the Creative Commons Attribution 4.0 License.
the Creative Commons Attribution 4.0 License.
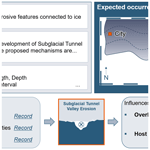
Expected and deviating evolutions in representative preliminary safety assessments – a focus on glacial tunnel valleys
Eva-Maria Hoyer
Anne Bartetzko
Wolfram Rühaak
- Article
(559 KB) - Full-text XML
- BibTeX
- EndNote
Müller, P., Hoyer, E.-M., Bartetzko, A., and Rühaak, W.: Expected and deviating evolutions in representative preliminary safety assessments – a focus on glacial tunnel valleys, E&G Quaternary Sci. J., 72, 73–76, https://doi.org/10.5194/egqsj-72-73-2023, 2023.
Germany gave a fresh start to its site selection procedure for a repository for high-level radioactive waste with the Repository Site Selection Act (StandAG, 2017) in 2017. The procedure consists of three phases; Phase I is split into two steps. BGE published the Sub-areas Interim Report in 2020 to conclude Step 1 (BGE, 2020). Ninety areas passed the geoscientific criteria and were declared sub-areas with a generally favourable geological situation.
To select a site for a high-level radioactive waste repository, the performance of the possible sites as disposal systems that safely contain radionuclides over 1 million years has to be investigated. Hence, in Step 2 of Phase I, representative preliminary safety assessments are an important part of the regulatory toolbox for finding the most suitable areas. They are performed according to the Disposal Safety Analysis Ordinance (EndlSiAnfV, 2020; EndlSiUntV, 2020) of 2020. Their results will help in narrowing down the sub-areas, which currently cover approximately 54 % of the German land surface, to a small number of siting regions that will be subject to surface exploration in Phase II. Preliminary safety assessments are performed in each phase of the German site selection procedure. The representative preliminary safety assessments of Phase I have a limited scope compared to the assessments in Phases II and III (EndlSiAnfV, 2020). For a more detailed overview of the site selection process and the role of the preliminary safety assessments, see Hoyer et al. (2021).
The safety of a disposal system is not only dependent on the present-day geological situation of its site, a good repository design, and an excellent technological implementation during construction and operation of the repository but also especially on the future behaviour of the whole system. Since safety has to be achieved for 1 million years, a well-founded, circumspect estimate of the processes acting in and on the repository becomes vital. In the representative preliminary safety assessments, the focus is on future processes in the geosphere, with the challenge to estimate and attach probabilities and risks to any of these geogenic processes1.
The range of possible evolutions of the system is the core of a safety assessment. They are ordered by likelihood (§ 3 EndlSiAnfV):
-
the expected evolution is the most likely development from the most likely initial state of the disposal system, and
-
deviating evolutions are not expected, but they are considered as far as they cannot be ruled out from occurring in the future.
For now, the geogenic processes are the starting point for developing the evolutions (§ 7 EndlSiUntV). In later phases, all components and processes in the disposal system can be a starting point for deviating evolutions.
Any attempt at forecasting the future is likely to be wrong to some degree. The aim of a safety assessment structured around the scenario method (a particular method of thinking about the future, further explained below) is to optimise the repository in such a way that deviating unexpected developments are very unlikely to compromise the safety of the repository – as far as it is humanly possible to tell. To achieve this, it is necessary to stretch the imagination beyond extrapolating past or current trends and data observations, to examine all assumptions, and to question the sufficiency of the data used.
Sandia National Laboratories first applied the scenario methodology in the context of nuclear disposal in the USA, after the method was previously used for policy strategy development for both governments and businesses (Cranwell et al., 1990). As part of an OECD-NEA (Organisation for Economic Co-operation and Development Nuclear Energy Agency) initiative, several national nuclear waste disposal organisations started using the scenario methodology. Since then, it has become a standard instrument for the development of a safety case (IAEA, 2012).
A scenario study requires
-
a clearly defined scope (area of interest, time period, goals),
-
a compilation of knowledge about the current state (relevant actors, facts, well-known processes and trends in science and society), and
-
an understanding of which elements of the current state are least understood or least predictable in their properties or behaviour while posing the greatest risk to the goals (MacKay and McKiernan, 2018).
In a safety assessment for a potential nuclear waste disposal site, the scope is defined by national regulations. In the German site selection process, the areas of interest are the sub-areas identified in Step 1 of Phase I. The period of interest is 1 million years after closure of the repository; the goal is a disposal system that keeps within the limits of radionuclide mass and number of atoms transported beyond the essential barriers as given by the Disposal Safety Requirements Ordinance (EndlSiAnfV, 2020; StandAG, 2017). Dose calculations are excluded from the representative preliminary safety assessments but will be part of later assessments.
The compilation of knowledge about the current state and trends is limited to a description of the components and processes in the geosphere and the repository by the EndlSiUntV. Future human actions will not be taken into account, and at this stage, it is permitted to assume that the repository was successfully constructed and closed according to the current repository concept. Possible risks related to these aspects will be included in the preliminary safety assessments from Phase II onward.
Safety assessments for potential nuclear waste disposal sites usually build up a database called a “FEP catalogue” to document the description of the features, events, and processes studied in the safety assessment. SKB developed this highly structured system description for their safety assessments (Swedish Nuclear Fuel and Waste Management Co., 1989). Today, the OECD-NEA provides the International FEP List as a starting point or auditing instrument for new safety assessment projects (Capouet et al., 2019).
A catalogue entry of a feature, event, or process will typically include a short definition and a longer description, a statement on whether the element is relevant to the repository and why, the times at which the element is relevant, and its influence on other elements with a record of the reasoning behind the identified influence (Fig. 1).
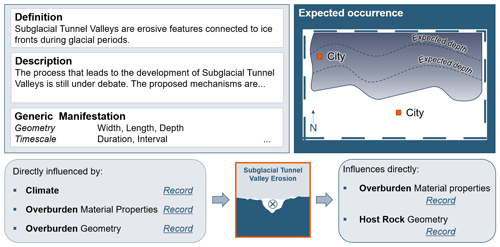
Figure 1Sketch of FEP catalogue entry for the process of subglacial tunnel valley erosion. These are only examples of possible influences within the system. The final FEP catalogue entry in the representative preliminary safety assessments performed by BGE may look different and include different conclusions.
The complete FEP catalogue holds a network of direct and indirect influences that have to be assessed for their safety relevance. This is done by analysing the performance of safety functions of different barriers in the disposal system over time.
The third part of a scenario study is an examination of knowledge, lack of knowledge, and risk. Uncertainties for all features and processes under consideration need to be mapped out and quantified wherever possible.
Subglacial tunnel valley erosion is a geogenic process (occurring independently of the existence of a repository system) that has produced large-scale linear erosional features during past glaciations of what is now Germany (e.g. Weitkamp and Bebiolka, 2017). The process that leads to the development of subglacial tunnel valleys is still under debate (Weitkamp and Bebiolka, 2017). Indications that tunnel valleys are filled in quickly (most commonly with coarse material or locally reworked sediments) but can be reactivated in successive glaciations have been observed (Kuster and Meyer, 1979).
The minimum depth for a containment-providing rock zone (CRZ)2 is 300 m below present ground surface. However, the integrity of the CRZ has to hold for 1 million years. Therefore, the depth of the repository must be below the depth reached by direct and indirect effects of exogenic processes, and there must be no reasonable doubt about the long-term integrity of the barriers in the disposal system, particularly the host rock (§ 23 StandAG). If subglacial tunnel valley erosion can be expected to occur with any glaciation, the likely intensity of the process (erosional depth) is the key to estimating whether the siting of a repository at a certain location and depth can ensure the long-term integrity of the CRZ.
In the safety assessment, the process will first be entered into the FEP catalogue (Fig. 1). Its definition distinguishes it from other forms of erosion. The description contains a summary of the current understanding of the process in the scientific community, as well as the areas affected by the process in the past.
Next, the properties of features in the catalogue that can directly influence the manifestation of the process are identified, as are the properties of features that are in turn directly affected by the process. Tunnel valley erosion can locally reduce the thickness of the overburden of the CRZ, as well as change the permeability when the valley is filled up again. Assuming, for instance, 10 future glaciations over 1 million years, then multiple potential erosion “events” can affect a potential disposal system.
Uncertainty about the processes that form subglacial tunnel valleys could invite the definition of a vertical safety margin, for example 100 m. This strategy, though, has issues: to start with, increasing the depth of the repository may offer more long-term safety, but it can increase short-term risks during the operational phase, depending on the host rock, since the drilling of the mine can become more difficult.
Another issue is the problem of missing knowledge (epistemic): how can we know that we have observed the deepest possible tunnel valley? For example, in the Netherlands, a maximum erosion depth of 500 m was known. Reprocessing of existing seismic data, however, led to the discovery of “new” tunnel valleys with depths of nearly 600 m below ground surface (Ten Veen, 2015). This necessitated a re-evaluation of the risk tunnel valley erosion posits for radioactive waste disposal, increasing the maximum observed depth by approximately 100 m.
The deepest known tunnel valley in Germany is the so-called Hagenower Rinne with a depth of 584 m below present ground surface (Kuster and Meyer, 1979), but there is no way of knowing at this time whether that is the deepest valley. Tunnel valley research in the North Sea allows for the conclusion that tunnel valleys will be found when specifically (re)processing data, e.g. seismic data (e.g. Lutz et al., 2009). An absence of tunnel valleys on a thematic map does not imply that there are none present. It may just mean no appropriate study has been conducted there to gain significant data, or existing data were not analysed for this special purpose.
This leads to our understanding of the risks of tunnel valley erosion and how we assess which evolution is expected and which is deviating. It becomes hard to judge whether a safety buffer of 100 m below the deepest known tunnel valley is actually a sensible conservative measure. To account for this, Weitkamp and Bebiolka (2017) suggest zones of different representative predicted depths of erosion. Even so, this could either exclude possibly safe sites from further consideration by strongly overestimating tunnel valley erosion depths or include too many by underestimating them.
Considering the future with scenario methods should inevitably call into question the extent and certainty of our knowledge and understanding regarding both the past and the present. Particularly in high-reliability institutions such as a disposal system for high-level radioactive waste, the consequences of error need to be minimised as far as possible. A disposal system needs to be sited and conceptualised with sufficient safety reserves to accommodate a range of future evolutions beyond the expected evolution.
No data sets were used in this article.
PM: conceptualisation, writing – original draft preparation. AB: writing – review and editing, supervision. EMH: conceptualisation, writing – review and editing. WR: supervision, writing – review and editing.
The contact author has declared that none of the authors has any competing interests.
Publisher’s note: Copernicus Publications remains neutral with regard to jurisdictional claims in published maps and institutional affiliations.
This article is part of the special issue “Subglacial erosional landforms and their relevance for the long-term safety of a radioactive waste repository”. It is the result of a virtual workshop held in December 2021.
We thank all the colleagues that have contributed and will contribute in the process of nuclear waste site selection. Their contributions are most important and valued. We especially thank the editor and reviewer for their input.
This paper was edited by Sonja Breuer and reviewed by Axel Weitkamp.
BGE: Sub-areas Interim Report pursuant to Section 13 StandAG, Bundesgesellschaft für Endlagerung mbH, Peine, SG01101/16-1/2-2021#1, https://www.bge.de/fileadmin/user_upload/Standortsuche/Wesentliche_Unterlagen/Zwischenbericht_Teilgebiete/Zwischenbericht_Teilgebiete_-_Englische_Fassung_barrierefrei.pdf (last access: 1 August 2022), 2020.
Capouet, M., Carter, A., and Ciambrella, M.: International Features, Events and Processes (IFEP) List for the Deep Geological Disposal of Radioactive Waste, Version 3.0, OECD-NEA, Report No. NEA-RWM-R–2019-1, Paris, France, 166 pp., 2019.
Cranwell, R. M., Guzowski, R. W., Campbell, J. E., and Ortiz, N. R.: Risk Methodology for Geologic Disposal of Radioactive Waste: Scenario Selection Procedure, US Department of Energy, NUREG/CR-1667 SAND80-1429, Sandia National Laboratories, Albuquerque, New Mexico, USA, 1990.
EndlSiAnfV: Endlagersicherheitsanforderungsverordnung vom 6. Oktober 2020 (BGBl. I S. 2094), ISSN 0341-1095, 2020.
EndlSiUntV: Endlagersicherheitsuntersuchungsverordnung vom 6. Oktober 2020 (BGBl. I S. 2094, 2103), ISSN 0341-1095, 2020.
Hoyer, E.-M., Kreye, P., Lohser, T., and Rühaak, W.: Preliminary safety assessments in the high-level radioactive waste site selection procedure in Germany, Saf. Nucl. Waste Disposal, 1, 37–38, https://doi.org/10.5194/sand-1-37-2021, 2021.
IAEA: The Safety Case and Safety Assessment for the Disposal of Radioactive Waste: No. SSG-23, IAEA Safety Standards, International Atomic Energy Agency, Vienna, Austria, ISBN 978-92-0-128310-8, 2012.
Kuster, H. and Meyer, K.-D.: Glaziäre Rinnen im mittleren und nördlichen Niedersachsen, E&G Quaternary Sci. J., 29, 135–156, https://doi.org/10.3285/eg.29.1.12, 1979.
Lutz, R. K., Gaedicke, C., Reinhardt, L., and Winsemann, J.: Pleistocene tunnel valleys in the German North Sea: spatial distribution and morphology, Z. Dtsch. Ges. Geowiss., 160, 225–235, https://doi.org/10.1127/1860-1804/2009/0160-0225, 2009.
MacKay, R. B. and McKiernan, P.: Scenario thinking: A historical evolution of strategic foresight, Elements in business strategy, Cambridge University Press, Cambridge, 83 pp., ISBN 9781108571494, 2018.
StandAG: Standortauswahlgesetz vom 5. Mai 2017 (BGBl. I S. 1074) (das zuletzt durch Artikel 1 des Gesetzes vom 7. Dezember 2020 (BGBl. I S. 2760) geändert worden ist, ISSN 0341-1095), 2017 (updated 2020).
Swedish Nuclear Fuel and Waste Management Co.: The joint SKI/SKB scenario development project, Swedish Nuclear Fuel and Waste Management Co., Report No. SKB TR 89-35, Stockholm, Sweden, 176 pp., 1989.
ten Veen, J.: Future evolution of the geological and geohydrological properties of the geosphere: OPERA-PU-TNO412, Task 4.1.2, Centrale Organisatie foor Radioactief Avfal, Vlissingen, NL, 2015.
Weitkamp, A. and Bebiolka, A. C.: Subglaziale Rinnen – Darstellung und Bewertung des Kenntnisstandes, Bundesanstalt für Geowissenschaften und Rohstoffe, Hannover, Pleistozäne übertiefte Strukturen und ihre Bedeutung für die Langzeitsicherheit möglicher Endlagerstandorte in Süddeutschland, B3.4/B50112-44/2016-0004/002, 36 pp., 2017.
A term newly used in the context of nuclear waste disposal in the Site Selection Act. It is interpreted as those geogenic processes that would occur independently of the repository and is used to distinguish them from technogenic processes, which are dependent on the existence of the repository structures.
The part of the host rock that ensures the safe containment of the radioactive waste in interaction with technical and geotechnical barriers (§ 2 Nr. 9 StandAG).
- How to cite
- Introduction
- Evolutions of the disposal system
- Example – subglacial tunnel valley erosion
- Consequences of expected and deviating evolutions
- Conclusions
- Data availability
- Author contributions
- Competing interests
- Disclaimer
- Special issue statement
- Acknowledgements
- Review statement
- References
representative preliminary safety assessmentsthe possible future evolutions of potential disposal sites will be developed from our understanding of their past evolution. Erosion processes connected to glaciations can reach especially deep and could threaten a repository, while being very hard to predict. This makes them important to the site selection process.
- How to cite
- Introduction
- Evolutions of the disposal system
- Example – subglacial tunnel valley erosion
- Consequences of expected and deviating evolutions
- Conclusions
- Data availability
- Author contributions
- Competing interests
- Disclaimer
- Special issue statement
- Acknowledgements
- Review statement
- References