD students who have not been cited should be mentioned here: Susanne Angelstein, Anke Barthel, Johann Bastrop, Käthe Effenberger, Ulrike Kerstan, Steffen Koch, Elke Mandelkow, Mathias Möller, Anne Müller, Andreas Musolff, Michael Naumann, Susanne Rohleder, Annette Roßdeutscher, Nico Schmedemann, Christian Svensson, Henning Tiarks, Peter Voss and Björn Wohlrab. Particularly valuable contributions were made by my former colleagues Hinrich Meyer and Wolfgang Janke. Thanks also to Matthias Lampe for his support during the completion of the manuscript.
The main part of the data summarised in this paper was collected within projects mentioned below, the funding of which is thankfully acknowledged.
The author gratefully acknowledges critical comments and useful suggestions from the managing editor, Michael Zech; from the Copernicus editorial team; from the anonymous reviewer; and especially from Friederike Bungenstock as the second reviewer. They all helped to improve the paper.
This research has been supported by the EU Commission (project no. PL-910398), the Federal Ministry for Research and Technology (BMBF; grant no. 01LK9405/4), State Mecklenburg-Vorpommern, the German Federal Environmental Foundation (DBU; project no. 20000/134), Federal State Mecklenburg-Vorpommern, and Deutsche Forschungsgemeinschaft (DFG; project no. FOR 488).
The article processing charges for this open-access publication were covered by the University of Greifswald.
This paper was edited by Michael Zech and reviewed by Friederike Bungenstock and one anonymous referee.
Ad-hoc-Arbeitsgruppe Boden: Bodenkundliche Kartieranleitung KA5, Schweizerbart, Stuttgart, 438 pp., ISBN 978-3-510-95920-4, 2005.
Ad-hoc-Arbeitsgruppe Geologie: Geologische Kartieranleitung. Allgemeine Grundlagen, Geologisches Jahrbuch, G9, 135 pp., 2002.
AG Geologie: https://www.geokartieranleitung.de/Fachliche- Grundlagen/Genese/Petrogenetische-Gesteinsbezeichnung/ Lockergesteine/entry/ last access: 6 April 2021.
Ahrendt, K.: Ein Beitrag zur holozänen Entwicklung Nordfrieslands, Die Küste, 71, 1–32, https://hdl.handle.net/20.500.11970/101554 (last access: 14 May 2022), 2006.
Andrén, E., Andrén, T., and Sohlenius, G.: The Holocene history of the southwestern Baltic Sea as reflected in a sediment core from Bornholm Basin, Boreas, 29, 233–250, 2000.
Barckhausen, J., Preuss, H., and Streif, H.: Ein lithologisches Ordnungsprinzip für das Küstenholozän und seine Darstellung in Form von Profiltypen, Geologisches Jahrbuch, A44, 45–74, 1977.
Behre, K.-E.: Meeresspiegelverhalten und Besiedlung während der Zeit um Christi Geburt in den Nordseemarschen, Offa, 43, 45–53, 1986.
Behrends, B., Hertweck, G., Liebezeit, G., and Goodfriend, G.: Earliest Holocene occurrence of the soft-shell clam, Mya arenaria, in the Greifswalder Bodden, Southern Baltic, Mar. Geol., 216, 79–82, 2005.
Berglund, B. E., Sandgren, P., Barnekow, L., Hannon, G., Jiang, H., Skog, G. and Yu, S.-Y.: Early Holocene history of the Baltic Sea, as reflected in coastal sediments in Blekinge, southeastern Sweden, Quatern. Int., 130, 111–139, https://doi.org/10.1016/j.quaint.2004.04.036, 2005.
Borówka, R. K. Osadczuk, A., Witkowski, A., Wawrzyniak-Wydrowska, B., and Duda, T.: Late Glacial and Holocene depositional history in the eastern part of the Szczecin Lagoon (Great Lagoon) basin–NW Poland, Quatern. Int., 130, 87–96, https://doi.org/10.1016/j.quaint.2004.04.034, 2005.
Brand, G., Hageman, B. P., Jelgersma, S., and Sindowski, K.-H.: Die lithostratigraphische Unterteilung des marinen Holozäns an der Nordseeküste, Geologisches Jahrbuch, 82, 365–384, 1965.
Bundesamt für Seeschifffahrt und Hydrographie (BSH): Meeresbodensedimente in der deutschen Ostsee, 1:100 000, BSH Nr. 2931–2939, 2012.
Bundesamt für Seeschifffahrt und Hydrographie (BSH): Anleitung zur Kartierung des Meeresbodens mittels hochauflösender Sonare in den deutschen Meeresgebieten, BSH Nr. 7201, 147 pp., 2016.
Cepek, A. G.: Die Lithofazieskarten Quartär 1:50 000 (LKQ 50)–eine Erläuterung des Kartenkonzepts mit Hinweisen für den Gebrauch, Brandenburgische Geowissenschaftliche Beiträge, 6, 3–38, 1999.
Dietrich, R. and Liebsch, G.: Zur Variabilität des Meeresspiegels an der Küste von Mecklenburg-Vorpommern, Zeitschrift für Geologische Wissenschaften, 28, 615–624, 2000.
Dörfler, W., Jakobsen, O., and Klooß, S.: Indikatoren des nacheiszeitlichen Meeresspiegelanstiegs der Ostsee. Eine methodische Diskussion am Beispiel der Ostseeförde Schlei, Schleswig-Holstein, Universitätsforschungen zur prähistorischen Archäologie, 165, 177–186, 2009.
Endtmann, E.: Häufige botanische Makroreste in Ablagerungen des südlichen Ostseeraumes und das Küstenmoor Körkwitz nahe Ribnitz-Damgarten (Saaler Bodden), Meyniana, 58, 57–74, 2006.
Frenzel, P.: Organismenreste aus holozänen Sedimenten der Ostsee als Paläomilieuindikatoren, Meyniana, 58, 97–128, 2006.
German Stratigraphic Commission (DSK) and the Federal Institute for Geosciences and Natural Resources (BGR): Litholex – “Lithostratigraphisches Lexikon Deutschland”, BGR, https://www.bgr.bund.de/DE/Themen/GG-Stratigraphie/LithoLex/litholex_node.html last access: 6 April 2021.
Gruszka, P.: The River Odra Estuary as a Gateway for Alien Species Immigration to the Baltic Sea Basin, Acta Hydroch. Hydrob., 27, 374–382, 1999.
Helama, S., Jones, P. D., and Briffa, K. R.: Dark ages cold period: A literature review and directions for future research, The Holocene, 27, 1600–1606, https://doi.org/10.1177/0959683617693898, 2017.
Hensel, R., Meyer, H., and Lampe, R.: Untersuchungen an Schlickkernen in der Darss-Zingster Boddenkette, Final project report, Umweltplan GmbH, Stralsund, 56 pp., 2006.
Hijma, M. P., Engelhart, S. E., Törnqvist, T. E., Horton, B. P., Hu, P., and Hill, D. F.: A protocol for a geological sea-level database, in: Handbook of Sea-Level Research, edited by: Shennan, I., Long, A. J., and Horton, B. P., Wiley, 536–553, 2015.
Hoffmann, G.: Rekonstruktion und Modellierung der Küstenevolution im Bereich der Pommerschen Bucht in Abhängigkeit von holozänen Meeresspiegelschwankungen, PhD thesis, University of Greifswald, Germany, 129 pp., 2004.
Hoffmann, G. and Barnasch J.: Late Glacial to Holocene coastal changes of SE Rügen Island (Baltic Sea, NE Germany), Aquat. Sci., 67, 132–141, https://doi.org/10.1007/s00027-005-0763-8, 2005.
Hoffmann, G. and Lampe, R.: Sediment budget calculation to estimate Holocene coastal changes on the southwest Baltic Sea (Germany), Mar. Geol., 243, 143–156, https://doi.org/10.1016/j.margeo.2007.04.014, 2007.
Hoffmann, G., Lampe, R., and Barnasch, J.: Postglacial evolution of coastal barriers along the West Pomeranian coast, NE Germany, Quatern. Int., 133/134, 47–59, https://doi.org/10.1016/j.quaint.2004.10.014, 2005.
Hoffmann, G., Schmedemann, N., and Schafmeister, M.-T.: Relative sea-level curve for SE Rügen and Usedom Island (SW Baltic Sea coast, Germany) using decompacted profiles, Zeitschrift der deutschen Gesellschaft für Geowissenschaften, 160, 69–78, 2009.
Hurtig, T.: Physische Geographie von Mecklenburg, Deutscher Verlag der Wissenschaften, Berlin, 252 pp., 1957.
Jaeckel, S.: Zur Ökologie der Molluskenfauna in der westlichen Ostsee, Schriften des Naturwissenschaftlichen Vereins für Schleswig-Holstein, 26, 18–50, 1952.
Janke, W.: Beitrag zur Entstehung der Dünen der Lubminer Heide sowie der Peenemünde-Zinnowitzer Seesandebene, Wissenschaftliche Zeitschrift der Ernst-Moritz-Arndt-Universität Greifswald, mathematisch-naturwissenschaftliche Reihe, 1/2, 39–54, 1971.
Janke, W.: Untersuchungen zu Aufbau, Genese und Stratigraphie küstennaher Talungen und Niederungen Nordost-Mecklenburgs als Beitrag zu ihrer geoökologischen und landeskulturellen Charakteristik, Habilitation treatise, University of Greifswald, Germany, 172 pp., 1978.
Janke, W. and Kliewe, H.: Genese und Sedimentabfolge einiger ausgewählter Bodden, Wissenschaftliche Zeitschrift der Ernst-Moritz-Arndt-Universität Greifswald, mathematisch-naturwissenschaftliche Reihe, 39, 3, 7–11, 1990.
Jeschke, L. and Lange, E.: Zur Genese der Küstenüberflutungsmoore im Bereich der vorpommerschen Boddenküste, in: Jungquartäre Landschaftsräume. Aktuelle Forschungen zwischen Atlantik und Tienschan, edited by: Billwitz, K., Jäger, K.-D., and Janke, W., Springer, Berlin, Heidelberg, Germany, 208–215, 1992.
Khan, N. S., Horton, B. P., Engelhardt, S., Rovere, A., Vacchi, M., Ashe, E. L., Törnquist, T. E., Dutton, A., Hijma, M. P., Shennan, I., and HOLSEA working group: Inception of a global atlas of sea levels since the Last Glacial Maximum, Quaternary Sci. Rev., 220, 359–371, https://doi.org/10.1016/j.quascirev.2019.07.016, 2019.
Keilhack, K.: Die Verlandung der Swinepforte, Jahrbuch der Preußischen Geologischen Landesanstalt, 32, part II/2, 209–244, 1912.
Kliewe, H.: Die Insel Usedom in ihrer spät- und nacheiszeitlichen Formenentwicklung, Deutscher Verlag der Wissenschaften, Berlin, 277 pp. 1960.
Kliewe, H.: Zur Genese der Dünen im Küstenraum der DDR, Petermanns Geographische Mitteilungen, 117, 161–168, 1973.
Kliewe, H. and Janke, W.: Zur Stratigraphie und Entwicklung des nordöstlichen Küstenraumes der DDR; Petermanns Geographische Mitteilungen, 122, 2, 81–91, 1978.
Kliewe, H. and Janke, W.: Der holozäne Wasserspiegelanstieg der Ostsee im nordöstlichen Küstengebiet der DDR, Petermanns Geographische Mitteilungen, 126, 65–74, 1982.
Kolp, O.: Die Sedimente der westlichen und südlichen Ostsee und ihre Darstellung, Beiträge zur Meereskunde, 17/18, 9–60, 1966.
Kolp, O.: Die submarinen Terrassen der südlichen Ost- und Nordsee und ihre Beziehungen zum eustatischen Meeresanstieg, Beiträge zur Meereskunde, 35, 5–48, 1976.
Kraft, J. C.: Coastal stratigraphic sequences, in: Coastal sedimentary environments, edited by: Davis Jr., R. A., Springer, New York, 361–384, ISBN 0-387-90300-3, 1978.
Krisch, H.: Die Abhängigkeit der Phragmites-Röhrichte am Greifswalder Bodden von edaphischen Faktoren und der Exponiertheit des Standortes, Archiv für Naturschutz und Landschaftsforschung, 18, 121–144, 1978.
Lampe, M. and Lampe, R.: Evolution of a large Baltic beach ridge plain (Neudarss, NE Germany): A continuous record of sea-level and wind-field variation since the Homeric Minimum, Earth Surf. Proc. Land., 43, 3042–3056, https://doi.org/10.1002/esp.4468, 2018.
Lampe, R. and Janke, W.: The Holocene sea-level rise in the southern Baltic as reflected in coastal peat sequences, Polish Geological Institute Special Papers, 11, 19–30, 2004.
Lampe, R. and Lampe M.: The role of sea-level changes in the evolution of coastal barriers – An example from the southwestern Baltic Sea, The Holocene, 31, 515–528, https://doi.org/10.1177/0959683620981703, 2021.
Lampe, R. and Meyer, H.: Sedimentqualität und hydrographische Bedingungen – ein Vergleich verschiedener Boddengewässer, BODDEN, 2, 7–26, 1995.
Lampe, R., Janke, W., Ziekur, R., Schuricht, R., Meyer, H., and Hoffmann, G.: The Late glacial/Holocene evolution of a barrier spit and related lagoonary waters – Schmale Heide, Kleiner Jasmunder Bodden and Schmachter See, Greifswalder Geographische Arbeiten, 27, 75–88, 2002.
Lampe, R., Endtmann, E., Janke, W., Meyer, H., Lübke, H., Harff, J., and Lemke, W.: A new relative sea-level curve for the Wismar Bay, N-German Baltic coast, Meyniana, 57, 5–35, 2005.
Lampe, R., Endtmann, E., Janke, W., and Meyer, H.: Relative sea-level development and isostasy along the NE German Baltic Sea coast during the past 9 ka, E&G Quaternary Sci. J., 59, 3–20, https://doi.org/10.3285/eg.59.1-2.01, 2010.
Lange, W. and Menke, B.: Beiträge zur frühpostglazialen erd- und vegetationsgeschichtlichen Entwicklung im Eidergebiet, insbesondere zur Flussgeschichte und zur Genese des sogenannten Basistorfes, Meyniana, 17, 29–44, 1967.
Leipe, T.: Beiträge zur Geochemie und Ökologie rezenter Sedimente der Boddengewässer im Nordosten der DDR, PhD thesis, University of Greifswald, Germany, 85 pp., 1986.
Leipe, T., Eidam, J., Lampe, R., Meyer, H., Neumann, T, Osadczuk, A., Janke, W., Puff, T., Blanz, T., Gingele, F. X., Dannenberger, D., and Witt, G.: Das Oderhaff–Beiträge zur Rekonstruktion der holozänen geologischen Entwicklung und anthropogenen Beeinflussung des Oder-Ästuars, Meereswissenschaftliche Berichte, 28, 61 pp., ISSN 0939-396X, 1998.
Lemke, W.: Sedimentation und paläogeographische Entwicklung im westlichen Ostseeraum (Mecklenburger Bucht bis Arkonabecken) vom Ende der Weichselvereisung bis zur Litorinatransgression, Habilitation treatise, University of Greifswald, Germany, 186 pp., 1998.
Lindner, A.: Soziologisch-ökologische Untersuchungen an der submersen Vegetation in der Boddenkette südlich des Darßes und des Zingst. PhD thesis, University of Rostock, Germany, 137 pp., 1972.
LUNG MV (Landesamt für Umwelt, Naturschutz und Geologie M-V: Historische Karten: WebAtlasDE (Graustufen), 1:1367390, LUNG MV, https://www.umweltkarten.mv-regierung.de/atlas/script/index.php, last access: 22 November 2022.
Martin-Puertas, C., Matthes, K., Brauer, A., Muscheler, R., Hansen, F., Petrick, C., Aldahan, A., Possnert, G., and van Geel, B.: Regional atmospheric circulation shifts induced by a grand solar minimum, Nat. Geosci., 5, 397–401, https://doi.org/10.1038/NGEO1460, 2012.
Mayewski, P. A., Rohling, E. E., Stager, J. C., Karlén, W., Maasch, K. A., Meeker, L. D., Meyerson, E. A., Gasse, F., van Kreveld, S., Holmgren, K., Lee-Thorp, J., Rosqvist, G., Rack, F., Staubwasser, M., Schneider, R. R., and Steig, E. J.: Holocene climate variability, Quaternary Res., 62, 243–255, https://doi.org/10.1016/j.yqres.2004.07.001, 2004.
Michaelis, D. and Joosten, H.: Mire development, relative sea-level change, and tectonic movement along the Northeast-German Baltic Sea coast, Bericht der Römisch-Germanischen Kommission 2007, 101–134, 2009.
Ministerium für Landwirtschaft, Umwelt und Verbraucherschutz Mecklenburg-Vorpommern (Ed.): Regelwerk Küstenschutz Mecklenburg-Vorpommern, Übersichtsheft, Schwerin, 102 pp., https://www.stalu-mv.de/mm/Themen/Küstenschutz/Regelwerk-Küstenschutz-Mecklenburg-Vorpommern/ (last access: 22 November 2022), 2009.
Möbus, G.: Geologie der Insel Hiddensee (südliche Ostsee) in Vergangenheit und Gegenwart – eine Monographie, Greifswalder Geowissenschaftliche Beiträge, 8, 150 pp., 2000.
Neumann, T., Rausch, N., Leipe, T., Dellwig, O., Berner, Z., and Böttcher, M.: Intense pyrite formation under low-sulfate conditions in the Achterwasser lagoon, SW Baltic Sea, Geochim. Cosmochim. Ac., 69, 3619–3630, 2005.
Niedermeyer, R.-O., Lampe, R., Janke, W., Schwarzer, K., Duphorn, K., Kliewe, H., and Werner, F.: Die deutsche Ostseeküste, Sammlung geologischer Führer, Vol. 105, 2nd edn., Gebr. Bornträger, Stuttgart, 370 pp., 2011.
Piller, W. E., van Husen, D., and Schnabel, W.: Zur lithostratigraphischen Handhabung quartärer Sedimente und deren Darstellung auf geologischen Karten, in: Stratigraphia Austriaca, edited by: Piller, W. E., Österreichische Akademie der Wissenschaften, Schriftenreihe der Erdwissenschaftlichen Kommission, Wien, 16, 7–10, 2003.
Pratje, O.: Die Bodenbedeckung der südlichen und mittleren Ostsee und ihre Bedeutung für die Ausdeutung fossiler Sedimente, Deutsche Hydrographische Zeitschrift, 1, 45–61, 1948.
Prusinkiewicz, K. and Noryśkiewicz, B.: Zagadnienie wieku bielic na wydmach brunatnych Mierzei Świny w świetle analizy palynologicznej i datowania radioweglem 14C, Zeszyty Naukowe Uniwersytetu Mikołaja Kopernika w Toruniu, Geografia, 5, 75–88, 1966.
Reimann, T., Tsukamoto, S., Harff, J., Osadczuk, K., and Frechen, M.: Reconstruction of Holocene coastal foredune progradation using luminescence dating – An example from the Świna barrier (southern Baltic Sea, NW Poland), Geomorphology, 132, 1–16, https://doi.org/10.1016/j.geomorph.2011.04.017, 2011.
Remane, J., Adatte, T., Berger, J.-P., Burkhalter, R., Dall`Agnolo, S., Decrouez, D., Fischer, H., Funk, H., Furrer, H., Graf, H.-R., Gouffon, Y., Heckendorn, W., and Winkler, W.: Richtlinien zur stratigraphischen Nomenklatur, Eclogae Geol. Helv., 98, 385–405, https://doi.org/10.1007/s00015-005-1167-4, 2005.
Rosentau, A., Klemann, V., Bennike, O., Steffen, H., Wehr, J., Latinović, M., Bagge, M., Ojala, A., Berglund, M., Peterson Becher, G., Schoning, K., Hansson, A., Nielsen, L., Clemmensen, L. B., Hede, M. U., Kroon, A., Pejrup, M., Sander, L., Stattegger, K., Schwarzer, K., Lampe, R., Lampe, M., Uścinowicz, S., Bitinas, A., Grudzinska, I., Vassiljev, J., Nirgi, T., Kublitskiy, Y., and Subetto, D.: A Holocene relative sea-level database for the Baltic Sea, Quaternary Sci. Rev., 266, 107071, https://doi.org/10.1016/j.quascirev.2021.107071, 2021.
Rößler, D., Moros, M., and Lemke, W.: The Littorina transgression in the southwestern Baltic Sea: new insights based on proxy methods and radiocarbon dating of sediment cores, Boreas, 40, 231–241, https://doi.org/10.1111/j.1502-3885.2010.00180.x, 2011.
Scheder, J., Engel, M., Bungenstock, F., Pint, A., Siegmüller, A., Schwank, S., and Brückner, H.: Fossil bog soils (“dwog horizons”) and their relation to Holocene coastal changes in the Jade Weser region, southern North Sea, Germany, J. Coast. Conserv., 22, 51–69, https://doi.org/10.1007/s11852-017-0502-z, 2018.
Schlungbaum, G.: Untersuchungen über die Sedimentqualität der Darß-Zingster Boddenkette unter besonderer Berücksichtigung der Stoffaustauschverhältnisse zwischen Wasser und Sediment, Habilitation treatise, University of Rostock, Germany, 129 pp., 1979.
Schumacher, W. and Bayerl, K.-A.: The shoreline displacement curve of Rügen Island (Southern Baltic Sea), Quatern. Int., 56, 107–113, 1999.
Schumacher, W., Lampe, R., Janke, W., Bayerl, K., Reisch, F., Müller, A., and Gusen, R.: Klimaänderung und Boddenlandschaft–KLIBO, Verbundvorhaben des BMFT, Fördernr. 01 LK9405/4, Final project report 1994–1998, 34 pp., 1998.
Schwarzer, K.: 4.2 Beeinflussung der Küstenmorphodynamik durch Wasserstandsänderungen, in: Die Wasserstände an der Ostseeküste. Entwicklung–Sturmfluten–Klimawandel, edited by: Hupfer, P., Harff, J., and Sterr, H., Die Küste, 66, 223–243, 2003.
Schwarzer, K., Ricklefs, K., Lohrberg, A., and Valerius, J.: Die geologische Entwicklung von Nord- und Ostsee, Die Küste, 87, 343–376, https://doi.org/10.18171/1.087114, 2019.
Sindowski, K.-H.: Gliederungsmöglichkeiten im sandig ausgebildeten Küsten-Holozän Ostfrieslands, Eiszeitalter und Gegenwart, 19, 209–218, 1968.
Slobodda, S.: Landschaftsökologische Kennzeichnung und Typisierung von Bodden-Verlandungsufern an den inneren Seegewässern der DDR unter Einbeziehung vegetationsökologischer Untersuchungen, Habilitation treatise, University of Greifswald, Germany, 156 pp., 1989.
Strahl, J.: Pollenanalytische Untersuchungen von Sedimentkernen aus dem Seegebiet des Greifswalder Boddens (NE-Deutschland, südliche Ostsee), Zeitschrift der deutschen geologischen Gesellschaft, 148, 81–93, 1997.
Stratigraphische Tabelle von Deutschland 20016 (STD 2016): http://www.stratigraphie.de/std/Bilder/5_2.pdf, last access: 14 May 2022.
Streif, H.: Die Profiltypenkarte des Holozän–eine neue geologische Karte zur Darstellung von Schichtenfolgen im Küstenraum für praktische und wissenschaftliche Zwecke, Die Küste, 34, 79–86, https://hdl.handle.net/20.500.11970/101151 (last access: 14 May 2022), 1979.
Succow, M.: 6. Durchströmungsmoore, in: Landschaftsökologische Moorkunde, edited by: Succow, M. and Joosten, H., Fischer, Jena, 365–379, ISBN 978-3-510-65198-6, 2001.
Verse, G.: Sedimentation und paläogeographische Entwicklung des Greifswalder Boddens und des Seegebietes der Greifswalder Oie (südliche Ostsee) seit dem Weichsel-Spätglazial, PhD thesis, University of Greifswald, Germany, 135 pp., 2001.
Verse, G., Niedermeyer, R.-O., Flemming, B., and Strahl, J.: Seismostratigraphie, Fazies und Sedimentationsgeschichte des Greifswalder Boddens (südliche Ostsee) seit dem Spätglazial, Meyniana, 50, 213–236, 1998.
Verse, G., Niedermeyer, R.-O., and Strahl, J.: Kleinskalige holozäne Meeresspiegelschwankungen an Überflutungsmooren des NE-deutschen Küstengebietes (Greifswalder Bodden, südliche Ostsee), Meyniana, 51, 153–180, 1999.
Weerts, H. J. T., Westerhoff, W. E., Cleveringa, P., Bierkens, M. F. P., Veldkamp, J. G., and Rijsdijk, K. F.: Quaternary geological mapping of the lowlands of the Netherlands, a 21st century perspective, Quatern. Int., 133–134, 159–178, https://doi.org/10.1016/j.quaint.2004.10.011, 2005.
Wernicke, W.: Die Küste der Insel Usedom und Wollin vom Peenemünder Haken bis zum Swinhöft, 1. Beiheft zum 47./48. Jahrbuch der Pommerschen Geographischen Gesellschaft 1929/1930, Greifswald, 113 pp., 1930.