the Creative Commons Attribution 4.0 License.
the Creative Commons Attribution 4.0 License.
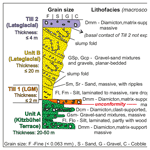
Subglacial deformation and till formation in a stratigraphic complex Late Pleistocene sequence (Einödgraben/Aurach, Kitzbühel Alps, Austria)
Jürgen M. Reitner
John Menzies
Reitner, J. M. and Menzies, J.: Subglacial deformation and till formation in a stratigraphic complex Late Pleistocene sequence (Einödgraben/Aurach, Kitzbühel Alps, Austria), E&G Quaternary Sci. J., 73, 101–116, https://doi.org/10.5194/egqsj-73-101-2024, 2024.
Knowledge of subglacial conditions is of great relevance in understanding glacier dynamics. A combination of micro- and macrosedimentological analysis of diamictons and deformation structures can form the basis for the reconstruction of past subglacial conditions. We present the results of such a study on subglacial tills, within an Alpine environment, at Einödgraben in the Kitzbühel Alps (Tyrol, Austria). The Late Pleistocene succession there (MIS 5d–MIS 2) shows great diversity in facies from alluvial and glaciolacustrine deposits to subglacial deposits. Two glaciogenic diamictons (tills) within the sequence were analysed at the microscale and are correlated to the Last Glacial Maximum (LGM; Würmian Pleniglacial) and the early Lateglacial phase of ice decay. The first deformation phase of pre-LGM deposits most likely occurred in a subglacial setting close to the advancing glacier margin and resulted in diapir-like glaciotectonic macrostructures, which are unique to an inner-alpine area. Subglacial erosion over these structures occurred, and later pre-LGM-emplaced deposits underwent deformation and partial homogenisation immediately beneath the glacier base, leading to diamictons, indicative of subglacial deformable bed conditions. The tills of the LGM and the Würmian Lateglacial show a range of microfacies and deformation structures that are evidence of close and rapid changes in till rheology and the stress field dynamic in the subglacial environment. Our study demonstrates the need for a reinvestigation of deposits occurring in the proximity of past active ice interfaces. The paleoglaciological evidence assembled from the detailed and spatially close research on the microsedimentology of tills at Einödgraben reflects our increasing comprehension and understanding of till microsedimentology in Alpine environments. An awareness is also shown of the need for much further research on the glacial depositional mechanics in mountainous terrains that are different from those in the immense lowland plains of the extensive paleo-ice sheets of North America and northern Europe.
Die Kenntnis der subglazialen Bedingungen ist für das Verständnis der Gletscherdynamik von großer Bedeutung. Eine Kombination aus mikro- und makrosedimentologischer Analyse von Diamikten und Deformationsstrukturen bildet die Grundlage für die Rekonstruktion vergangener subglazialer Bedingungen. Wir stellen die Ergebnisse einer solchen Studie an subglazialen Ablagerungen in einem alpinen Umfeld vor, und zwar vom Einödgraben in den Kitzbüheler Alpen (Tirol/Österreich). Die dortige spätpleistozäne Abfolge (MIS 5d-MIS 2) zeigt eine große Faziesvielfalt von alluvialen über glaziolakustrinen bis zu subglazialen Ablagerungen. Zwei glaziogene Diamikte innerhalb der Abfolge wurden auf der Mikroskala analysiert und mit dem Letzten Glazialen Maximum (LGM; Würm-Hochglazial) und der Eiszerfallsphase im frühen Würm-Spätglazial in Verbindung gebracht. Die erste Deformationsphase der prä-LGM-Ablagerungen fand höchstwahrscheinlich in einem subglazialen Umfeld in der Nähe des vorrückenden Gletscherrandes statt und führte zu diapirartigen glaziotektonischen Makrostrukturen, die für ein inneralpines Gebiet einzigartig sind. Über diesen Strukturen fand subglaziale Erosion statt, und die vor dem LGM abgelagerten Ablagerungen wurden unmittelbar unter der Gletscherbasis deformiert und teilweise homogenisiert, was zu Diamikten führte, die auf ein subglazial deformierbares Gletscherbett hinweisen. Die Grundmoränenablagerungen des LGM und des Würm-Spätglazials weisen eine Reihe von Mikrofazies und Deformationsstrukturen auf, die auf kleinräumige und rasche Veränderungen in der Rheologie der glaziogenen Sedimente und der Dynamik des Spannungsfeldes in der subglazialen Umgebung hinweisen. Unsere Studie zeigt die Notwendigkeit einer erneuten Untersuchung von Ablagerungen, die in der Nähe vergangener aktiver Eisränder auftreten. Die paläoglaziologischen Beweise, die aus der detaillierten und räumlich engmaschigen Erforschung der Mikrosedimentologie der Grundmoränenablagerungen vom Einödgraben zusammengetragen wurden, spiegeln unser zunehmendes Verständnis der Mikrosedimentologie der glaziogenen Sedimente im alpinen Umfeld wider. Es wird auch deutlich, dass die Bildungsmechanismen der glazialen Ablagerungen in Gebirgslandschaften, die sich von denen in den riesigen Tiefebenen der ausgedehnten Paläo-Eisschilde Nordamerikas und Nordeuropas unterscheiden, weiter erforscht werden müssen.
- Article
(12688 KB) - Full-text XML
- BibTeX
- EndNote
The complex records of Pleistocene glaciations of the European Alps are well documented, but many detailed questions remain concerning the sedimentology of many alpine sediments within glacial systems (e.g. Swift et al., 2002; Ivy-Ochs et al., 2022a, b; Reitner, 2022). Glacial overdeepenings, sedimentation, proglacial lake formations and disappearances are only part of this often fragmentary and multifaceted geological history (Buechi et al., 2018). With respect to various subglacial tills, indicative of ice mass advances as well as ice lobe and ice stream activity throughout the region, considerable effort has been devoted to understanding till types, basal ice conditions and stratigraphic interrelationships (e.g. Reitner et al., 2010, 2016; Menzies and Reitner, 2016; Buechi et al., 2018; Reitner and Menzies, 2020; Kamleitner et al., 2022; van Husen and Reitner, 2022). The study reported here is in an area south of Kitzbühel (Austria), lying between the Inn Valley to the north and the Salzach Valley to the south, in the Eastern Alps. Often only fragments or patches of tills can be examined in mountainous terrains, but occasionally larger units of tills within stratigraphic sequences permit detailed macro- and microsedimentological analyses. This paper focusses on the microsedimentology of an exposure of two subglacial diamictons at Einödgraben.
The Einödgraben Valley, a tributary of the Jochberger Ache, is some 8 km south of Kitzbühel and part of the Achen ice lobe (Chiemsee ice lobe) (Reitner et al., 2010) (Fig. 1). The sediments at Einödgraben identified at the macroscale (Waltl, 1995; Reitner and Draxler, 2002; Reitner, 2005) include a 60 m thick sequence of Late Pleistocene sediments. This site provides insights into subglacial paleo-dynamics of subglacial till deposition within an Alpine environment. The study illustrates the ongoing processes at the base of an ice mass where subglacial tills are emplaced over highly erodible and deformable underlying sediments, resulting in the development of a proto-till subfacies (Evans, 2017; Phillips and Hiemstra, 2022). Our findings highlight the importance of deformable beds (Boulton, 1982; Menzies, 1989; Alley, 1991; van der Meer et al., 2003; Menzies et al., 2018) for the understanding of glacier motion in Alpine valleys, which have not been considered in recent ice flow models for the Alps (e.g. Seguinot et al., 2018). Evidence from Austria and Switzerland indicates that most ice streams and lobes within deeply incised Alpine valleys were underlain by deforming subglacial sediments (Menzies and Reitner, 2016; Buechi et al., 2017; Reitner and Menzies, 2020). These subglacial deforming beds provide sediment fluxes, active traction conditions ideal for subglacial bedform formation and forward ice motion in many locations, such as the valleys around Wildschönau, Wilder Kaiser, and Ainet (Menzies and Reitner, 2016, 2019; Reitner and Menzies, 2020; for locations see Fig. 1). This paper provides evidence, in the form of mapped information relevant to the structural geology, of subglacial tills that may be applied to tills throughout the past-glaciated European Alps and beyond.
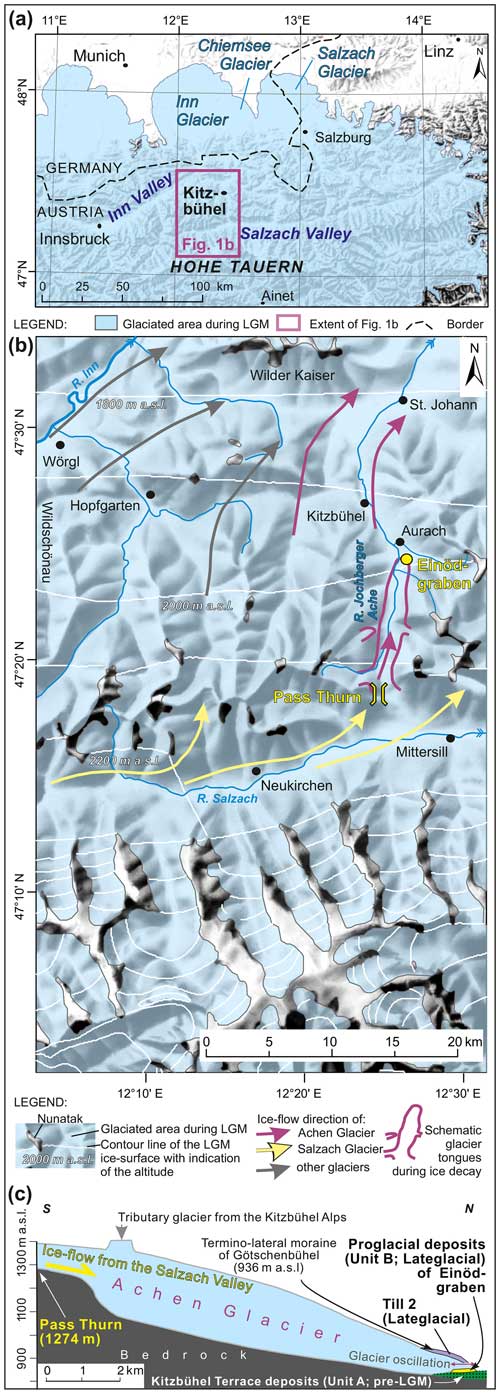
Figure 1(a) Overview map with the LGM ice extent (according to Ehlers et al., 2011) and the indication of the study area and surroundings as shown in panel (b). (b) Ice flow geometry with the ice flow pattern, ice-surface contours and nunataks during LGM (according to van Husen, 1987) and the schematic extent of the glacier tongue in the valley of Jochberger Ache during the phase of ice decay in the early Lateglacial. The location of the Einödgraben site is indicated. (c) Geometry of the glacier tongue during the phase decay in the longitudinal section between the Thurn Pass in the south and Einödgraben in the north (see panel b for location). The main sedimentary units are shown schematically (adapted from Reitner, 2005).
The Einödgraben site, named after the farmhouse Einöd (also called Einathen), is located on the eastern flank of the Jochberg Valley in the community of Aurach within the Kitzbühel Alps (Eastern Alps, Austria) (Figs. 1, 2). The main sampling sites are E1–4 (47.40646° N, 12.43346° E) and E5 (47.40584° N, 12.43256° E), with additional outcrops at E-X (47.40673° N, 12.43210° E) and E-Y (47.40766° N, 12.43097° E) occurring at an elevation between 830 and 855 m a.s.l. along the Einödgraben creek. The Kitzbühel Alps with peaks in the surroundings of around 2400 m a.s.l. are tectonically part of the Austroalpine Superunit (Schuster et al., 2014) with a bedrock lithology composed of Paleozoic meta-sediments and meta-magmatites (Heinisch et al., 2003, 2015).
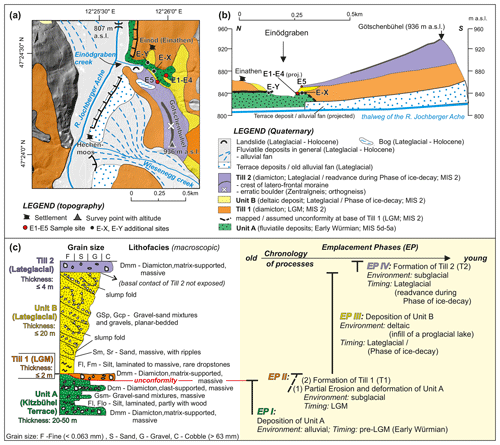
Figure 2(a) Map of the Quaternary geology at Einödgraben and surroundings (modified after Heinisch et al., 2003). Sampling sites E1–E5 and additional important sites are indicated. Areas not covered by Quaternary units are made of bedrock. (Shaded relief image from the TIRIS online map of the province of Tyrol: https://www.tirol.gv.at/, last access: 1 July 2023.) (b) S–N section in the axis of the Jochberger Ache Valley between Götschenbühel (for location see panel a) and Einödgraben with sampling and further important sites indicated. (c) Idealised lithological log of the different lithological units and indication of the corresponding emplacement phases (EPs).
The Einödgraben Quaternary sequence represents a complex inner-alpine stratigraphic sequence of the Last Glacial Cycle (Fig. 2). The sequence starts in the Early Würmian (MIS 5d–a; Chaline and Jerz, 1984) with the wood-bearing deposits of the Kitzbühel Terrace and comprises one of the stratigraphically best-constrained transitions from the LGM to the early deglaciation (phase of ice decay) in the early Lateglacial with two till layers (Reitner, 2005; Heinisch et al., 2015). The LGM, in this area of Austria, was characterised by strong ice transfluence from the east-to-west-trending Salzach Valley to the north over the Thurn Pass (1248 m) bringing ice containing Zentralgneis erratics into this central segment of the Jochberger Ache basin (Fig. 1). In this phase of deglaciation, up to 1200 m thick ice decayed rapidly, and a small, mostly (dead) ice-dammed lake was formed. During a small and most probably short oscillation, the last remnant of ice advanced over the previously deposited lake infill. In this last glacial phase, the ice transfluence towards the north was for the last time active after the LGM (Fig. 1).
Macroscopical logging of exposures was done using lithofacies codes of Keller (1996), and grain-size and grain-shaped information was obtained during the study of the regional Quaternary geology of this area of Austria (Reitner, 2005). Undisturbed, oriented bulk samples from five sites (E1–E5; Fig. 2) at Einödgraben were collected in the field for microsedimentological study of thin sections (van der Meer 1993, 1996; Rice et al., 2013, 2014; Menzies et al., 2018). Samples used Kubiëna tins and bulk samples, both taken parallel to the ice flow direction (see Rice et al., 2014, for detailed field collection and thin-section production). In total, 65 samples were collected from Unit A, Till 1 (T1) and Till 2 (T2) at different sites (16 from E1, 9 from E2.1, 10 from E2a, 7 from E3, 5 from E4 and 18 from E5; for stratigraphy and location see Figs. 2 and 3). No samples were taken of Unit B as the sediments were too loose and friable. Analysis of thin sections collected from Einödgraben was completed at low magnification (6.5–10X) in plain and cross-polarised light using a Wild Heerbrugg® M420 microscope affixed with a Nikon® Digital Sight DS-Fi1 digital imaging device and using Nikon® NIS-Br imaging software. All microstructures were mapped utilising CorelDraw 20®, Photoshop Elements®, JMicroVision® and StereosStat® software (see Phillips et al., 2011; Menzies, 2023). In addition, the orientation of microshears (ms's) was calculated and plotted. The analyses provide approximate estimates of strain signatures and a chronosequence of microstructure development.
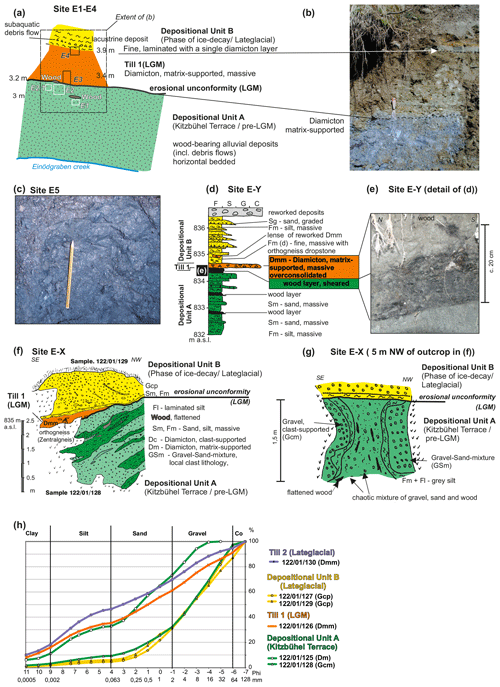
Figure 3Field sketches and images from the Einödgraben. (a) Sketch with geological units and stratigraphy at the sampling site of samples E1, E2, E2.1 (Unit A), E3 (Till 2) and E4 (Unit B). The extent of the area covered by the image in panel (b) is indicated. (b) Image of panel (a) with the three units. Note the sharp erosional contact between Unit A and Till 1. (c) Sampling site of E5 (matrix-supported and massive diamicton, Till 2), approximately 18 m above the site of E1–4 (the length of the spatula is 22 cm). (d) Sediment log at site E-X with the stratigraphy and facies. Note the occurrence of sheared wood (Unit A) at the base of Till 1. The stratigraphic range of Fig. 3e is indicated. (e) Image of outcrop at site E-X: a piece of wood marks the top of the unit overlain by a grey matrix-supported and massive diamicton (Till 1). Note the sheared appearance of the wood piece with signs of disintegration towards the diamicton indicated by isolated wood pieces within Till 1. (f) Field sketch showing the stratigraphy, facies and geometry of Unit A, Till 1 (with orthogneiss boulders from the Hohen Tauern range), and Unit B at site E-X, east of sampling sites E1–4. The location of the grain-size sample 122/01/125 is shown in panel (h). Note the tilted dip of Unit A due to glaciotectonic deformation and the disappearance of Till 1 towards NW where only the erosional unconformity (LGM) at the base of Till 1 is evident. (g) Field sketch of a temporal outcrop just west of E-X. Unit B shows a vertical to overtopped layering. This structure looks like a truncated diapir. Sharp contact is made with Unit B, which overlays the LGM erosional unconformity. The location of the grain-size sample 122/01/128 is shown in panel (h). (h) Diagram with grain-size distributions of the coarse-grained facies of the different lithological units (adapted from Reitner, 2005).
At this site in Einödgraben the term emplacement phase (EP) was used to specifically identify the lithofacies within the stratigraphic column where sedimentation and/or deformation had occurred. In the past, the term deposition had perhaps been overused in glaciogenic successions since the term is often too broad (Evans, 2017). Where sediment has become “inert” following transport in the subglacial environment, the term deposition perhaps does not impart the concept of deposition through stress immobilisation of soft deforming sediment following subglacial advection of sediments (Menzies, 2003; Evans, 2017). By using the term emplacement, the authors wish to convey the idea that the subglacial till has become immobilised due to a change in internal bulk shear strength or external stress levels. Each emplacement phase, where appropriate, is assigned to one or two lithofacies units; thus a chronosequence of these phases can be identified (Fig. 2c).
4.1 Lithofacies description and interpretation
- 1.
Unit A, stratigraphically the lowest, is the Kitzbühel Terrace, which has a thickness ranging between 20 and 50 m (Fig. 2c). It consists of poorly sorted gravel, matrix- and clast-supported diamictons with a sandy to fine-gravelly matrix, and massive to laminated fines (clayey silt) (Fig. 3a, b, d, g, h). The clast lithology is of local provenance (greywacke zone), and roundness varies between angular and subrounded. Flattened wood pieces (Fig. 3a, d, e), mostly of the Picea/Larix type (Waltl, 1995), occur in all samples. The originally horizontally bedded strata are slightly tilted (Fig. 3e). Occasionally, “truncated” diapir structures are present (Fig. 3f).
- 2.
Till 1 (T1), at the macroscale, is a dark grey, overconsolidated massive matrix-supported diamicton (Fig. 3a, b, h) with subangular to subrounded, facetted and striated clasts. The Zentralgneis–orthogneiss represents up to 2 % of the clast lithology, which is dominated by the local greywacke lithologies. The contact with the underlying sediments is sharp (Figs. 2c and 3a, b, f). Sheared wood (see above) at the boundary indicates the erosive nature of the unconformity where the 0.5 to 2 m thick T1 is not always present (Fig. 3f, g). The tilting of Unit A (Fig. 3f) and the formation of diapir-like structures (Fig. 3g) are regarded as evidence of glaciotectonism. In the areas to the north of Einödgraben, T1 occurs in partly drumlinised areas on top of the terrace (see Heinisch et al., 2003). In such places and south of Einödgraben the thickness of T1 may reach ≥ 20 m (see Fig. 2b).
- 3.
Unit B consists of a coarsening upward sequence beginning on top of T1 with laminated silt, sandy silt and ripple-bedded sand, with occasional dropstones and lenses of grey diamictons (Figs. 2c and 3a, b, d). The unit is ≤ 20 m thick with a fine sediment layer sealing the unconformity where T1 is missing (Fig. 3f, g). Gravel–sand mixtures (Fig. 3h for grain-size distribution) make up the upper part of Unit B with occasional orthogneiss within the predominantly local clast lithology (Fig. 3e). Clinoforms are rare, and the stratification appears chaotic with occasionally recognisable slump folds. Only in the southern portion of the Einödgraben creek is Unit B covered by a ≥ 4 m thick grey, overconsolidated massive matrix-supported diamicton (Till 2; T2) containing subangular to subrounded clasts (Figs. 2c, 3c, h). In the lower part of the unit, two 2–3 cm thick layers of laminated silts occur within the diamicton where greywacke clasts with occasional shear planes were identified. The T2 diamicton can be traced southward to the front of the end moraine at Götschenbühel (Aurach; Fig. 2), which most likely documents a short glacier halt during ice decay (Patzelt, 1975; Reitner, 2005). Diamictons along the flank of the moraine contain rare but significant Zentralgneis clasts (Fig. 2b).
4.2 Subdivision in emplacement phases (EP I–EP IV)
Unit A corresponds to EP I (Fig. 2c). Based on the macrofacies sedimentology together with the wood content and pollen analyses (Reitner and Draxler, 2002; Reitner, 2005), EP I is characterised by overbank and fluvial deposits interfingered with debris flow deposits from smaller tributary creeks. Such an alluvial environment is typical of modern Alpine valley floors. According to the organic content, an obtained 14C age of > 50 ka shows Unit A is of Early Würmian (MIS 5d–a) age (see Heinisch et al., 2015).
Till 1, as the product of EP II (Fig. 2c), is a subglacial till lying above the unconformity that separates it from the underlying Unit A and was deposited by the ≥ 1200 m thick Achen glacier fed by an ice stream overtopping Thurn Pass as a diffluent lobe (Fig. 1b). From the evidence in the Kitzbühel Alps (Reitner et al., 2010) and the basal unconformity indicating a major hiatus, T1 is of LGM age (MIS 2) (Fig. 2c). Based on field evidence, the glaciotectonic deformation occurred during EP II, prior to the formation of T1.
In the context of a major deglaciation phase, at the onset of the Würmian Lateglacial just after the LGM (Reitner, 2007), a small proglacial lake was formed and infilled by delta deposits, which led to the formation of Unit B during EP III (Fig. 2c). During this ice decay, a short oscillation of the remnants of the still-active Achen glacier resulted in a spatially limited overriding of the previously deposited glaciolacustrine Unit B (Fig. 1b, c). Finally, during EP IV (Till 2; T2), a subglacial till was formed when a restricted ice flow came over Thurn Pass (Fig. 2b, c).
5.1 Descriptions
The site at Einödgraben contains two subglacial tills (Fig. 2c) with stratified sediments below and above the lower till (T1) and below the upper till (T2), providing a useful stratigraphic complex of contact interfaces and depositional processes (Figs. 2, 3). At each sampling location, several samples were taken to reflect the variation in till sedimentology across the sites.
5.1.1 Unit A (EP I and II)
Sample E1.1a was taken from pre-LGM alluvial sediments (Unit A) ∼ 0.2 m below the lower contact with the lower till (T1) (Fig. 3a). This is a fine-grained diamicton containing many wood fragments (Fig. 4). The sediment clearly exhibits a deformed domain curving around the large subrounded clast on the left-hand side of the image. Much of the domain contains long, attenuated segments of organics within a slightly coarser sandy unit. The single large clast of metasandstone is derived from the bedrock in the catchment of the Jochberger Ache river. Occasional grain stacks can be seen within the deformed domains as well as small truncated microshears (Fig. 4a, b, c).
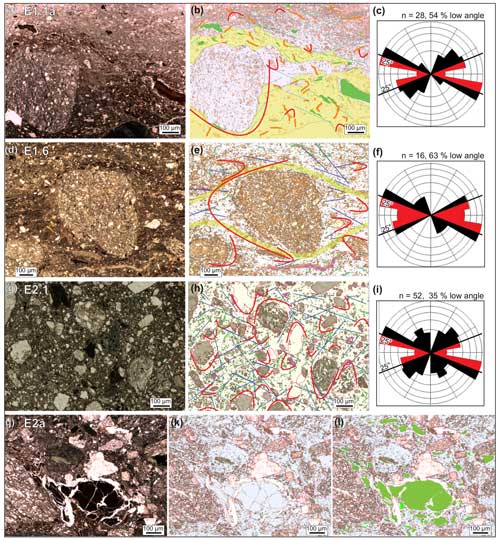
Figure 4Mapped images of thin sections from the Einödgraben site samples E1.1a (a, b, c), E1.6 (d, e, f), E2.1 (g, h, i) and E2a (j, k, l). Note the presence of organic fragments (green) in panel (b) that can also be seen in panel (l). Two large rotation structures are also visible in panels (a), (b), (d) and (e), while panels (g) and (h) show many rotations indicative of intense non-pervasive deformation. Microshear fabrics in panels (c), (f) and (i) exhibit 64 %, 63 % and 35 % of shear below the 25° angle of high-stress-level-intensity shearing. The latter reduced value might be due to the large number of clasts that may “lock” the deformation process and reduce shearing. Note in panels (d) and (e) purple ovals indicate edge-to-edge crushing events. All thin sections are photographed in plain light at the top of the thin section. The scale appears on the plain-light original images of each thin section at the bottom right. In all stereo fabric diagrams, microshears with angles of < 25° are in red and all others in black. The number of microshears mapped are shown as n, and the percentages of microshears < 25° are shown. Grain stacks are shown as green lines, microshears as blue lines and rotation structures as curvilinear red lines. Organic domains are shown in light green, while all other domains are typically in yellow, light brown, light orange or light pink.
Sample E1.6 was taken close to E1.1, containing a large central rotated subrounded clast (metasandstone). A series of short clay lineaments occur to the left of the large clast, and below the clast a broken ribbon of clay occurs. A domain of deformed fine sandy silt appears within which the large clast in the centre has been “included” during deformation (Fig. 4d, e, f). The large clast appears to have rotated clockwise within the deforming matrix.
Sample E2.1 was taken approximately 10 cm above and to the left of sample E1 within the pre-LGM alluvial sediment (Fig. 3a). The sample exhibits microshears, grain stacks and multiple rotation structures throughout (Fig. 6g, h, i).
Sample E2a, taken to the right of sample E2.1, shows a coarse diamicton with many subangular to subrounded large clasts (mostly metasandstone) and a large wood fragment and scattered woody fragments trailing to the upper left of the large wood particle (Fig. 4j, k, l). This sediment contains no grain stacks, microshears or rotation structures. The clasts exhibit obvious signs of fracture and rupture (Fig. 4k); however there is a flow movement of clasts and the matrix through the sediment.
5.1.2 Till 1 (EP II)
Samples E3.4a and E3.6 occur immediately above the macroscale interface between the lower alluvial deposit (Unit A) and T1. Sample E3.4a shows a distinctive diamicton with subangular clasts (various lithologies of the greywacke zone) within a fine-grained matrix (Fig. 5a, b c). A finer-grained domain can be observed over the right part of this image. Many grain stacks, microshears, edge-to-edge crushing events and rotation structures occur, the latter largely around the larger clasts.
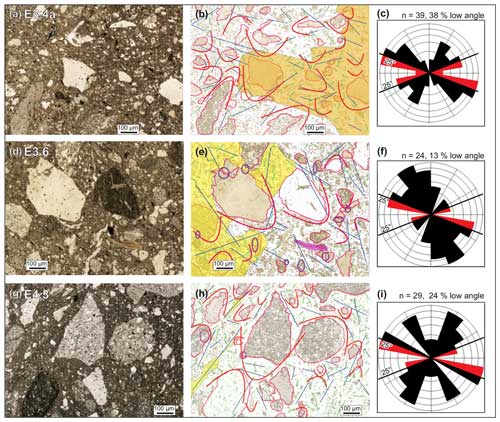
Figure 5Mapped image domains occur across the left side of the image of thin sections from the Einödgraben site samples E3.4a (a, b, c), E3.6 (d, e, f) and E4.3 (g, h, i). Note the oblique shearing in all the images and the cross shearing for example in panels (a) and (b). Note the organic fragments (in purple) in panels (d) and (e). These samples all contain large numbers of rotation structures and a high clast content. Like Fig. 4, the microshear fabrics in these samples show shear angles < 25° in relatively low numbers (38 %, 13 % and 24 %).
In sample E3.6 a similar clast and matrix interrelationships can be observed, including a shard of woody material at the lower right of the image. A finer-grained domain occurs across the left side of the image (Fig. 5d, e, f), and grain stacks, microshears and rotation structures are noted. Unusually, there are numerous edge-to-edge grain-crushing events in this sample.
Sample E4.3 was taken at the top of the diamicton (T1) (just below the truncation) close to the contact with the overlying lacustrine sediments (Unit B) that contain debris flow materials (Fig. 5g, h, i). A fine-grained matrix, which contains both subangular and rounded clasts (mostly metasandstone), occurs with a distinctive pattern of smaller subhorizontal microshears, with other larger microshears exhibiting a near-vertical orientation. Many grain stacks, microshears and rotation structures can be seen, the latter occurring around the axes of larger clasts showing a distinct “up-shearing” – like a pattern in alignment with the larger microshears at a near-vertical preferential orientation. Finally, a narrow ribbon of fine-grained lenses can be seen parallel to a large microshear along the left side of the thin section.
5.1.3 Till 2 (EP IV)
Samples E5.3, 5.4, 5.6 and 5.12 were taken from the upper till (T2) lying some 18 m above the lower till (T1) and immediately above the sequence of glaciolacustrine sediments that, in places, contain debris flow sediments.
Sample E5.3 is a fine-grained diamicton with many subangular and smaller subrounded clasts (Fig. 6a, b, c).
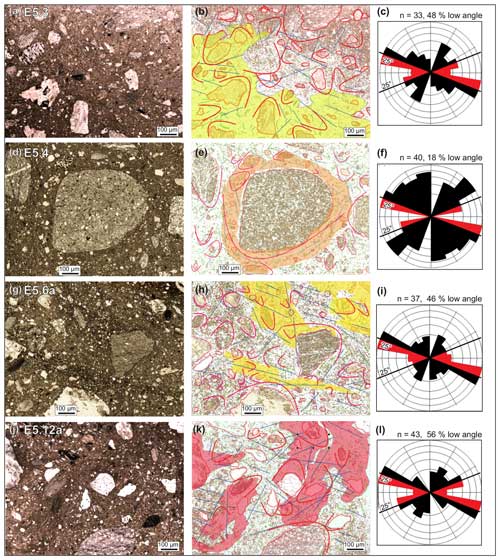
Figure 6Mapped image domains occur across the left side of the image of thin sections from the Einödgraben site samples E5.3 (a, b, c), E5.4 (d, e, f), E5.6a (g, h, i) and E5.12a (j, k, l). Note the large rotation structures in panels (d) (e), (g) and (h). Microshear fabrics are low in panels (d) and (e) at 18 % < 25°, as the large central clast likely prevented much localised shearing. In all other samples, shearing in a relatively strongly preferred direction led to high shearing values of 48 %, 46 % and 56 %, indicative of strong fabrics occurring within sheared sediment where clasts are well scattered and not clast-supported.
Sample E5.4 is similar, with a large subrounded clast in the centre of the section surrounded by a zone of heavily deformed diamictons (Fig. 6d, e, f).
Sample E5.6a is a banded diamicton with large subangular clasts within a matrix containing subrounded clasts and thin clay-rich zones (Fig. 6g, h, i). A complex set of domains occurs throughout this thin section, and near-parallel microshears occur along with grain stacks and rotation structures.
Sample E5.12a is typical of diamictons in the upper till (T2), with evidence of deformation, as shown by orientated but fragmented domains, as well as grain stacks, microshears and rotation structures (Fig. 6j, k, l).
5.2 Interpretation of microsedimentological evidence
The samples from Einödgraben exhibit a range of microstructures that permit a detailed interpretation of the sediment, facies and subfacies types. It can be seen, at the microscale, that the two subglacial tills (T1, T2) were both formed from the underlying alluvial and deltaic deposits. The upper part of T1 was subsequently re-eroded or re-worked and thus replaced by penecontemporaneously disrupted glaciolacustrine sediments (Fig. 2c).
The lowest facies at Einödgraben (Unit A), an alluvial fill, consisting of fine- to coarse-grained sediment with embedded organics (pre-LGM ice advance into the valley), was sampled close to the junction of the overlying till (T1). It is very likely that the uppermost part of this alluvium was a stratified sediment deposited prior to the final ice advance. The samples (E1.1, 1.6, 2.1, 21, 3.4 and 3.6) are all in some manner diamictic-like proto-tills, indicating deformation and likely reworking by overriding ice creating a subglacial subfacies (Menzies, 2023; Zoet et al., 2023). The sediment is noticeably disrupted and retains little evidence of alluvial deposition. Instead, evidence of localised deformation characteristics exists, such as microshears, rotation and grain stack microstructures. All these characteristics appear to negate the sediment being a possible debris flow deposit. In sample E1.1, as well as in the presence of organics (likely wood fragments), there are two domains indicative of mixing occurring likely prior to deformation (Fig. 4b). Mixing is corroborated by the presence of microshears exhibiting a bi-polar fabric distribution (Fig. 4c) with primary S1 and secondary C1 Riedel shears present. Microshear fabrics show that 54 % are < 25° to the horizontal, indicative of relatively localised high shear strain (Menzies and Reitner, 2016). This sediment is interpreted as a glaciogenically influenced diamicton composed of extant alluvial sediment heavily affected by overlying ice and overriding strain effects. The sediment is only 0.2 m below the contact with a likely subglacial interface (see Zoet et al., 2023).
In contrast, sample E1.6 contains many small woody fragments, with a relatively long clay stringer (broken into several small units at the right lower side of the image; Fig. 4d, e). The clay may have been inherited from the alluvial sediment that has been disrupted due to overriding ice movement. The microshears bracket the rotated clast in the centre and present a relatively high strain around the clasts (63 % of microshears are ≤ 25°) (Fig. 4f). As in the case of sample E1.1, this sediment is interpreted as a glaciogenically influenced diamicton, a proto-till composed of extant alluvial sediment heavily impacted by overlying ice.
Samples E2.1 (Fig. 4a) and E2a (Fig. 4j) were both collected close to E1.1 within the alluvial facies below the first recognised till (T1). Sample E2.1 is clearly diamictic with grain stacks, microshears and rotation structures (Fig. 4h). The microshear fabric (Fig. 4i) exhibits a strong bi-polar orientation where Riedel shears can be noted, with 48 % of shears < 25°, indicating a relatively high strain across the sediment sample. This sample can be construed as a localised glacially deformed or traction till facies unit (Evans et al., 2006; Evans, 2017).
In sample E2a, large, fragmented units of organic material (wood) draped around a large clast can be observed (Fig. 4j, k). Around the inclusion there are many subangular clasts packed closely together in what appears to be a deformed subfacies unit. This unit is clearly a diamictic subfacies with no grain stacks, microshears or grain rotations present. It might be that this unit has been compacted and intercalated as a unit within the diamictic subfacies just below the ice–bed interface discontinuity. This sample is heavily glacially impacted by overlying glacial stress activities and suggests that the other surrounding samples of E2.1 and E2a are of a similar subfacies type.
Samples E3.4 (Fig. 5a) and E3.6 (Fig. 5d) were collected within the lower diamicton (T1) between approximately 0.2 to 0.4 m above the lower interface with the underlying alluvial sediments. Sample E3.4 was taken approximately 0.2 m above the lower contact and is a characteristic glacial diamicton with all attendant microstructure signatures of grain stacks, microshears and rotation structures (see Phillips et al., 2007; Menzies et al., 2018). This sample contains two domains that precede the development of the microstructures (Fig. 5b). The microshear fabrics indicate that 38 % occur < 25°, evidence of a moderately high-strain effect, with two dominant shearing directions distinctive of Riedel shearing within a deforming matrix, typical of subglacial soft sediment deformation (Fig. 5c).
Sample E3.6 (Fig. 5d) was sampled a few centimetres from E3.4 and shows a glacial diamicton somewhat coarser grained than the latter but with all the characteristic microstructures present (Fig. 5e). Microshear fabrics (Fig. 5f) show a strong, almost unipolar, orientation of shears with only 13 % of shears < 25°, indicating a generally low-strain environment in which this diamicton was emplaced (see Rice et al., 2014). Samples E3.4 and 3.6 are glacial in derivation and emplacement but indicate deposition within a relatively low-strain localised subglacial environment. It might be hypothesised as porewater drained from this diamicton into the underlying alluvial material (although no porewater escape structures were detected); its effective stress was raised, which in this layer would lead to till emplacement. As ice rapidly moved across the lower sedimentary contact and deposited the till under relatively low-strain conditions, more till continued to be accreted (Zoet et al., 2021).
Sample E4.3, as noted above, was taken within the upper part of the lower diamicton (T1) close to the boundary between the till and the upper proglacial glaciolacustrine sediments (Unit B) (Fig. 5g). This sediment carries the characteristics of a subglacial till, with strong evidence of deformation towards the near-vertical, in the sense that the larger clasts, a narrow set of domains and longer microshears suggest such directional motion (Fig. 5h). It is interesting to note that the microshear fabric for this sample is distinctly bi-polar with only 24 % of shears < 25°, indicating a relatively low-shear-strain environment (Fig. 5i).
As no samples could be derived from the glaciolacustrine sediments at Einödgraben (Unit B, EP III), the next set of samples were obtained from the upper till (T2) unit (Fig. 6a, b, c) (EP IV). The diamicton in sample E5.3 (Fig. 6a) shows a glacial diamicton with all characteristic microstructures present, corroborating that it is a subglacial till. Microshear fabrics indicate a bi-polar fabric with 48 % of shears < 25°, indicating a relatively high strain.
Sample E5.4 (Fig. 6d, e, f) is an example of the impact of rotation around a large clast, indicative of likely non-pervasive shear within a subglacial till (Menzies, 2022). Interestingly, microfabric shear patterns of this sample are strongly bi-polar with only 18 % of the shears < 25°. This fabric indicates that the till was sheared at relatively low strain levels. In further analyses, sample E5.6 (Fig. 6g, h, i) (< 25 cm away) carried a strong microshear fabric (48 % of low-angled shear < 25°). In further contrast, sample E5.12 (Fig. 6j, h, l), just a few centimetres below E5.3, shows 56 % of microshears with ≤ 25°, indicating that within a very small space within the till stress conditions varied enormously. The physical proximity of all these samples in this upper till (T2) with quite strongly differing levels of strain raises the interesting issue of rheological fluctuations in confined areas of the till (Rice et al., 2014; Menzies and Reitner, 2016).
In the microsedimentology of subglacial tills, very localised matrix rheologies, changing particle sizes, the interlocking of clasts, local thermal fluctuations and localised microchanges in effective stress levels occur as porewater contents alter and change. These large yet very localised changes all lead to relatively large variations (fluctuations) in viscoplastic flow within a till (see Iverson, 2003; Menzies, 2022). These samples from E5 are especially relevant for illustrating and detailing the complexity of till rheology.
In summary, the results of microsedimentological analyses illustrate not only the complexity of subglacial till within quite closely spaced samples but also the processes and events occurring at both the upper and the lower contacts between tills and overlying and underlying sediments under ice masses in confined mountainous terrain. In most instances where till sedimentation has occurred as a result of a deforming subglacial bed, microstructure signatures that retain the characteristics of subglacial tills are generated. These signatures have the added aspects/signatures of attributes that can be ascribed to the parent sediment over which deformation processes have travelled. In some cases, for example, it is a lack or rarity of certain microstructures, such as edge-to-edge events as in E3.6 or little or no microshears as in E2a, which locally differentiate the tills (Menzies, 2022; Menzies et al., 2023).
The question arises from this detailed study as to what a till is (see Evans, 2017) in terms of sedimentological characteristics. At Einödgraben there are sediments that could be construed as a till. The appearance of these sediments that are otherwise closely associated sediments, which are glacially influenced diamictons, is an important finding. This discovery raises the issue of other glacial diamicton-like sediments and their position within stratigraphic profiles. The complexity and localisation of glacial depositional mechanics in the confined valleys of the European Alps raise the question of a different style of glacial deposition in relation to much of the present literature that has emphasised lowland-style ice sheet glaciation over wide stretches of terrain where side-valley confinement, for example, is rare (Evans, 2017). The depositions of glacial diamictons in the northern European plain or across huge areas of the Laurentide Ice Sheet terrains in Canada and the USA are enormously different from those that occur in mountainous terrains (Menzies et al., 2023). This study reiterates these significant differences, raises new questions and increases the understanding of the mechanics of glacial deposition in mountainous terrains.
The combination of macro- and microsedimentological evidence results in an improved understanding of the glacial development and chronology of processes during the Last Glacial Cycle, especially during the LGM and the following phase of ice decay (both MIS 2). The oldest deposits of the Einödgraben sequence (Unit A) are part of the Kitzbühel Terrace, which are composed of alluvial deposits, chronostratigraphically most likely Early Würmian (MIS 5d–a) in age (Reitner and Draxler, 2002; Reitner, 2005; Heinisch et al., 2015). EP I is evident in the micromorphological analyses only via the general grain size and clast lithology as well as the organic content (wood remains). The original sedimentary structures just below the major LGM unconformity at the base of Till 1 (LGM) were likely erased.
The impact of the lower till (T1) emplacement phase (EP II) and overriding ice on the underlying alluvial sediments can be observed, and the depths (of approximately 3 m) to which strain effects produce diamicton facies units can be detected (see Zoet et al., 2023). In disrupting the underlying sediment, evidence of the effect of deformation processes generated a form of proto-till (diamicton) within the underlying sediment. The impact of deformation led to the partial cannibalisation of underlying sediments and wood fragments (Fig. 3d, e), causing the contact at the microscale to be gradational over of several tens of centimetres before till emplacement began to dominate.
Overall, the LGM ice advance (EP II) resulted in a chain of process events. A major part of the Würmian pre-LGM stratigraphic record is missing in the Kitzbühel Terrace at Einödgraben. Hence, it is very likely that subglacial erosion during LGM is responsible for this hiatus. In contrast, in the Inn Valley (Starnberger et al., 2013; Barrett et al., 2017) and in the tributary valleys at Hopfgarten west of Kitzbühel (Reitner, 2024), deposits of the Middle Würmian (MIS 4–3) and of the early phase of LGM ice buildup are partly preserved below the LGM till.
- 1.
During EP II, the first phase of deformation resulted in the formation of diapir-like structures and tilted strata. This glaciotectonic evidence, which has not previously been reported before in the Alps, indicates non-frozen conditions within Unit A during deformation below the advancing glacier. Previous studies, for example by McCarroll and Rijsdijk (2003), have suggested that such structures are typical in the proglacial and glaciolacustrine environments. Given the facies of the deformed deposits (Unit A), the latter can be excluded from this discussion. The most probable scenario is that at the macroscale deformation occurred in a subglacial setting close to the advancing glacier margin.
- 3.
Microsedimentology indicates that the style of deformation changed from proglacial/submarginal to subglacial, during which substantial erosion took place at the base of the glacier, evidenced by the truncated diapir (EP II in Unit A). By disturbing the underlying sediment, evidence can be observed of the depth and extent of deformation processes that, in turn, produced a form of proto-till (diamicton). This is an important finding, revealing the effects of re-transport on already-emplaced subglacial sediment occurring initially but only sporadically during very localised subglacial till deposition.
- 3.
Where the LGM till (T1), at the upper contact, is superseded by glaciolacustrine sediments, the contact is gradational such that a subfacies diamicton (or till) was disrupted and deformed but retained many characteristics typical of subglacial till.
- 4.
The glaciolacustrine deposits (Unit B) are the result of the EP III event (Fig. 2c), which occurred in the early Lateglacial phase of ice decay (Reitner, 2007) after the more than 1.2 km thick glacier collapsed and melted in this sector of the Jochberger Ache Valley. Slump folds at the macroscale, not discussed here but occurring in other neighbouring locations, indicate a highly dynamic infill in a short-lived ice marginal to proglacial lake.
Finally, the last remaining part of an actively flowing Achen glacier revealed a small glacier oscillation overriding the previously deposited lake basin fill. This last glacier activity was assumed to be characterised by deformable bed conditions (EP IV).
These new findings show that, within an Alpine geological perspective, subglacial conditions during the LGM and early Lateglacial at Einödgraben are in general accordance with those from the Wildschönau Valley (Kitzbühel Alps) and Wilder Kaiser mountains (Menzies and Reitner, 2016, 2019; see Fig. 1b for location). It must be emphasised that the Einödgraben site, together with the Ainet site south of the Hohen Tauern and, thus, of the Alpine main divide (Reitner and Menzies, 2020; for location see Fig. 1a), likely represents a complete sequence from the peak of LGM glaciation to the following deglaciation with two macro- and microsedimentologically described subglacial tills.
In a final summary the research from Einödgraben can be subdivided into (1) paleoglaciological aspects, (2) till depositional mechanics and (3) specific till depositional mechanisms operating in mountainous terrain as opposed to lowland glacial systems.
- 1.
From a paleoglaciological point of view, the evidence for the detailed and spatially close research on the microsedimentology of till at Einödgraben reflects our increasing grasp and understanding of till microsedimentology in mountainous terrains. It can be clearly demonstrated that very close and rapid changes in till rheology, and thus stress fields, occur within these tills.
- 2.
It also reveals that the definition of a till needs to be broadened to permit these sediments that, due to their proximity to active ice interfaces, do appear to be till-like in so many aspects of their sedimentology. This later discovery suggests that many till-like sediments need to be revisited and stratigraphies, if not corrected, at least reassessed.
- 3.
Finally, glacial depositional mechanics in mountainous terrains cannot be equated with our previous understanding of these processes based solely upon our understanding of glacial deposition in the long low-gradient terrains of the prairies of North America or the vast expanse of the lowlands of the northern European plain.
It is evident that a new model of glacial deposition and till formation is required for the steeply incised and often constrained ice mass flow conditions that occur in mountainous terrains. Likewise, differing styles of depositional and sedimentological conditions develop under these subglacial environments that require much further research.
Additional data are available from the authors upon reasonable request.
JMR and JM conceptualised this study. Sampling was carried out in 2007. Macrosedimentological analyses were done by JMR, while JM carried out microsedimentological analyses. Both authors contributed equally to this paper.
The contact author has declared that neither of the authors has any competing interests.
Publisher's note: Copernicus Publications remains neutral with regard to jurisdictional claims made in the text, published maps, institutional affiliations, or any other geographical representation in this paper. While Copernicus Publications makes every effort to include appropriate place names, the final responsibility lies with the authors.
This article is part of the special issue “Quaternary research in times of change – inspired by INQUA Roma 2023”. It is a result of the INQUA conference, Rome, Italy, 14–20 July 2023.
The authors wish to thank Marty Ouellette for the thin-section production and Mike Lozon for his superb draftsmanship. Jürgen M. Reitner is grateful to the participants of the AGAQ 2003 field meeting and the INQUA-CECLAP 2013 post-workshop excursion for their contribution to fruitful discussions on the sedimentology and stratigraphy of Einödgraben. Jürgen M. Reitner thanks Christoph Iglseder for sharing his expertise on clast lithology and Emrys Phillips for a fruitful discussion on macroscale deformation. John Menzies is most grateful to the Geologische Bundesanstalt (Geological Survey of Austria) and the Commission for Quaternary Research of the Austrian Academy of Science for supporting fieldwork. Finally, our sincere thanks goes to Marius Buechi and Jean François Ghienne for their superb reviewing skills and profound advice on an earlier version of this paper.
This paper was edited by Gilles Rixhon and reviewed by Marius Buechi and Jean-Francois Ghienne.
Alley, R. B.: Deforming-bed origin for southern Laurentide till sheets?, J. Glaciol., 37, 67–76, https://doi.org/10.3189/S0022143000042817, 1991.
Barrett, S., Starnberger, R., Tjallingii, R., Brauer, A., and Spötl, C.: The sedimentary history of the inner-alpine Inn Valley, Austria: extending the Baumkirchen type section further back in time with new drilling, J. Quaternary Sci., 32, 63–79, https://doi.org/10.1002/jqs.2924, 2017.
Boulton, G. S.: Subglacial processes and the development of glacial bedforms, in: Research in Glacial, Glacio-fluvial and Glacio-lacustrine Systems, 6th Guelph Symposium on Geomorphology, University of Guelph, Canada, June 1982, edited by: Davidson-Arnott, R., Nickling, W., and Fahey, B. D., Geo Books Publishing, Norwich, 1–31, 1982.
Buechi, M. W., Frank, S. M., Graf, H. R., Menzies, J., and Anselmetti, F. S.: Subglacial emplacement of tills and meltwater deposits at the base of overdeepened bedrock troughs, Sedimentology, 64, 658–685, https://doi.org/10.1111/sed.12319, 2017.
Buechi, M. W., Graf, H. R., Haldimann, P., Lowick, S. E., and Anselmetti, F. S.: Multiple Quaternary erosion and infill cycles in overdeepened basins of the northern Alpine foreland, Swiss J. Geosci., 111, 133–167, https://doi.org/10.1007/s00015-017-0289-9, 2018.
Chaline, J. and Jerz, H.: Arbeitsergebnisse der Subkommission für Europäische Quartärstratigraphie, Stratotypen des Würm-Glazials – Eiszeitalter und Gegenwart, 35, 185–206, 1984.
Ehlers, J., Gibbard, P. L., and Hughes, P. D. (Eds.): Quaternary glaciations – extent and chronology: a closer look, in: Developments in Quaternary Science, Elsevier, 15, 108 pp., 2011.
Evans, D. J.: Till: A glacial process sedimentology, John Wiley & Sons, 400 pp., ISBN 978-1-118-65259-6, 2017.
Evans, D. J. A., Phillips, E. R., Hiemstra, J. F., and Auton, C. A.: Subglacial till: Formation, sedimentary characteristics and classification. Earth-Sci. Rev., 78, 115–176, https://doi.org/10.1016/j.earscirev.2006.04.001, 2006.
Heinisch, H., Pestal, G., Reitner, J., and Stingl V.: Geologische Karte der Republik Österreich 1:50.000, ÖK 122, Kitzbühel, Geologische Bundesanstalt, Vienna, 2003.
Heinisch, H., Pestal, G., and Reitner, J. M.: Geologische Karte der Republik Österreich 1:50 000, Erläuterungen zu Blatt 122 Kitzbühel, Geologische Bundesanstalt, Vienna, 301 pp., ISBN-13: 978-3-85316-079-4, 2015.
Iverson, R. M.: The debris-flow rheology myth, in: Debris-flow hazards mitigation: mechanics, prediction, and assessment, edited by: Rickenmann, D. and Chen, C., US Govt. publishers, 303–314, https://pubs.er.usgs.gov/publication/70024614 (last access: 1 July 2023), 2003.
Ivy-Ochs, S., Monegato, G., and Reitner, J. M.: Chapter 39 – The Alps: glacial landforms prior to the Last Glacial Maximum, in: European Glacial Landscapes – Maximum Extent of Glaciations, edited by: Palacios, D., Hughes, P. D., García-Ruiz, J. M., and Andrés, N., Elsevier, 283–294, https://doi.org/10.1016/B978-0-12-823498-3.00008-X, 2022a
Ivy-Ochs, S., Monegato, G., and Reitner, J. M.: Chapter 58 – The Alps: glacial landforms from the Last Glacial Maximum, in: European Glacial Landscapes – Maximum Extent of Glaciations, edited by: Palacios, D., Hughes, P. D., García-Ruiz, J. M., and Andrés, N., Elsevier, 449–460, https://doi.org/10.1016/B978-0-12-823498-3.00030-3 , 2022b.
Kamleitner, S., Ivy-Ochs, S., Monegato, G., Gianotti, F., Akçar, N., Vockenhuber, C., Christl, M., and Synal, H.-A.: The Ticino-Toce glacier system (Swiss-Italian Alps) in the framework of the Alpine Last Glacial Maximum, Quaternary Sci. Rev., 279, 107400, https://doi.org/10.1016/j.pgeola.2018.11.001, 2022.
Keller, B.: Lithofazies-Codes fur die Klassifikation von Lockergesteinen, Mitteilungen der Schweizerischen Gesellschaft für Boden- und Felsmechanik 132, 5–12, 1996.
McCarroll, D. and Rijsdijk, K. F.: Deformation styles as a key for interpreting glacial depositional environments, J. Quaternary. Sci., 18, 473–489, https://doi.org/10.1002/jqs.780, 2003.
Menzies, J.: Subglacial hydraulic conditions and their possible impact upon subglacial bed formation, Sediment. Geol., 62, 125–150, https://doi.org/10.1016/0037-0738(89)90112-7, 1989.
Menzies, J.: Tills and tillites, in: The Encyclopaedia of Sediments and Sedimentary Rocks, edited by: Middleton, G.V., Kluwer Academic Publishers, 744–747, ISBN 1-4020-0872-4, 2003.
Menzies, J.: Differentiation of diamictons (glacigenic – non-glacigenic) using microstructure abundancies and types, P. Geologist Assoc., 133, 603–615, https://doi.org/10.1016/j.pgeola.2022.07.006, 2022.
Menzies, J.: A Micromorphological Perspective on the Neoproterozoic Smalfjord and Mortensnes Formation Diamictites – Varangerfjord, Norway. Can. J. Earth Sci., 60, 696–712, https://doi.org/10.1139/cjes-2022-0019, 2023.
Menzies, J. and Reitner, J. M.: Microsedimentology of ice stream tills from the Eastern Alps, Austria – a new perspective on till microstructures, Boreas, 45, 804–827, https://doi.org/10.1111/bor.12189, 2016.
Menzies, J. and Reitner, J. M.: Microstructures, subglacial till deposition, and shear band development revealing up-section changes in shear – A study from Weissbach, Austria, P. Geologist Assoc., 130, 196–209, https://doi.org/10.1016/j.pgeola.2018.11.001, 2019.
Menzies, J., van der Meer, J. J. M., and Shilts, W. W.: Chapter 5 – Subglacial Processes and Sediments, in: Past Glacial Environments, 2nd edn., edited by: Menzies, J. and van der Meer, J. J. M., Elsevier, 105–158, 2018.
Menzies, J., Paulen, R. C., Reitner, J. M., Gao, C., Hodder, T., and Ross, M.: Subglacial tills: a process model based on microsedimentological clues, J. Sediment. Res., 93, 705–728, https://doi.org/10.2110/jsr.2022.017, 2023.
Patzelt, G.: Unterinntal–Zillertal–Pinzgau–Kitzbühel. Spät- und Postglaziale Landschaftsentwicklung, in: Tirol – Ein geographischer Exkursionsführer, edited by: Fliri, F. and Leidlmair, A., Innsbrucker Geographische Studien, Innsbruck, 2, 309–329, 1975.
Phillips, E. and Hiemstra, J. F.: Micromorphology – the description and interpretation of glacial sediments in thin section, in: Glacial and Periglacial Materials – a field-based sedimentology and engineering geology workshop, edited by: Evans, D. J. A., North Wales, Quaternary Reseach Association, London, 117–127, 2022
Phillips, E., Merritt, J., Auton, C., and Golledge, N.: Microstructures developed in subglacially and proglacially deformed sediments: faults, folds and fabrics, and the influence of water on the style of deformation, Quaternary Sci. Rev., 26, 1499–1528, https://doi.org/10.1016/j.quascirev.2007.03.007, 2007.
Phillips, E., van der Meer, J. J. M., and Ferguson, A.: A new “microstructural mapping” methodology for the identification, analysis and interpretation of polyphasedeformation within subglacial sediments, Quaternary Sci. Rev., 30, 2570–2596, https://doi.org/10.1016/j.quascirev.2011.04.024, 2011.
Reitner, J. M.: Quartärgeologie und Landschafts-entwicklung im Raum Kitzbühel – St. Johann i.T. – Hopfgarten (Nordtirol) vom Riss bis in das Würm-Spätglazial (MIS 6-2), PhD thesis, University of Vienna, 190 pp., https://doi.org/10.13140/RG.2.1.2505.6405, 2005.
Reitner, J. M.: Glacial dynamics at the beginning of Termination I in the Eastern Alps and their stratigraphic implications, Quatern. Int., 164–165, 64–84, https://doi.org/10.1016/j.quaint.2006.12.016, 2007.
Reitner, J. M.: The Imprint of Quaternary Processes on the Austrian Landscape, in: Landscapes and Landforms of Austria, edited by: Embleton-Hamann, C., Springer International Publishing, Cham, 47–72, https://doi.org/10.1007/978-3-030-92815-5_3, 2022.
Reitner, J. M.: Bericht 2016–2020 über geologische Aufnahmen im Quartär des Einzugsgebietes der Brixentaler Ache und im Spertental auf den Blättern 121 Neukirchen am Großvenediger und NL 33-01-13 Kufstein, Jahrbuch der Geologischen Bundesanstalt, GeoSphere Austria, Vienna, 162/1–4, 183–202, 2024.
Reitner, J. M. and Draxler, I.: Die klimatisch-fazielle Entwicklung vor dem Würm-Maximum im Raum Kitzbühel – St. Johann – Hopfgarten (Nordtirol/Österreich), Terra Nostra, 6, 298–304, 2002.
Reitner, J. M. and Menzies, J.: Microsedimentology of tills near Ainet, Austria – were palaeo-ice streams in the European Alps underlain by soft deforming bed zones?, Austrian J. Earth Sc., 113, 71–86, https://doi.org/10.17738/ajes.2020.0005, 2020.
Reitner, J. M., Gruber, W., Römer, A., and Morawetz, R.: Alpine overdeepenings and paleo-ice flow changes: an integrated geophysical-sedimentological case study from Tyrol (Austria), Swiss J. Geosci., 103, 385–405, https://doi.org/10.1007/s00015-010-0046-9, 2010.
Reitner, J. M., Ivy-Ochs, S., Drescher-Schneider, R., Hajdas, I., and Linner, M.: Reconsidering the current stratigraphy of the Alpine Lateglacial: Implications of the sedimentary and morphological record of the Lienz area (Tyrol/Austria), E&G Quaternary Sci. J., 65, 113–144, https://doi.org/10.3285/eg.65.2.02, 2016.
Rice, J. M., Paulen, R., Menzies, J., McClenaghan, M., and Oviatt, N.: Glacial stratigraphy of the Pine Point Pb-Zn mine site, Northwest Territories, Geological Survey of Canada, https://doi.org/10.4095/292184, 2013.
Rice, J. M., Paulen, R. C., Menzies, J., and McClenaghan, M. B.: Micromorphological descriptions of till from pit K-62, Pine Point mining district, Northwest Territories; Geological Survey of Canada, Open File 7526, 30 pp., https://doi.org/10.4095/293478, 2014.
Schuster, R., Daurer, A., Krenmayr, H. G., Linner, M., Mandl, G. W., Pestal, G., and Reitner, J. M.: Rocky Austria, Geology of Austria – brief and colouful, Geological Survey of Austria, Vienna, 80 pp., ISBN-13: 978-3-85316-078-7, 2014.
Seguinot, J., Ivy-Ochs, S., Jouvet, G., Huss, M., Funk, M., and Preusser, F.: Modelling last glacial cycle ice dynamics in the Alps, The Cryosphere, 12, 3265–3285, https://doi.org/10.5194/tc-12-3265-2018, 2018.
Starnberger, R., Drescher-Schneider, R., Reitner, J. M., Rodnight, H., Reimer, P. J., and Spötl, C.: Late Pleistocene climate change and landscape dynamics in the Eastern Alps: the inner-alpine Unterangerberg record (Austria), Quaternary Sci. Rev., 68, 17–42, https://doi.org/10.1016/j.quascirev.2013.02.008, 2013.
Swift, D. A., Nienow, P. W., Spedding, N., and Hoey, T. B.: Geomorphic implications of subglacial drainage configuration: rates of basal sediment evacuation controlled by seasonal drainage system evolution, Sediment. Geol., 149, 5–19, https://doi.org/10.1016/S0037-0738(01)00241-X, 2002.
van der Meer, J. J. M.: Microscopic evidence of subglacial deformation, Quaternary Sci. Rev., 12, 553–587, https://doi.org/10.1016/0277-3791(93)90069-X, 1993.
van der Meer, J. J. M.: Micromorphology, in: Past Glacial Environments – sediments, forms and techniques, edited by: Menzies, J., Butterworth-Heineman, Oxford, 335–356, ISBN 0750623527, 1996.
van der Meer, J. J. M., Menzies, J., and Rose, J.: Subglacial till: the deforming glacier bed, Quaternary Sci. Rev., 22, 1659–1685, https://doi.org/10.1016/S0277-3791(03)00141-0, 2003.
van Husen D.: Die Ostalpen in den Eiszeiten, Veröffentlichungen der Geologischen Bundesanstalt, Vienna, 2, 1–24, 1987.
van Husen, D. and Reitner, J. M.: Quaternary System, in: The lithostratigraphic units of Austria: Cenozoic Era(them), edited by: Piller, W. E., Abhandlungen der Geologische Bundesanstalt, Vienna, 76, 240–267, 2022.
Waltl, R.: The “Einödgraben“ near Aurach, Kitzbühel, in: Quaternary Field Trips in Central Europe, INQUA, XIV Intern. Congress, 3–10 August 1995, Berlin, Germany, edited by: Schirmer, W., Verlag Dr. Friedrich Pfeil, Munich, Vol. 1, 398–399, 1995.
Zoet, L. K., Iverson, N. R., Andrews, L., and Helanow, C.: Transient evolution of basal drag during glacier slip, J. Glaciol., 68, 741–750, https://doi.org/10.1017/jog.2021.131, 2021.
Zoet, L., Hansen, D., Morgan-Witts, N., Menzies, J., Sobol, P., and Lord, N.: An experimental baseline for ice-till strain indicators, Can. J. Earth Sci., 60, 537–549, https://doi.org/10.1139/cjes-2022-0074, 2023.
- How to cite
- Abstract
- Kurzfassung
- Introduction
- Geological and paleoglaciological setting
- Methodology
- Macroscale description and interpretation
- Microsedimentology
- What is a till?
- Synthesis of macro- and microsedimentological evidence and conclusions
- Data availability
- Author contributions
- Competing interests
- Disclaimer
- Special issue statement
- Acknowledgements
- Review statement
- References
- How to cite
- Abstract
- Kurzfassung
- Introduction
- Geological and paleoglaciological setting
- Methodology
- Macroscale description and interpretation
- Microsedimentology
- What is a till?
- Synthesis of macro- and microsedimentological evidence and conclusions
- Data availability
- Author contributions
- Competing interests
- Disclaimer
- Special issue statement
- Acknowledgements
- Review statement
- References