the Creative Commons Attribution 4.0 License.
the Creative Commons Attribution 4.0 License.
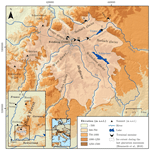
Revisiting Late Pleistocene glacier dynamics north-west of the Feldberg, southern Black Forest, Germany
Felix Martin Hofmann
Florian Rauscher
William McCreary
Jan-Paul Bischoff
Frank Preusser
The southern Black Forest was temporarily covered by a ∼1000 km2 large ice cap during the Late Pleistocene. However, during the last glaciation maximum in the Alps the atmospheric circulation over Europe was presumably characterised by the advection of humid air masses from the Mediterranean Sea. As a consequence, the ice cap of the Black Forest was likely in a leeward position due to its location north of the Alps. This raises the question of whether it reached its last maximum extent simultaneously with the glaciers in the Alps. As modern dating techniques have hitherto not been applied to the southern Black Forest, the timing of the last local glaciation maximum remains poorly constrained. As a first step towards an independent regional glacier chronology, we present a critical re-examination of glacial landforms in the area north-west of the highest summit of the Black Forest (Feldberg, 1493 m a.s.l.). It relies on both the analysis of remote sensing data and field mapping. The review of previous studies highlights important disagreements regarding the location of ice-marginal positions and their correlation. In addition, our findings challenge earlier studies on the glaciation of the Black Forest: some previously described ice-marginal positions could not be confirmed, whereas some of the newly identified moraines are described for the first time. This highlights the need for detailed geomorphological investigations prior to the application of geochronological methods. A multi-ridged series of terminal moraines in one of the studied valleys, Sankt Wilhelmer Tal, is proposed as the main target for future dating. Due to discrepancies with earlier studies, future efforts should reinvestigate other key areas related to the last glaciation of the southern Black Forest.
Der südliche Schwarzwald war während des Spätpleistozäns zeitweise von einer etwa 1000 km2 großen Eiskappe bedeckt. Während des letzten glazialen Maximums in den Alpen war die atmosphärische Zirkulation über Europa jedoch wahrscheinlich durch eine Zufuhr feuchtebringender Luftmassen aus dem Mittelmeergebiet geprägt. Demnach lag die Eiskappe des Schwarzwaldes vermutlich im Lee der Alpen. Es stellt sich somit die Frage, ob die Ausdehnung der Eiskappe des Schwarzwaldes synchron mit den Alpengletschern ihr letztes Maximum erreichte. Da moderne Methoden der Altersbestimmung im Südschwarzwald bisher noch nicht eingesetzt worden sind, ist der Zeitpunkt des letzten lokalen glazialen Maximums derzeit schlecht erfasst. Als erster Schritt zu einer unabhängigen regionalen Gletscherchronologie präsentieren wir eine kritische Bewertung bisheriger Studien zu Spuren der Vergletscherung nordöstlich der höchsten Erhebung des Schwarzwaldes (Feldberg, 1493 m ü. NHN). Diese beruht sowohl auf der Auswertung von Fernerkundungsdaten als auch auf Feldkartierungen. Die Betrachtung der Ergebnisse bisheriger Studien unterstreicht teilweise deutliche Unterschiede in Bezug auf die Lage von Eisrändern und deren Korrelation. Zusätzlich zeigt diese Arbeit Diskrepanzen zu früheren Untersuchungen auf: einige der beschriebenen Eisrandlagen konnten nicht bestätigt werden, während in dieser Arbeit andere Endmoränen zum ersten Mal erwähnt werden. Dies zeigt die Notwendigkeit von sorgfältigen geomorphologischen Untersuchungen vor der Anwendung geochronologischer Methoden. Eine Serie mehrerer Endmoränenwälle in einem untersuchten Tal, dem Sankt Wilhelmer Tal, wird als Zielobjekt zukünftiger Datierungen vorgeschlagen. Aufgrund der offensichtlichen Diskrepanzen zu früheren Studien sollten zukünftig weitere Schlüsselgebiete der letzten Schwarzwaldvergletscherung geomorphologisch neu untersucht werden.
- Article
(28803 KB) - Full-text XML
-
Supplement
(11964 KB) - BibTeX
- EndNote
Ice caps and glaciers are considered valuable archives for palaeoclimatic reconstructions (Kerschner and Ivy-Ochs, 2008; Stokes et al., 2015; Mackintosh et al., 2017) if factors other than climate, such as topography, do not significantly influence their mass balance (Barr and Lovell, 2014). Glacier-based palaeoclimatic reconstructions require landforms indicative of former glacier extents to be identified and dated. Structure-from-motion-based digital surface models or high-resolution light detecting and ranging data are nowadays commonly used for their identification (Chandler et al., 2018). Starting with the pioneering work of Ivy-Ochs et al. (1996) in the Alps, terrestrial cosmogenic nuclide (TCN) dating of boulders on terminal moraines has become a frequently used tool for the establishment of glacier chronologies (see Balco, 2020). In addition, new approaches in the field of optically stimulated luminescence (OSL) dating have been developed. One of these techniques is the reconstruction of luminescence depth profiles from boulders buried inside glacial deposits. This approach provides not only the age of an ice-marginal position but also insights into the transport history of the dated boulders (Rades et al., 2018). Chronological information on past glacier extents then serves as a base for palaeoglacier reconstruction (Pellitero et al., 2016), whereby geographical information system (GIS) approaches are commonly used (Pearce et al., 2017). The subsequent determination of the equilibrium line altitude (ELA) reveals information on palaeotemperature and/or precipitation (Moran et al., 2016; Hofmann et al., 2019; Protin et al., 2019).
Due to its lower elevation when compared with the southern Black Forest, the last glaciation of the northern Black Forest was restricted to small valley glaciers and cirque glaciers in the area around Hornisgrinde (Fig. 1; Hemmerle et al., 2016, and references therein). The southern Black Forest was covered by a 1000 km2 large ice cap during the Late Pleistocene (Fig. 1; Metz and Saurer, 2012). It was characterised by radial ice flow from its centre on top of the summit of the Feldberg (1493 m a.s.l.), and several passes in its surroundings were overridden by outlet glaciers. Steinmann (1902) already recognised that the subsequent deglaciation was interrupted by periods of stationary glaciers. The current subdivision of the deglaciation comprises, from the oldest to the youngest, the Hölzebruck, Titisee, Bärental, Waldhof, Feldsee and Seebuck-Nischenkar stades (Metz and Saurer, 2012; Hemmerle et al., 2016). The few limited conclusions about their age were drawn by analogy with data from sediment cores from mires and lakes inside terminal moraines. These approximate age estimates are based on the apparent presence of the Laacher See Tephra (deposited at ca. 12.9 ka; van den Bogaard and Schmincke, 1985) and on the succession of lithostratigraphic units in the cores (Lang et al., 1984; Merkt, 1985; Lang, 2005). None of the modern geochronological methods mentioned above have hitherto been applied to the southern Black Forest.
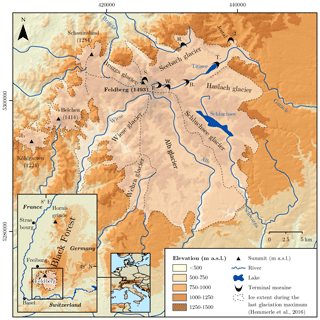
Figure 1Location of southern Black Forest, last glaciation maximum ice extent and prominent terminal moraines used for the subdivision of the last glaciation. S: Seebuck-Nischenkar stade; F: Feldsee stade; W: Waldhof stade; B: Bärental stade; T: Titisee stade; H: Hölzlebruck stade; J: Jostal stade. The shaded relief is based on data from the Shuttle Radar Topography Mission (SRTM; version 4) available at http://srtm.csi.cgiar.org/srtmdata (last access: 9 July 2019). Coordinate systems: ETRS 1989 UTM Zone 32N and WGS 1984.
From a palaeoclimatological perspective, there is an urgent need to determine the timing of the last local glaciation maximum. Since the study of Florineth and Schlüchter (2000), it has been repeatedly suggested that the atmospheric circulation over Europe was characterised by the advection of humid air masses from the Mediterranean Sea during the last glaciation maximum in the Alps (at ca. 26–24 ka; Kuhlemann et al., 2008; Luetscher et al., 2015; Monegato et al., 2017). If this scenario is correct, the ice cap of the Black Forest would have been in a leeward position with regard to the Alps. Only a reduced amount of precipitation would thus have been available for ice build-up. This is also consistent with data from the Bergsee lacustrine record (southernmost Black Forest) indicating cold and arid conditions in the 30–23.5 ka period and a subsequent shift towards wetter conditions (Duprat-Oualid et al., 2017, 2020). These lines of evidence raise the question of whether the last glaciation maximum in the Black Forest was out of phase with the Alps. The establishment of an independent regional glacier chronology would allow for testing the hypothesis about the prevailing atmospheric circulation over Europe.
The establishment of such a chronological framework first requires detailed geomorphological investigations. This step is important, as careful geomorphological analyses are often overlooked when establishing glacier chronologies (Winkler, 2018). As high-resolution remote sensing data have hitherto not been used in this context, glacial landforms at key sites in the southern Black Forest were mapped by utilising these data and performing geomorphological field mapping. Our study enables former studies on the glaciation to be critically evaluated and suitable sites for terminal moraine dating to be selected. As a reinvestigation of all glacial landforms in the southern Black Forest would be too extensive to be presented in one paper, we focus hereinafter on glacier extent and dynamics in the area north-west of the Feldberg. The geomorphological re-evaluation of glacial landforms in other parts of the southern Black Forest is intended to be published separately. Since the subdivision of the deglaciation mainly relies on glacial features in Seebachtal north-west of the Feldberg, this region is first reviewed in detail. The results of previous studies for three different areas (Schauinsland area, Sankt Wilhelmer Tal and Zastler Tal) are subsequently critically discussed in the light of our investigations. We do not comment on the correlation of terminal moraines in our study area with previously defined glacial stades, as such an assessment would require geochronological data.
The Black Forest (48∘ N, 8∘ E) is situated in SW Germany close to the borders to France and Switzerland (Fig. 1). Its highest summit, the Feldberg (1493 m a.s.l.), is situated south-east of the city of Freiburg. The former ice cap of the southern Black Forest is commonly subdivided into the Brugga, Seebach, Haslach, Schluchsee, Alb, Wehra and Wiese palaeoglaciers (Fig. 1; Hemmerle et al., 2016). With a length of about 25 km and a thickness up to 440 m during the last glaciation maximum (Sawatzki, 1992), the Alb palaeoglacier was the longest and thickest of these glaciers (Hemmerle et al., 2016). The summits of the Schauinsland (1284 m a.s.l.), the Belchen (1414 m a.s.l.) and the Köhlgarten (1224 m a.s.l.) were probably covered by distinct ice caps that were linked to the main ice cap extending from the Feldberg (Fig. 1; Giermann, 1964; Rahm, 1987; Hemmerle et al., 2016).
Our study area comprises Bruggatal, Zastler Tal, Sankt Wilhelmer Tal and the Schauinsland area (Fig. 2). With the exception of the western part of the latter area, our study area belongs to the Brugga catchment, a tributary of the river Dreisam, which flows ultimately into the Rhine north-west of Freiburg. Zastler Tal and Sankt Wilhelmer Tal are neighbouring valleys north-west of the Feldberg. The upper part of the former valley is commonly referred to as Zastler Loch. At an elevation of 750 m a.s.l., a change in valley orientation occurs to the north-west. The valley meets Bruggatal at an elevation of 450 m a.s.l. Rinkendobel, Angelsbach and Stollenbach are its formerly glaciated tributary valleys. The upper part of the Sankt Wilhelmer Tal further south is in the following referred to as Napf. Wittenbach and Katzensteig are its tributary valleys. The valley meets Bruggatal at an elevation of 650 m a.s.l. The further SW Buselbachtal including its two tributary valleys (Steinwasental and Krummenbachtal) is hereinafter subsumed into the upper Brugga catchment. It is delimited to the west by a relatively smooth plateau reaching from the Schauinsland (1284 m a.s.l.) in a southerly direction towards the Haldenköpfle (1265 m a.s.l.). Kappeler Tal, a valley with a steep longitudinal profile north of Schauinsland, the upper Brugga catchment and the upper portions of all other valleys around the summit are hereinafter subsumed as the Schauinsland area (Fig. 2).
Multiple groups of terminal moraines inside the last glaciation maximum ice extent (Fig. 1) bear witness to standstills and/or glacial readvances during the deglaciation. As the terminal moraines of the Seebach palaeoglacier were the main target of early studies (e.g. Steinmann, 1902), they were defined as stratotypes for the 7-fold subdivision of the last glaciation (Fig. 1). Only the Seebuck-Nischenkar stade refers to terminal moraines outside of Seebachtal (Fig. 1; Zienert, 1973). An overview of the glacial stades and the characteristics of the glaciation as well as a tentative correlation with biozones (Schreiner, 2011) are given in Table 1.
Table 1Glacial stades in the southern Black Forest and their stratotypes (Fig. 1), characteristics of the glaciation, and a tentative correlation with biozones.
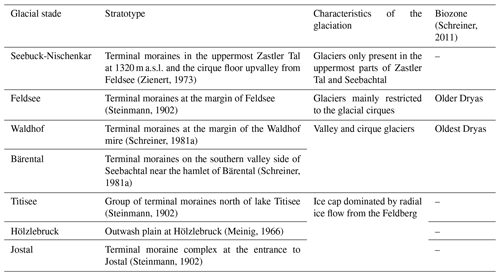
During the Late Pleistocene, the Seebach palaeoglacier advanced in a NW direction into the lower portion of the NW–SE-trending Jostal and built up a three-crested terminal moraine complex (Fig. 1). Steinmann (1902) linked this event to the last glaciation maximum and introduced the term Jostal stade. According to later sedimentological observations summarised in Meinig (1980), the base of the terminal moraine complex consists of delta sediments. The Seebach palaeoglacier overrode this delta and deposited subglacial till on top. A period of glaciofluvial sedimentation occurred thereafter, before the ice advanced again to the second-outermost terminal moraine and deposited till on top of the glaciofluvial sediments. These observations suggest that the stade was probably a multiphased period of glacial activity.
Terminal moraines were identified west of Jostal slightly inside the last glaciation maximum glacier extent. They probably formed during glacial standstills after the Jostal stade (Meinig, 1966). Schreiner (1999) grouped them and assigned the moraines to the Hölzlebruck stade named after an outwash plain south-east of the Jostal terminal moraine complex (Meinig, 1966). The most prominent glacial landform deposited during this stade is the Föhrwald moraine marked with “H.” in Fig. 1. A period of ice recession occurred thereafter.
This phase of ice decay was interrupted when the Seebach palaeoglacier readvanced and shaped the moraines north of lake Titisee (marked with “T.” in Fig. 1). The Titisee stade (Steinmann, 1902) was later subdivided into three phases (Ti1–Ti3; Schreiner, 1999). The terminal moraine of the first phase (Ti1) is found in the area north-west of the village of Titisee. A second, relatively poorly developed terminal moraine is observed at the western limit of Titisee (Ti2). The terminal moraines of the third phase (Ti3) are located directly north of the northern shore of the lake. Lang et al. (1984) found clay at the base of their sediment core from the lake and suggested that its deposition had already started during the Oldest Dryas, thereby implying that the group of terminal moraines is probably older (Fig. 3).
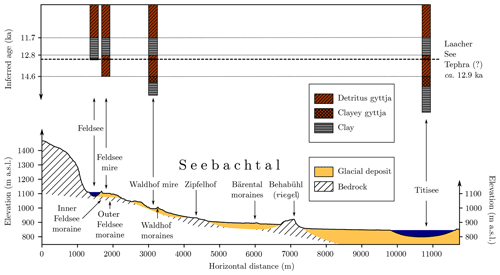
Figure 3Longitudinal profile of Seebachtal showing its lakes, terminal moraines and mires. See Lang et al. (1984) and Merkt (1985) as well as the later synthesis of Lang (2005) for a detailed description of the sediment cores. Note that ages on the ordinate axis are based on the supposed presence of Laacher See Tephra in the cores as well as on the succession of lithostratigraphic units. Radiocarbon dates were not obtained. Modified and updated from Schreiner (1981a).
Another set of five terminal moraines was identified further upvalley in Seebachtal at an elevation of 920 m a.s.l. near the hamlet of Bärental (Figs. 1 and 3). Due to their location well upvalley of the group of terminal moraines discussed before (Fig. 1), they were defined as a type site of the Bärental stade (Schreiner, 1981a). The ridges further upvalley near Zipfelhof (Fig. 3) were classified as terminal moraines and attributed to a distinct glacial stade (Zipfelhof stade; Schrepfer, 1925). Later field observations revealed that they are hilltops made up of bedrock partly covered by till (Schreiner, 1981a), and the Zipfelhof stade has thus been abandoned (Liehl, 1982; Leser and Metz, 1988; Schreiner, 1999).
Two terminal moraines further upvalley at the margin of the Waldhof mire at an elevation of around 1000 m a.s.l. (Figs. 1 and 3) were already described by Ramsay (1862). The mire is situated in a depression on the floor of a cirque extending in a southerly direction from the main valley. The terminal moraines were probably deposited when a transfluent glacier advanced in a northerly direction into the ice-free Seebachtal. Hence, Schreiner (1981a) attributed them to a distinct glacial stade, the Waldhof stade.
Two nearby arc-shaped terminal moraines are found further upvalley at an elevation of around 1100 m a.s.l. (Figs. 1 and 3). The outer moraine is located at the eastern end of the Feldsee mire, whereas the inner moraine is situated further west directly at the eastern shore of lake Feldsee (Fig. 3; Ramsay, 1862). In his 3-fold subdivision of the last glaciation, Steinmann (1902) attributed these features to the last glacial stade during the deglaciation. The terminal moraines were later attributed to two distinct stades (Zienert, 1973; Schreiner, 1977b, 1981a, b; Metz, 1985b). The Laacher See Tephra was apparently identified in sediment cores from the mire (Fig. 3; Lang et al., 1984) and later in the lake (Fig. 3; Merkt, 1985; see Lang, 2005, for a later synthesis). Hence, it was concluded that the ages of the terminal moraines are not considerably different, and both moraines were subsequently attributed to the Feldsee stade (Leser and Metz, 1988; Lang, 2005; Metz and Saurer, 2012). Outside of Seebachtal, the glaciation of the southern Black Forest was restricted to small cirque glaciers (Liehl, 1982). The apparent presence of the Laacher See Tephra in the sediments of the lake suggests that this area had already become ice-free during the climatically favourable period between ca. 14.6 and 12.8 ka documented in lacustrine records in and around the Alps (Heiri et al., 2014, and references therein). Hence, the Feldsee stade probably occurred during this time or, alternatively, before the climatic amelioration at ca. 14.6 ka (Lang, 2005). Metz and Saurer (2012) suggested that the stade is correlated with the Gschnitz stadial in the Alps dated to 17–16 ka at the type locality (Ivy-Ochs et al., 2006; Ivy-Ochs, 2015).
The youngest glacial stade, the Seebuck-Nischenkar stade, was introduced by Zienert (1973). The formation of both the cirque floor upvalley of Feldsee and the terminal moraines in Zastler Tal at around 1320 m a.s.l. (Fig. 1) was assigned to this stade (Zienert, 1973; Schreiner, 1990). According to Zienert (1973), only the uppermost parts of Zastler Tal and Seebachtal were covered by residual glaciers at that time. Lang et al. (1984) speculated that it may have occurred during the cooling in Europe between 12.8 and 11.7 ka documented in palaeoclimatic archives in and around the Alps (Heiri et al., 2014, and references therein). After this stade, the glaciers of the southern Black Forest finally disappeared.
The comprehensive review of previous studies was performed by creating an inventory of previously published maps of glacial landforms north-west of the Feldberg (e.g. Giermann, 1964). They were scanned and subsequently georeferenced in Esri ArcMap 10.5.1. For the establishment of our new geomorphological maps at a 1:10 000 scale, a high-resolution digital terrain model (DTM) including its derivates was chosen for a GIS-based approach that offers the unique opportunity to remove and add layers on the fly (Wagner, 2018). Firstly, a shaded relief was created using the “hillshade” tool. The application of the “slope” and “aspect” tools in ArcMap enabled two supplementary raster files to be derived. For geomorphological mapping of unforested areas, the derivates of the DTM were jointly used with orthophotos obtained in 2016 and 2018 available at https://gis.lkbh.net/buergergis/synserver?project=buergergis&view=kreiskarte (last access: 11 May 2020). The morphology of suspected ice-marginal landforms was assessed with the “3D analyst” tool. As remote sensing data do not provide insights into the internal structure of landforms, it was later verified in the field if the supposed ice-marginal features consisted of sediments. Outcrops were inspected, where available. All confirmed ice-marginal landforms were subsequently marked in a printout version of the shaded relief at the 1:5000 scale. The resulting field maps were finally scanned, imported in Esri ArcMap 10.5.1 and digitised.
Due to the presence of well-preserved terminal moraines and the lack of vegetation, an additional drone survey was conducted in the Katzensteig valley to derive a more precise digital surface model (DSM) based on structure-from-motion photogrammetry (Westoby et al., 2012). Photos obtained from a quadcopter with a 12 MP camera were processed in Agisoft Metashape using the highest default settings allowed for the establishment of a DSM with a 3.7 cm pixel size and an orthophoto with an x- and y-cell size of 5.4 and 3.7 cm, respectively. A detailed description of the methodology is given in the Supplement. As discussed for the DTM, three supplementary raster files were derived from the DSM in ArcMap using the hillshade, slope and aspect tools.
Geomorphological mapping was performed at a scale of 1:5000 in ArcMap 10.5.1 using the ArcGIS version of the geomorphological mapping system of the University of Lausanne (Schoeneich, 1993). The mapping system is based on the principle that surfaces mainly affected by erosion or denudation are not highlighted in colours. The colours of the depositional landforms stand for distinct process categories. For example, glacial landforms are highlighted in violet. A section of the detailed geomorphological map of Sankt Wilhelmer Tal is given in Fig. 10. All other maps are found in the Supplement. Morphostratigraphies were established by first grouping terminal moraines apparently belonging to the same ice-marginal position. The following criteria were employed for grouping terminal moraines: the topographic position on the valley sides, the geometry of the ends of the terminal moraines and the size. All frontal positions defined by terminal moraines, or those inferred from a group of terminal moraines, were then numbered. The numbering of the terminal moraines starts from the cirque and thus enables older ice-marginal positions further down the valley to be added in the future if appropriate.
Radiocarbon ages from a mire inside a terminal moraine complex in our study area (Friedmann, 1998/1999) were recalibrated using the OxCal program (version 4.3; Bronk Ramsey, 2009) based on IntCal13 (Reimer et al., 2013). They are expressed by both the median of the probability distribution function and the 2σ interval of calibration (Telford et al., 2004).
5.1 Schauinsland area
It is generally accepted that the Brugga catchment was covered by an outlet glacier, the Brugga palaeoglacier, fed by both the ice cap of the Schauinsland and the significantly larger ice cap centred on the Feldberg (Fig. 1; Steinmann, 1896; Schrepfer, 1931; Giermann, 1964; Hüttner, 1967; Schreiner, 2011). The most recent synthesis of glacial landforms in the northern part of the Schauinsland area is given by Hüttner (1967). Glacial landforms further south are depicted on the geological map “8113 Todtnau” (Hann et al., 2011) and described in Schreiner (2011). As they are particularly abundant, terminal moraines in Sankt Wilhelmer Tal and its tributary valleys are presented in a distinct section below. A map of previously identified glacial cirques and moraines is given in Fig. 4. Prominent terminal moraines and glacial cirques are depicted in Fig. 5. Confirmed and newly mapped terminal moraines and glacial cirques are presented in Fig. 6. The complete geomorphological maps of key areas are found in the supplementary material (Fig. S1).
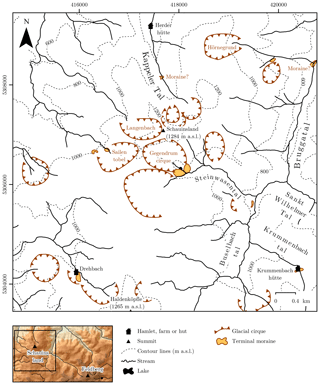
Figure 4Previously mapped glacial cirques and terminal moraines in the Schauinsland area (Giermann, 1964; Wimmenauer and Hüttner, 1968; Schreiner, 1996b; Hann et al., 2011). See Fig. 1 for the data source of the shaded relief in the inset. Coordinate system: ETRS 1989 UTM Zone 32N.
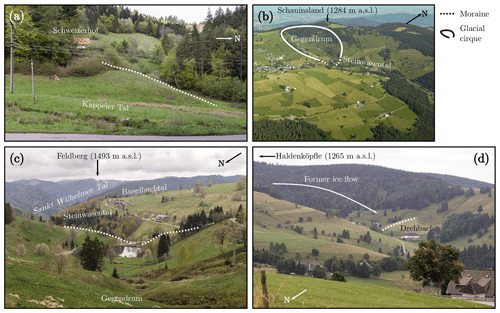
Figure 5Terminal moraines and glacial cirques in the Schauinsland area. (a) A terminal moraine in Kappeler Tal at around 600 m a.s.l. is mapped for the first time. (b) The Gegendrum cirque and the terminal moraine at its entrance viewed from an aircraft above Buselbachtal (photo: Matthias Geyer). (c) The moraines at the entrance to the Gegendrum cirque. (d) The prominent terminal moraine at Drehbach formed at the front of a small palaeoglacier originating from Haldenköpfle. See Fig. 6 for the locations from where the photos were taken.
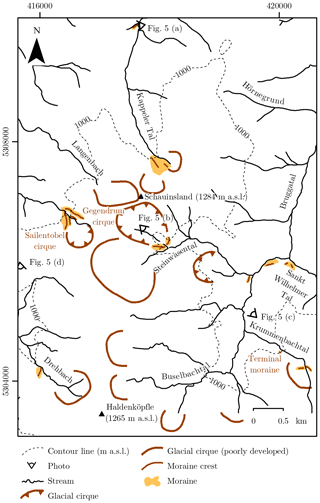
Figure 6Confirmed and newly mapped terminal moraines as well as glacial cirques in the Schauinsland area. See Fig. S2 for a complete geomorphological map of key localities in the Schauinsland area. Coordinate system: ETRS 1989 UTM Zone 32N.
5.1.1 Kappeler Tal
There is a consensus that the S–N-trending valley with a steep longitudinal profile (Fig. 2) was occupied by a small valley glacier during the last glaciation maximum (Steinmann, 1896; Schrepfer, 1931; Erb, 1948; Giermann, 1964; Hüttner, 1967). Despite its favourable orientation (Fig. 2), the valley does not feature the typical U-shape of a glacial valley. The lack of large accumulation areas in the upper part of the valley (Hüttner, 1967) is one possible explanation for the absence of a clear glacial morphology. It seems unlikely that the valley precursor was not suitable for modification into a glacial trough. In fact, the well-developed trough in the upper part of Sailentobel further south-west is situated in an area where the same bedrock lithologies occur (Schreiner, 1996b). The upper end of the valley consists of two neighbouring slightly amphitheatre-shaped niches. These are interpreted as poorly developed glacial cirques in this study. They are separated by two smooth sediment ridges extending from 960 to 1120 m a.s.l. Due to their position between the two poorly developed glacial cirques, they are interpreted as medial moraines in agreement with Hüttner (1967). A further small ridge at around 860 m a.s.l. further down the valley, on the centre of the valley floor (Fig. 4), may be either a terminal moraine or an anthropogenic dam for timber rafting (Giermann, 1959; Hüttner, 1967). Due to the unknown origin of the ridge, it was not classified as an ice-marginal position in this study (Fig. 6).
A ridge emerges from the steep western valley side further down the valley. The several-metres-thick ridge meets the valley floor with an angle of 75∘ at around 600 m a.s.l. (Fig. 5a). No outcropping bedrock was observed during field mapping, and it seems that the feature consists of sediments. It is about 200 m long, extends up to an elevation of 685 m a.s.l and is separated from the valley side by a 4 m deep and about 30 m wide depression. The separation from the valley side and the arcuate shape lead to the conclusion that the sediment ridge has to be classified as a terminal moraine. As the landform is particularly prominent (Fig. 5a), it is surprising that it was never mentioned in previous studies. Due to the absence of glacial landforms further down the valley, it reflects the outermost securely identified ice-marginal position. This study does not provide evidence for formerly suggested ice-marginal positions at around 500 m a.s.l. (Steinmann, 1896) and at around 700 m a.s.l. near Herderhütte (Fig. 4; Schrepfer, 1931).
5.1.2 Steinwasental
Two prominent, 15 m high terminal moraines consisting of rounded clasts and finer sediments are situated on both sides of the stream at the entrance to the Gegendrum cirque (Klute, 1911; Schrepfer, 1931; Giermann, 1964; Schreiner, 1996b). The presence of well-preserved terminal moraines at the entrance to the cirque is confirmed here (Figs. 5b–c and 6).
A N–S-oriented sediment ridge is located further downvalley at the entrance to Steinwasental (Fig. 4). Steinmann (1896) already classified the ridge as terminal moraine. Giermann (1959) suggested that the ridge should be regarded either as a medial moraine deposited between the palaeoglaciers from Steinwasental and Buselbachtal or as a terminal moraine built up at the margin of the palaeoglacier from Buselbachtal. Based on the geometry of the ridge, Hüttner (1967) rejected this interpretation. Instead, he proposed that it was built up during a readvance of a small palaeoglacier from a cirque north-west of the feature (not shown in Fig. 4) when the snout palaeoglaciers in Steinwasental and Buselbachtal were located well upvalley. Schreiner (2011) suggested that the terminal moraine formed during an advance of a palaeoglacier from a cirque directly west of the ridge (Fig. 4). We did not identify a cirque directly west of the terminal moraine but a slope with a fairly constant angle. Hence, we suggest that the sediment ridge is a terminal moraine deposited by a palaeoglacier from the well-developed glacial cirque further north-west (Fig. 6) mentioned by Hüttner (1967).
5.1.3 Krummenbachtal
Klute (1911) describes a terminal moraine at an elevation of 1000 m a.s.l. that is confirmed in this study (Fig. 6). An about 15 m high terminal moraine is found on the area further upvalley around Krummenbachhütte (Fig. 4; Schrepfer, 1931; Hann et al., 2011), with two ridges extending from 1085 to 1105 m a.s.l., separated by a stream. Together they form an about 270 m long, 40 m wide and 3 m high arch. These parameters confirm the terminal moraine interpretation by Schrepfer (1931) and Hann et al. (2011).
5.1.4 Buselbachtal
A terminal moraine consisting of boulders is the only glacial landform in this valley, situated about 200 m west of the entrance to Sankt Wilhelmer Tal. As the landform is partly separated from the surrounding slope and it has an asymmetric shape, the terminal moraine interpretation by Hüttner (1967) is confirmed (Fig. 6).
5.1.5 Bruggatal
A triangular feature solely consisting of boulders is found at the entrance to Sankt Wilhelmer Tal at about 650 m a.s.l. It is located on the southern part of the valley floor (Fig. 6). The surface of the landform is almost flat. As it consists of sediments, the landform was classified as a medial moraine deposited between the confluent palaeoglaciers from both valleys (Hüttner, 1967). Hüttner (1967) suggested that the matrix between the boulders was washed away by meltwater after the deposition of the medial moraine. As the boulders are partially rounded, we infer that the boulders must have been transported for at least some distance. Therefore, it is unlikely that the boulders originate from the escarpment directly uphill. We suggest that a rockslide occurred somewhere on the southern side of Sankt Wilhelmer Tal and boulders were deposited on the ice-free valley floor. The deposit was then moved to its present location by an advancing glacier. During this process the boulders were partially rounded. The triangular shape developed when the glacier front met the palaeoglacier from Buselbachtal. Hence, the landform is interpreted as a medial moraine derived from a rockslide and it might be of the same age as the terminal moraine 200 m further west described in the previous section (Fig. 6).
Schrepfer (1931) proposed a halt in the glacier recession of the Brugga palaeoglacier that occurred when the terminus was located halfway between the entrance to Hörnegrund and Sankt Wilhelmer Tal. This study does not reveal geomorphological evidence supporting this hypothesis (Fig. 6).
Steinmann (1896) described a roughly 10 m thick accumulation of boulders near the entrance to Hörnegrund, a tributary valley of Bruggatal (Fig. 2). It was interpreted as a terminal moraine (Fig. 4) and classified as the last glaciation maximum position of the Brugga palaeoglacier, confirmed by Schrepfer (1931). For the widening of a road, the slope at the entrance to the tributary valley was excavated, and the sediments in the lower portion of the outcrop were classified as moraine (till) material, whereas those in the upper part were interpreted as glaciofluvial sediments (Giermann, 1959). Giermann (1959) argued that the sediments should be considered remnants of a terminal moraine formed during the furthest advance of the palaeoglacier in Bruggatal. In contrast, Hüttner (1967) suggested that the sediments in the lower part of the outcrop should be classified as fluvial sediments from the tributary valley, interfingering with glaciofluvial sediments from the main valley. Nevertheless, Hüttner (1967) assumed that the ice margin was located somewhere near the outcrop by analogy with Zastler Tal, where the palaeoglacier possibly reached an elevation of about 500 m a.s.l. during the last glaciation maximum. It should be noted that this interpretation is not supported by geomorphological evidence (Schreiner, 2011). Although an ice-marginal position at the entrance to the tributary valley is still mentioned in the most recent syntheses on the glaciation of the southern Black Forest (Schreiner, 2011; Metz and Saurer, 2012; Hemmerle et al., 2016), this study does not provide any geomorphological evidence for an ice-marginal position at this locality. Rather, this area is occupied by a large cone-shaped feature interpreted as an alluvial fan (see Fig. S1c for further details). Hence, the terrace-shaped feature at the entrance to Sankt Wilhelmer Tal is considered the outermost evident ice-marginal position (Fig. 6). As no associated frontal position was identified, the location of the glacier terminus during the deposition of the triangular feature remains unknown.
5.1.6 Hörnegrund
The tributary valley of Bruggatal (Fig. 2) was probably occupied by a small valley glacier originating from the poorly developed glacial cirque in its uppermost part during the last glaciation maximum (Fig. 4). However, it remains uncertain whether the palaeoglacier reached the main valley or not (Schrepfer, 1931; Giermann, 1959). Schrepfer (1931) observed a ridge at an elevation of 640 m a.s.l. (Fig. 4) and suggested that this landform is either a terminal or a medial moraine that developed between two small confluent glacier systems from the upper part of the valley. According to later observations, the ridge consists of poorly rounded clasts in a matrix of loamy sand (Hüttner, 1967). This study reveals that the ridge is composed of bedrock, and, thus, the moraine interpretation has to be rejected. Hence, there is no evidence for an ice-marginal position in the entire valley (Fig. 6).
5.1.7 Drehbach
A 15 m high prominent ridge in the SE–NW-trending valley north-west of Haldenköpfle (Fig. 5d) was first described by Steinmann (in Klute, 1911) and later mapped by the State Geological Survey of Baden-Württemberg (LGRB; Schreiner, 1996b; Hann et al., 2011). It is interpreted as a terminal moraine of the palaeoglacier originating from the cirque in the uppermost part of the valley (Fig. 4; Schrepfer, 1931; Giermann, 1964; Schreiner, 2011). This study reveals the absence of indications of a mass movement from an area further upslope as well as the lack of channels further upslope. Hence, a gravitational or fluvial formation of the feature is excluded and the classification of the ridge as a terminal moraine (Schrepfer, 1931; Giermann, 1964; Schreiner, 2011) is approved (Fig. 6).
5.1.8 Sailentobel
The upper part of the SE–NW-trending valley was covered by a small glacier (Giermann, 1964; Wimmenauer and Hüttner, 1968). Its uppermost part is a NW-oriented glacial cirque characterised by a steep headwall. Nowadays a mire occupies the almost horizontal cirque floor at an elevation of about 1000 m a.s.l. The mire with a diameter of about 150 m is limited by a terminal moraine (Fig. 4). The terminal moraine is subdivided into two parts by a stream (Schrepfer, 1931; Giermann, 1959; Wimmenauer and Hüttner, 1968). The western terminal moraine partly covers roches moutonnées (Wimmenauer and Hüttner, 1968).
In addition to the previously mentioned terminal moraines, two ridges further downvalley are identified in this study. The sediment ridges meet at 975 m a.s.l. and are separated by a stream. The western ridge extends over 270 m and is 100 m wide and up to 20 m high. The ridge in the NE part of the cirque is described as a 370 m long and 50 wide flattening of the slope. As the sediment ridges resemble the previously mentioned terminal moraines at the margin of the mire, they were mapped as terminal moraines. The outer western moraine was mistakenly classified as a roche moutonnée by Giermann (1964) as well as by Wimmenauer and Hüttner (1968). No geomorphological evidence was found for an ice-marginal position further down the valley (Fig. 6).
5.1.9 Langenbach
In the poorly developed cirque north-east of Sailentobel (Fig. 2), Schrepfer (1931) observed two recessional moraines at an elevation of about 1020 m a.s.l. (not shown in Fig. 4 due to their unknown location). We did not observe any clear glacial landforms in the valley with a steep longitudinal profile (Fig. 6). Due to the location of the valley head at a higher elevation than that of Sailentobel, it is surprising that the upper part of the valley only meets the criteria for a poorly developed cirque. This pattern is probably explained by the considerably steeper longitudinal profile of the valley.
5.2 Sankt Wilhelmer Tal
The valley is one of the most impressive trough valleys in the Black Forest (Metz and Saurer, 2012). It was occupied by a branch of the Brugga palaeoglacier (Fig. 7). The first description of terminal moraines in this area dates back to the beginning of the 20th century (Klute, 1911). Glacial landforms in this area were first systematically investigated by Zienert (1973) and later mapped by the LGRB (Schreiner, 1977a). A more precise geological map at the 1:25 000 scale was later published (Hann et al., 2011) along with a detailed commentary (Schreiner, 2011). Previously mapped terminal moraines are depicted in Fig. 7, and prominent terminal moraines are shown in Fig. 8. Glacial cirques and moraines mapped in this study are presented in Fig. 9. A detailed geomorphological map is found in the supplementary material (Fig. S2). The ice-marginal positions are presented in an inverse chronological order starting with the terminal moraines in the Napf cirque and the main valley. For an overview of ice-marginal positions in this area, the reader is referred to the supplementary material (Table S1). Ice-marginal positions in the Wittenbach and Katzensteig cirques are detailed in the following two sections.
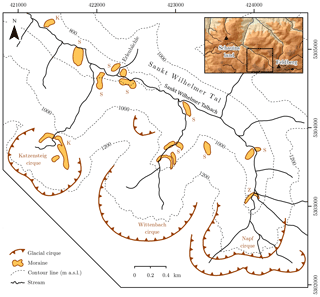
Figure 7Previously mapped glacial cirques and terminal moraines in Sankt Wilhelmer Tal as well as in the Katzensteig and Wittenbach cirques. K: Klute (1911); Z: Zienert (1973); S: Schreiner (Hann et al., 2011). See Fig. 1 for the data source of the shaded relief in the inset. Coordinate system: ETRS 1989 UTM Zone 32N.
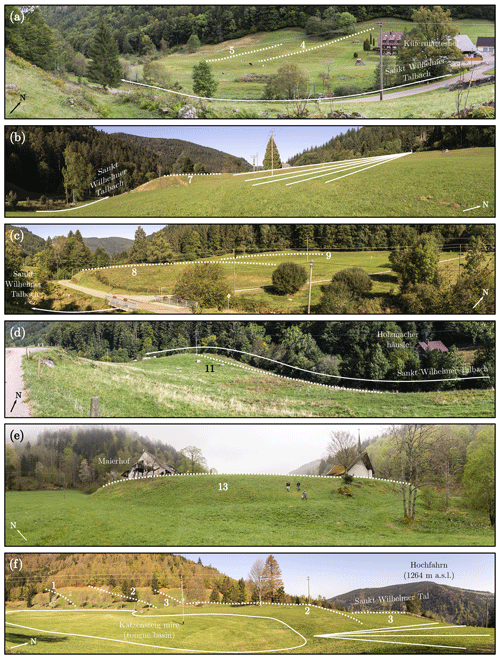
Figure 8Terminal moraines (dotted lines) in Sankt Wilhelmer Tal and in the Katzensteig cirque. The numbering refers to the ice-marginal position. (a) Two subdued terminal moraines are found near Küfermatteshof. (b) The terminal moraine of the ice-marginal position 7 is partly overlain by an alluvial fan (solid lines) of Erlenbächle. (c) The terminal moraines of the ice-marginal positions 8 and 9 caused the course of Sankt Wilhelmer Talbach to be redirected to the south. (d) The formation of the two-crested terminal moraine near Holzmacherhäusle led to a northward shift of Sankt Wilhelmer Talbach. (e) The prominent terminal moraine of the ice-marginal position 13 is situated at the western end of the village of Sankt Wilhelm. (f) A complete glacial series including a tongue basin, well-preserved terminal moraines (dotted lines) and a glaciofluvial terrace (solid lines) is observed in the Katzensteig cirque. The tongue basin is nowadays occupied by the Katzensteig mire. See Fig. 9 for the locations from where the photos were taken.
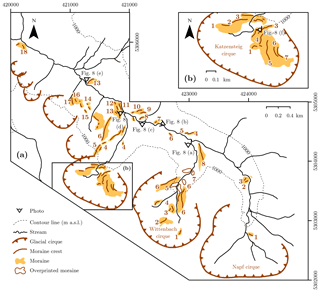
Figure 9(a) Confirmed and newly mapped terminal moraines as well as glacial cirques in Sankt Wilhelmer Tal and in the Wittenbach cirque. (b) Terminal moraines in the Katzensteig cirque. See Fig. S2 for a complete geomorphological map of Sankt Wilhelmer Tal and its tributary valleys. Coordinate system: ETRS 1989 UTM Zone 32N.
An ESE–WNW-oriented sediment ridge emerges from the eastern headwall of the Napf cirque at an elevation of 1030 m a.s.l. The sediment ridge is subparallel to the surrounding slope and about 100 m long. It is slightly asymmetric and features a smooth crest. These observations exclude a gravitational or fluvial formation and lead to the conclusion that the ridge is a terminal moraine. Since no terminal moraines have been identified further upvalley, it is assigned to ice-marginal position 1 (Fig. 9a). An ice-marginal position at 985 m a.s.l. (Fig. 7) further down the valley mentioned by Zienert (1973) was not identified here.
Another two-crested ridge is located at an elevation of 910 m a.s.l. at the entrance to the cirque (Fig. 7). As he identified outcropping bedrock, Zienert (1973) classified the ridge as a riegel. However, the ridge was later mapped as a two-crested terminal moraine (Fig. 7; Schreiner, 1977a; Hann et al., 2011). According to Schreiner (2011), it consists of coarse sediments as well as boulders. Due to the presence of two crests and the steep proximal and distal sides, the landform is probably as old as the outer terminal moraine at Feldsee in Seebachtal (Schreiner, 2011). The lack of visible bedrock at the surface on either side of the stream or in the crests themselves is an argument against the suggestion of Zienert (1973) that this feature is solely a riegel. Due to the lack of outcrops, it cannot be excluded that the feature is a combination of a riegel and a terminal moraine. The sediment ridge probably formed during a long stationary period characterised by the continuous dumping of debris from the ice margin. However, one key argument against this depositional model is the strong asymmetry of the feature, i.e. the steep distal side and the gently sloping proximal side. Terminal moraines related to gravitational processes are normally characterised by a flat distal and a steeper proximal side (Lukas and Rother, 2016). The reconstruction of the formation of the terminal moraine at 910 m a.s.l. requires further sedimentological observations. The crests of the terminal moraine are considered the ice-marginal positions 2 and 3 (Fig. 9a).
An accumulation of boulders is observed at an elevation of 850 m a.s.l. (Klute, 1911). It was considered an ice-marginal position by Zienert (1973). A dam for timber rafting was constructed on the NE flank of the feature (Klute, 1911). As the boulders of the terrace-shaped landform are partially rounded, Zienert (1973) suggested that it is a glacially transported rockslide. Since its morphology does not correspond to a terminal moraine, the landform is not considered an ice-marginal position in this study.
Two E–W-oriented ridges are found on the northern valley side near Küfermatteshof at an elevation of 820 m a.s.l. (Fig. 8a). The eastern ridge is about 80 m long, whereas the western ridge has a length of 50 m. The crests of the ridges are subdued, only 1–3 m high and oblique to the surrounding slope. Hence, a gravitational or fluvial formation is ruled out. It is more likely that the ridges are terminal moraines indicative of the ice-marginal positions 4 and 5 (Fig. 9a). Although the terminal moraines are quite conspicuous, they are described for the first time.
A ridge is found 200 m further downvalley on the southern valley side, situated at an elevation of 820 m a.s.l. and entirely consisting of partly rounded boulders. Hence, it is inferred that the boulders must have been transported for some distance. The feature has a SE–NW orientation, and it is 50 m long. As the ridge consisting of boulders is oblique to the surrounding slope, it is classified as a terminal moraine reflecting the ice-marginal position 6 (Fig. 9a). It has not been mentioned before.
Two further terminal moraines on the valley floor are described by Schreiner (2011) on both sides of the stream (Sankt Wilhelmer Talbach) at an elevation of 780 m a.s.l. (Fig. 7). The eastern moraine is partly overlain by an alluvial fan (Fig. 8b), with a smooth ridge south of the stream considered its counterpart (Hann et al., 2011). The northern part of the second terminal moraine 300 m further down the valley is described as a 50 m wide ridge oriented perpendicular to the orientation of the main valley (Figs. 7 and 8c). According to an outcrop at its southern end, the moraine consists of angular to rounded boulders (Schreiner, 2011). Its counterpart south of the stream is a subtle ridge emerging from the southern valley side (Hann et al., 2011). Both terminal moraines have been correlated with the Bärental stade (Schreiner, 2011). The presence of terminal moraines north of the stream is confirmed in this study, but we did not identify the counterparts of the moraines on the southern valley side. The terminal moraines are considered indicative of the ice-marginal positions 7 and 8 in this study (Fig. 9a).
Two sediment ridges slightly downvalley at an elevation of 780 m a.s.l. are first described in this study (Fig. 9a). The uppermost of these ridges (Fig. 8c) first starts parallel to the valley and curves to an ENE–WSW orientation on the western portion. The ridge rises 3 m above the surrounding area on the upvalley side to a rounded crest and then descends 0.5–1 m on the downvalley side. It is separated by 50 m from another 80 m long ridge (marked with “10” in Fig. 9a). It starts with an orientation parallel to the orientation of the main valley, but it curves into a NE to SW alignment as it progresses to the west. On the downvalley side of this ridge the surface changes from smooth grass-covered land to a rockier and rugged forest terrain with a continued increase in elevation. The lateral shape and orientation of these two ridges leads to the interpretation that they are a set of terminal moraines. The consistent terrain type and the noticeable crest of the upper ridge do strongly suggest a glacial genesis.
Another two-crested, 150 m wide terminal moraine is found at an elevation of 700–760 m a.s.l. (Fig. 7; Hann et al., 2011; Schreiner, 2011). Its formation caused a northward shift in the course of Sankt Wilhelmer Talbach (Fig. 8d). Due to its SSE–NNW orientation, the terminal moraine formed at the margin of a combined palaeoglacier from the Wittenbach and Napf cirques when the palaeoglacier from the Katzensteig cirque was already disconnected (Schreiner, 2011). The crests of the feature are assigned to the ice-marginal positions 11 and 12 (Fig. 9a).
A 50 m long sediment ridge is found south-west of the two-crested moraine. This feature is neither connected to a channel on the southern valley side nor located at the bottom of a steep slope. Hence, a gravitational or fluvial formation is considered unlikely. As the crest of the landform parallels the orientation of the main valley, the feature is considered a terminal moraine (Fig. 9a), described here for the first time. The landform is probably related to a prominent 100 m wide and 10–20 m high moraine at the western end of the village of Sankt Wilhelm (Fig. 8e). It documents the first unequivocal glacial standstill or readvance of the palaeoglaciers in the valley (Klute, 1911; Erb, 1948; Zienert, 1973; Schreiner, 2011). The arcuate terminal moraine at 700 m a.s.l. is oriented perpendicular to Sankt Wilhelmer Talbach (Fig. 7). Due to its supposed second-outermost position, the terminal moraine has been correlated with the Titisee stade (Erb, 1948; Zienert, 1973; Schreiner, 2011). As the maximum extent of the Brugga palaeoglacier remains unknown, the correlation with the terminal moraines at the margin of Titisee should be considered with care (Schreiner, 2011).
At the entrance to the two glacial cirques north and north-west of the Katzensteig cirque a set of sediment ridges with an asymmetric cross section is found. Due to the parallel orientation with regard to the main valley, these are considered terminal moraines. The lowermost series of five sediment ridges emerges from the southern valley side (marked with “14” in Fig. 9a). The two southernmost ridges at the lowest elevation have a N–S orientation, whereas the third ridge starts with a S–N orientation and changes subsequently its orientation to the north-west. Two other segments with a SE–NW orientation are found at 870 m a.s.l., west of the stream depicted in Fig. 9. As the southernmost two ridges have a N–S orientation, it is inferred that they were deposited at the margin of a combined palaeoglacier fed by the Katzensteig, Wittenbach and Napf cirques (Fig. 9a). As no terminal moraines have been identified on the valley floor downvalley from the terminal moraine of the ice-marginal position 13, the position of the palaeoglacier front during the deposition of the morphostratigraphically older terminal moraines cannot be determined. As the ends of the northernmost terminal moraines of these ice-marginal positions have a northward orientation, it seems unlikely that they are correlated with the terrace-shaped landform at the entrance to the valley. Due to this geometrical consideration, it is more likely that the palaeoglacier front was located somewhere between the terminal moraine at 700 m a.s.l. and the entrance to the valley during their deposition. The terrace-shaped feature at the entrance to the valley discussed above reflects the outermost ice-marginal position (Fig. 9a).
5.2.1 Wittenbach
The cirque features three swampy cirque floors at around 1170–1200, 1110 and 990 m a.s.l. (Schreiner, 2011). Terminal moraines mapped in earlier studies are shown in Fig. 7. For an overview of ice-marginal positions in the cirque, the reader is referred to the supplementary material (Table S2).
The uppermost sediment ridge at 1210 m a.s.l. (marked with “1” in Fig. 9a) has a N–S orientation and is the morphostratigraphically youngest terminal moraine. A SW–NE-oriented sediment ridge at 1170 m a.s.l. further north-west is also classified as a terminal moraine (marked with “2” in Fig. 9b). Two closely spaced N–S-oriented sediment ridges further north-east (marked with “3” and “4” in Fig. 9a) are considered terminal moraines indicative of the ice-marginal positions 3 and 4 in this study. A sediment ridge is found north-east of the intermediate cirque floor situated at an elevation of 1110 m a.s.l. Due to its arc shape, it is interpreted as a terminal moraine (marked with “4” in Fig. 9a). A terminal moraine at 1120 m a.s.l. is mentioned in the commentary to the most recent geological map of the area (Schreiner, 2011) but is not shown on the map itself (Hann et al., 2011). It is probably the terminal moraine at the northern end of the intermediate cirque floor shown on a previous geological map of the area (Schreiner, 1996b). We did not identify any terminal moraine at the margin of the intermediate cirque floor.
A set of terminal moraines is observed on the lowest cirque floor at 950–990 m a.s.l. A subdued hump on both sides of the stream at 990 m a.s.l. is interpreted as a terminal moraine in accordance with Schreiner (2011), but due to the lack of a distinct crest it is considered overprinted (Fig. 9a). The terminal moraine may be the local expression of the Feldsee stade (Schreiner, 2011). Another subdued hump is found at an elevation of 980 m a.s.l. at the eastern end of the lowermost cirque floor. It is interpreted as a modified terminal moraine, as it resembles the morphology of the overprinted terminal moraine at 990 m a.s.l. Two sediment ridges are observed on the eastern valley side further south-east (marked with “5” and “6” in Fig. 9a). Due to their oblique orientation with respect to the surrounding slope, they are considered terminal moraines associated with the frontal positions at 980 and 990 m a.s.l. The terminal moraine furthest downvalley on the lowermost cirque floor is situated at an elevation of 950 m a.s.l. (Schreiner, 2011). It is subdivided into two parts by a stream (Fig. 7). As both parts consist of sediments and have no distinct crest, they are interpreted as overprinted terminal moraines. A sediment ridge further south-west of the modified terminal moraine extending up to 1080 m a.s.l. on the western headwall of the cirque is classified as a terminal moraine (marked with “6” in Fig. 9a).
A prominent moraine is present at the entrance to the cirque (Fig. 7). It emerges from the eastern headwall in a SE direction up to an elevation of 900 m a.s.l. It could be an overridden medial moraine deposited between the confluent palaeoglaciers from the Wittenbach and Napf cirques (Schreiner, 2011) or a terminal moraine (Klute, 1911). As the northern end of the ridge progresses in a northerly direction up to the centre of the floor of the main valley, the feature is tentatively interpreted as a terminal moraine. It was probably deposited when the palaeoglacier from the cirque advanced into the main valley (Fig. 9a).
According to Schreiner (2011), another NW–SE-oriented medial moraine is found south-east of the terminal moraine (Fig. 7). As its deposition would require an upper limit of the corresponding palaeoglacier at around 1100 m a.s.l., the moraine was correlated with the last glaciation maximum (Schreiner, 2011). We were unable to identify this moraine. With eight ice-marginal positions presented here (Fig. 9a), the number of ice-marginal positions in the cirque is significantly higher than previously assumed (Schreiner, 2011; Fig. 7).
5.2.2 Katzensteig
Two floors at 1050 and 1020 m a.s.l. are found in the well-developed cirque, with the depression on the lower cirque floor being occupied by the Katzensteig mire (Figs. 8f, 10). Both of the cirque floors are surrounded by terminal moraines (Schreiner, 2011; Fig. 7). For a detailed geomorphological map of the cirque, the reader is referred to Fig. 10 and to Table S3 in the Supplement.
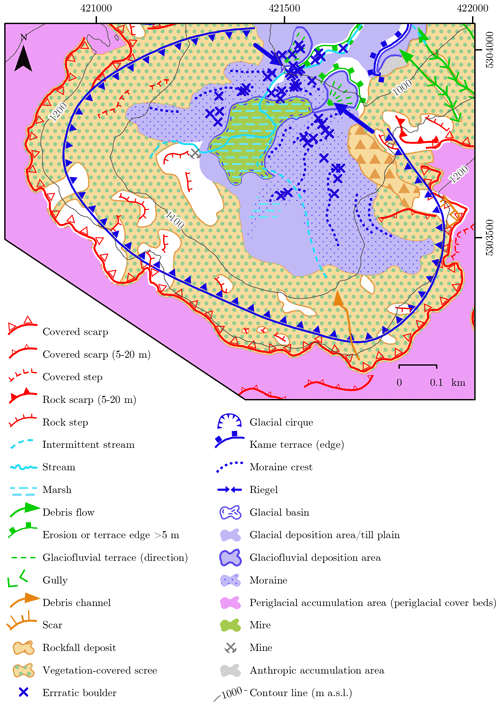
Figure 10Detailed geomorphological map of the Katzensteig cirque. This map is an extract of the geomorphological map of Sankt Wilhelmer Tal in the supplementary material (Fig. S2). Coordinate system: ETRS 1989 UTM Zone 32N.
The presence of a terminal moraine at the northern end of the upper cirque floor is confirmed here (Fig. 9a). The terminal moraine is attributed to a late glacial stage during a restricted glaciation of the cirque. Only one terminal moraine was previously mapped on the eastern headwall of the cirque (Fig. 7). In this study, a set of six N–S-oriented to SW–NE-oriented sediment ridges was identified at this locality (Fig. 9b). The ridges have a strongly asymmetric cross section with a steep western and a gently sloping eastern side. With the exception of the third-outermost feature, the ridges are separated from the surrounding slope. The asymmetric cross section and the separation from the surrounding slope are diagnostic geomorphological criteria for terminal moraines (Lukas et al., 2012a).
A two-crested terminal moraine was previously mapped at the northern end of the lower cirque floor at 1020 m a.s.l. (Fig. 7). The terminal moraine is confirmed here (Fig. 8f). It is probably as old as the terminal moraines at 910 and 990 m a.s.l. in the Napf and Wittenbach cirques, respectively (Schreiner, 2011) and has been correlated with the Feldsee stade (Klute, 1911; Schreiner, 2011). A set of three sediment ridges is found on the western headwall of the cirque (Fig. 8f). The oblique orientation to the surrounding slope leads to the rejection of the idea of a gravitational formation. Only one terminal moraine was previously mapped on the western headwall of the cirque (Fig. 7).
Six sediment cores from the mire in the centre of the lower cirque floor were obtained by the Baden-Württemberg State Institute for the Environment, Survey and Nature Conservation (LUBW; LUBW, 2009). Four of them are presented in Fig. 11. A succession of decayed peat and detritus gyttja with a thickness up to 12 m was identified in all sediment cores. As the Laacher See Tephra was apparently found below gyttja at the coring sites 2, 3 and 5, it is inferred that the lower cirque floor was ice-free and that lacustrine sedimentation occurred by 12.9 ka. Hence, all moraines at the margin of the mire should be older (Fig. 11).
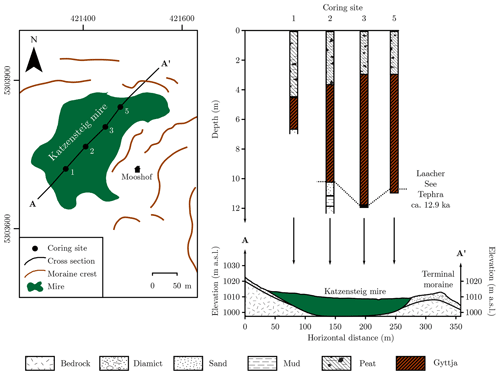
Figure 11Longitudinal profile of the Katzensteig mire and the terminal moraine at its northern end according to unpublished data of the LUBW (LUBW, 2009). See Fig. 2 for the location. Coordinate system: ETRS 1989 UTM Zone 32N.
A set of sediment ridges is observed further down the valley from the entrance of the cirque sensu stricto (Fig. 9a). It has not been mentioned before. The features are perpendicular or oblique to the surrounding slope. As the ridges are not related to an escarpment or a channel, a purely gravitational or fluvial formation is considered unlikely. Their formation is explained by the trapping of debris at the margin of a glacier and/or bulldozing by a glacier. This suggests that the ridges are terminal moraines. The two innermost terminal moraines (marked with “4” in Fig. 9a) formed when the glacier snout was located at around 890 m a.s.l. The next morphostratigraphically oldest terminal moraines (marked with “5” in Fig. 9a) imply that the glacier front was located at around 800 m a.s.l. during their formation. The orientation of the outermost moraine (marked with “6” in Fig. 9a) implies a location of the glacier terminus somewhere in the main valley. Overall, the analysis of the structure-from-motion-based DSM and the DTM together with geomorphological field mapping revealed seven ice-marginal positions (Fig. 9).
5.3 Zastler Tal
The valley and three of its tributary valleys (Rinkendobel, Angelsbach and Stollenbach) were occupied by glaciers during the Late Pleistocene. Moraines in these areas are presented and discussed starting with the uppermost ice-marginal position in the main valley, with previously mapped moraines shown in Fig. 12. Terminal moraines and glacial cirques mapped in this study are depicted in Fig. 13; the complete geomorphological map is found in the Supplement (Fig. S3). For an overview of ice-marginal positions, the reader is referred to the supplementary material (Table S4). Glacial features in the tributary valleys are presented in the three subsequent sections.
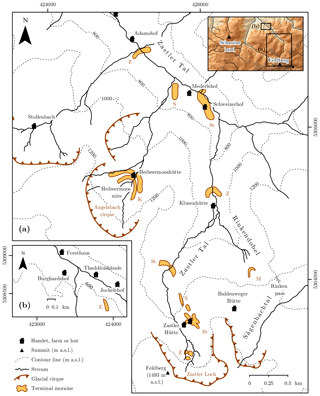
Figure 12Previously mapped glacial cirques and terminal moraines in (a) Zastler Tal as well as around (b) Jockelehof further downvalley. St: Steinmann (1902, 1910); K: Klute (1911); Z: Zienert (1973); S: Schreiner (Schreiner, 1977a; Wimmenauer et al., 1990); M: Metz (1985a). See Fig. 1 for the data source of the shaded relief in the inset. Coordinate system: ETRS 1989 UTM Zone 32N.
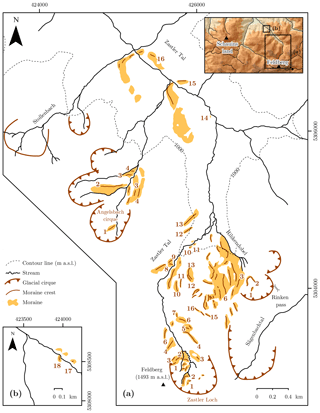
Figure 13Confirmed and newly mapped terminal moraines as well as glacial cirques in the (a) upper Zastler Tal and in the (b) lower Zastler Tal. The numbering of the moraines refers to the ice-marginal positions. See Fig. S4 for a complete geomorphological map of Zastler Tal and its tributary valleys. Coordinate system: ETRS 1989 UTM Zone 32N.
The uppermost part of Zastler Tal, Zastler Loch, is a prominent cirque with three cirque floors at 1400, 1320 and 1250 m a.s.l. (Metz, 1985b). The depression of the lowermost cirque floor is occupied by a mire. A set of four short sediment ridges is observed on the uppermost cirque floor at 1400–1420 m a.s.l. As they are neither associated with channels nor situated downvalley from escarpments, the features are considered terminal moraines of the ice-marginal position 1 (Fig. 13a). An arc-shaped sediment ridge is found at the NW end of the uppermost cirque floor. Due to its arcuate shape it is classified as a terminal moraine (Fig. 13a). The ice-marginal positions on the uppermost cirque floor are described for the first time.
A set of sediment ridges is observed at 1320–1340 m a.s.l. on the SE headwall of the cirque (Fig. 12a). Since they are arranged in an arcuate shape, the ridges are classified as terminal moraines in accordance with Zienert (1973). The Seebuck-Nischenkar stade was named after these landforms (Zienert, 1973). The features are here considered to be as old as the terminal moraine at the margin of the uppermost cirque floor, as the conditions for snow accumulation were more favourable in the SE corner of the cirque (Fig. 13a).
Two sediment ridges are observed north-east and north-west of the terminal moraines at 1320–1340 m a.s.l. Due to their arrangement in an arcuate shape, they are interpreted as terminal moraines of the ice-marginal position 3. The terminal moraines are mentioned here for the first time (Fig. 13a).
Steinmann (1902) already recognised that the NE margin of the lowermost cirque floor at 1250 m a.s.l. is surrounded by a terminal moraine (Fig. 12a). It was considered to be as old as (Metz, 1985b; Leser and Metz, 1988; Schreiner, 1990) or younger than the moraines at Feldsee (Schreiner, 1977b, 1981b; Schreiner, 1996a). A SW–NE-oriented ridge is found further west on the headwall of the cirque. As its orientation resembles that of a lamprophyre dike and of another dike of granite (Wimmenauer et al., 1990) and no outcropping bedrock is found, the ridge is interpreted as a combination of a riegel and a terminal moraine (Fig. S3). Both terminal moraines are assigned to the ice-marginal position 4 (Fig. 13a).
A set of five terminal moraines was mapped north and north-east of the end of the lowermost cirque floor (Fig. 12a; Wimmenauer et al., 1990). The terminal moraines were confirmed in this study and assigned to the ice-marginal positions 5, 6 and 7 (Fig. 13a). In contrast to Schreiner (1990), the moraines are not correlated with the terminal moraine at around 1030 m a.s.l., as their orientation indicates a position of the ice margin somewhere between the lowermost cirque floor and the terminal moraine at 1030 m a.s.l. (Fig. 13a). A relatively short sediment ridge is mapped on the centre of the valley floor further west. Its western end corresponds to the NE end of another sediment ridge on the western headwall of the cirque. As the two ridges are arranged in an arcuate shape together with the terminal moraine on the eastern headwall, the sediment ridges are classified as terminal moraines and assigned to the ice-marginal position 6 (Fig. 13a).
At an elevation of about 1030 m a.s.l., a prominent two-crested moraine is found (Fig. 12a). It was first mapped by Steinmann (1902) and later correlated with the Titisee stade (Steinmann, 1910). According to Zienert (1973), the moraine reflects the fourth-outermost ice-marginal position. Schreiner (1981a) assumed that this moraine is correlated with the moraine at the margin of the Feldsee mire in Seebachtal, whereas the terminal moraine further upvalley at 1250 m a.s.l. should be considered the equivalent of the moraines at the eastern shore of Feldsee. However, Leser and Metz (1988) rejected this interpretation, as the assignment of the two moraines at Feldsee to two distinct stades turned out to be arbitrary. Moreover, the rather large distance between the moraines in Zastler Tal (∼1200 m) and the significant difference in elevation (∼200 m) of the terminal moraines that have been correlated with the moraines at Feldsee led to the conclusion that the two-crested moraine at 1030 m a.s.l. should be correlated with the Waldhof stade and the terminal moraine at 1260 m a.s.l. with the moraines at Feldsee (Leser and Metz, 1988). However, this reasoning has not been taken into account in the commentaries to the geological maps of the areas (Schreiner, 1990, 1996a), where the two-crested moraine at 1030 m a.s.l. is still considered the local equivalent of the moraines at the margin of Feldsee.
A set of four arcuate sediment ridges is found further east. Due to their oblique orientation with respect to the surrounding slope, the features are interpreted as terminal moraines. The terminal moraines are mapped for the first time. This is also true for two short sediment ridges on the centre of the valley floor further north that are interpreted as terminal moraines. Two SW–NE-oriented sediment ridges are observed on the steeply inclined western valley side at 1010 and 1030 m a.s.l. further north. Since they are oriented oblique to the surrounding slope, the ridges are mapped as terminal moraines (Fig. 13a).
Zienert (1973) first described another ridge at around 860 m a.s.l. and interpreted it as a terminal moraine (Fig. 12a). According to him, it reflects the third-outermost ice-marginal position in Zastler Tal. It is noteworthy that the moraine is not shown on the most recent geological maps of the area (Wimmenauer et al., 1990; Schreiner, 1996b). We were unable to identify this terminal moraine. A small SW–NE-oriented sediment ridge is found further down the valley at 720 m a.s.l. Due to geomorphological considerations, i.e. the lack of a channel further uphill, the ridge is mapped as a terminal moraine (Fig. 13a). It has not been mentioned in the literature.
Steinmann (1910) first recognised another prominent ridge between Mederlehof and Schweizerhof (Fig. 12a). It is oriented perpendicular to the orientation of the valley floor. Zienert (1973) considered the landform on the southern valley side a large terminal moraine. Due to its supposed second-outermost morphostratigraphical position, both Hüttner (1967) and Zienert (1973) attributed the moraine to the Titisee stade. However, the ridge has never been mapped as a moraine by the LGRB (Schreiner, 1996b; Schreiner and Wimmenauer, 1999). As bedrock is outcropping on the entire NW flank of the feature, it is mapped as a riegel in this study. The terminal moraine interpretation of Zienert (1973) has to be rejected, as it does not reflect an ice-marginal position. The terrace-shaped landform south-east of the riegel is tentatively mapped as a glacial deposition area (Fig. S3). Further sedimentological investigations would be needed to evaluate this hypothesis. Interestingly, the riegel does not coincide with a dike consisting of bedrock that is more resistant to erosion according to the geological map of the area (Schreiner and Wimmenauer, 1999).
Another ridge-shaped accumulation of boulders parallel to the valley floor is observed south of Schweizerhof at an elevation of 940 m a.s.l. (Schreiner, 1996a). As this feature has been correlated with the last glaciation maximum, the ice must have been at least 230 m thick. However, the ridge aligns well with a terminal moraine at 640 m a.s.l. (Fig. 13a) first mapped by Schreiner (1977a). The terminal moraine emerges from the southern valley side and is partly covered by an alluvial fan at its northern end (Schreiner, 1996b). It was suggested that the feature formed during the Titisee stade (Schreiner, 1996a; Metz and Saurer, 2012) and is attributed to the ice-marginal position 15 in this study (Fig. 13a).
A morphostratigraphically older ridge on the valley floor has been identified near Adamshof at an elevation of roughly 600 m a.s.l. (Fig. 12a). Hüttner (1967) argued that the bedrock in this area is more resistant to erosion, and accordingly, the ridge was classified as a roche moutonnée. The ridge was later considered a terminal moraine and classified as the furthest downvalley ice-marginal position during the last glaciation maximum, as the ice-marginal position at Jockelehof further downvalley was not confirmed (Zienert, 1973). Outcropping bedrock was not observed on the ridge during field mapping for this study. The feature is either a combination of a riegel and a terminal moraine or simply a terminal moraine. The hypothesis that a part of the feature is a riegel can only be dismissed by further inspecting the subsurface. Irrespective of this discussion, the ridge reflects an ice-marginal position (Fig. 13a).
According to Hüttner (1967), a ridge-shaped accumulation of boulders is found further south-west on the southern valley side between the entrance to Stollenbach and the entrance to Angelsbach. The feature is situated at an elevation of 770–780 m a.s.l. Since the feature is parallel to the valley floor, it was considered indicative of the ice extent during the last glaciation maximum. Hence, the ice thickness must have exceeded 100 m at this locality. The ridge is probably related to the terminal moraine of the ice-marginal position 16 at Adamshof (Fig. 13a).
On the southern valley side near Jockelehof, a remnant of a terminal moraine was identified (Fig. 12b; Erb, 1948; Hüttner, 1967). Without any independent chronological evidence, this feature at an elevation of 540–600 m a.s.l. was considered indicative of the last glaciation maximum ice extent (Hüttner, 1967; Metz and Saurer, 2012). In this study, the subtle, N–S-oriented ridge on the southern valley side is confirmed. However, a gravitational genesis of the sediment ridge cannot be excluded. Hence, the feature is tentatively mapped as a terminal moraine indicative of the ice-marginal position 18 (Fig. 13b). Three ridges are observed on the northern valley side. According to outcrops at their SW ends, poorly rounded clasts and boulders reside in a matrix composed of finer sediments. The ridges interfinger with a cone-shaped feature further north-east that is interpreted as an alluvial fan (see Fig. S3 for a complete geomorphological map of the area). Only the SE ridge features a distinct crest. Due to their orientation perpendicular to the valley floor, the ridges are classified as terminal moraines (Fig. 13b). Whether the ridges at Jockelehof are correlated with the last glaciation maximum cannot be determined without chronological data.
5.3.1 Rinkendobel
Two amphitheatre-shaped niches in the upper part of the SE–NW-trending valley are interpreted as glacial cirques in this study (Fig. 13a). The Rinken pass separates the valley from Sägenbachtal further east. During the last glaciation maximum, the palaeoglacier in Zastler Tal was probably fed by a branch from Zastler Loch and a transfluent ice stream from the cirque in the uppermost Sägenbachtal (Fig. 12a; Metz, 1985b; Schreiner, 1990). As till is found up to an elevation of 1250 m a.s.l. north of the Rinken pass (Wimmenauer et al., 1990), it was concluded that the ice must have been at least 50 m thick at this locality (Schreiner, 1990). Numerous channels are observed on the southern valley side further west. One of these channels is roughly 300 m long. They were classified as meltwater channels formed at the ice margin (Schreiner, 1996a).
Both the analysis of remote sensing data and field mapping reveal that numerous sediment ridges are present in this area. They are separated by deep channels from the surrounding slope. Therefore, we suggest that the ridges and the channels are terminal moraines and lateral meltwater channels, respectively (Fig. 13a).
A subtle, partly eroded terminal moraine is found on the valley floor at an elevation of 1100 m a.s.l. (marked with “M” in Fig. 12; Metz, 1985a). This moraine was shaped during a restricted cirque glaciation (Metz, 1985b, 1997; Metz and Saurer, 2012). Initially correlated with the outer moraine at Feldsee in Seebachtal (Metz, 1985b), the moraine was later attributed to the Waldhof stade (Metz and Saurer, 2012). An arcuate sediment ridge further uphill is interpreted as a terminal moraine (marked with “1” in Fig. 12). Overall, the terminal moraines in the valley are assigned to 16 ice-marginal positions (Fig. 13a).
5.3.2 Angelsbach
The upper part of the valley is a well-developed cirque with two cirque floors. Its headwall reaches up to 1300 m a.s.l. The upper cirque floor is situated at 1220–1240 m a.s.l. The depression on the significantly larger cirque floor at around 1080 m a.s.l. is covered by the Heibeermoos mire, surrounded by two generations of terminal moraines (Fig. 12a; Schreiner, 2011). Sediment cores from the mire were first obtained by Friedmann (1998/1999) and later by the LUBW (LUBW, 2010; Fig. 14). A bulk sample from the base of the sediment core from the centre of the mire was dated to 7303–6954 cal BP (median probability 7148 cal BP; Fig. 15), thereby implying that peat formation on the cirque floor started at ca. 7 ka (Friedmann, 1998/1999). In contrast, the considerably older Laacher See Tephra was apparently identified in a sediment core from the NE part of the mire obtained by LUBW (2010; Fig. 14). This implies that the terminal moraines at the margin of the mire must be at least 12.9 kyr old. The presence of Laacher See Tephra in both the Heibeermoos and the Katzensteig mires would agree with the hypothesis of Schreiner (2011) that the moraines at their margins are possibly of the same age.
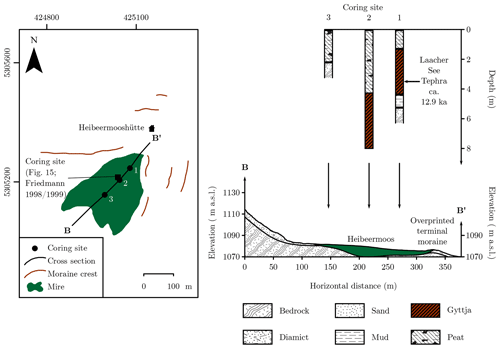
Figure 14Longitudinal profile of the Heibeermoos mire according to unpublished data of the LUBW (LUBW, 2010). See Fig. 2 for the location. Coordinate system: ETRS 1989 UTM Zone 32N.
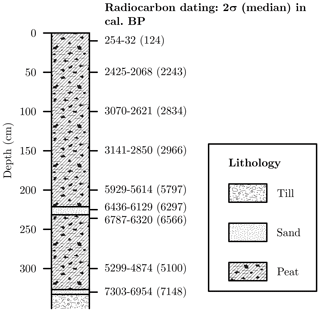
Figure 15Lithology of the sediment core obtained from the centre of the Heibeermoos mire as well as recalibrated radiocarbon ages (Friedmann, 1998/1999). The coring site is depicted in Fig. 14.
A sediment ridge was mapped at the northern end of the upper cirque floor. As the ridge is not associated with a channel or an escarpment, a fluvial or gravitational formation is considered unlikely. The ridge is probably a terminal moraine and developed during a period of stationary glaciers when the glaciation of the cirque was restricted to its very uppermost part (Fig. 13a). This terminal moraine at 1210 m a.s.l. has not been mentioned before. The terminal moraines directly at the margin of the Heibeermoos mire (Schreiner, 2011) are confirmed in this study. Thanks to the high-resolution remote sensing data, it was observed that the outer moraine of these moraines has two distinct crests. This assumption was later confirmed during geomorphological field mapping.
5.3.3 Stollenbach
Till was mapped on the valley floor at an elevation of 720–840 m a.s.l. (Wimmenauer and Hüttner, 1968). Its occurrence on the valley floor has been considered an indication that the ice from the cirque near the hamlet of Stollenbach reached the main Zastler Tal during the last glaciation maximum (Hüttner, 1967). However, the deposit may also be fluvially reworked till. Secondly, the valley features a strong V-shape north of the glacial cirque on the southern valley side (Fig. 13a). Note in particular the V-shaped 800 m a.s.l. contour line in Fig. 12. Due to these observations, we argue that the glaciation was restricted to the cirque on the southern valley side and the poorly developed cirque in the uppermost part of the valley (Fig. 13a).
As we identified terminal moraines associated with 18 ice-marginal positions in Sankt Wilhelmer Tal and Zastler Tal (Figs. 9 and 13), it is inferred that the deglaciation of the southern Black Forest was interrupted by more glacial standstills and/or glacial readvances than previously expected. The presence of terminal moraines associated with such a high number of ice-marginal positions is not surprising. In contrast to larger ice bodies, smaller glaciers react to climatic oscillations on short timescales (Mackintosh et al., 2017). Secondly, the palaeoglaciers were probably temperate or polythermal, as were former ice fields in Scotland (Lukas, 2005; Lukas and Benn, 2006; Boston et al., 2015). As the topographical control became stronger during the deglaciation, it is likely that at least some of the periods of moraine formation were topographically driven. For example, the palaeoglacier in the Katzensteig cirque may have stabilised during the retreat to the cirque despite a general trend of deglaciation. As the valley geometry may significantly impact the behaviour of glaciers (Boston and Lukas, 2019), the terminal moraine record north-west of the Feldberg should be cautiously interpreted with regard to the palaeoclimate without additional evidence. Disentangling topographically driven and climatically forced periods of moraine formation (Barr and Lovell, 2014) will be one of the key challenges in future research. The application of numerical dating methods only provides the age of an ice-marginal position (Lüthgens and Böse, 2012) and, in most cases, little information on the forcing and nature of glacial events (such as whether there was glacial advance or standstill during general retreat). These information gaps can only be filled by investigating the sediments within moraines (Winkler and Hagedorn, 1999; Lukas, 2007; Reznichenko et al., 2016). Thus, terminal moraines in the southern Black Forest will be sedimentologically investigated to provide insights into the forcing of the glacial events.
Most of the previously described ice-marginal positions in the Schauinsland area are confirmed (Fig. 6). Previously suggested ice-marginal positions in Hörnegrund, at the entrance to Hörnegrund, and between Sankt Wilhelmer Tal and Hörnegrund as well as in Langenbach have to be rejected. The terminal moraine at 600 m a.s.l. in Kappeler Tal and the outer terminal moraine in the Sailentobel cirque are described for the first time. Overall, the western part of our study area is conspicuously poor in glacial landforms. This has been explained by the lack of large, high-altitude areas favourable for glacial accumulation (Giermann, 1964). Only an insignificant portion of this region is located above 1200 m a.s.l. (Fig. 4), whereas the upper parts of the valleys in the eastern part of our study area are situated at considerably higher elevations (Figs. 7 and 12). Due the lack of significant topographical control, the palaeoglaciers were not well sheltered from incoming solar radiation with the exception of the S–N-oriented palaeoglacier in Kappeler Tal. The overall smooth topography (Fig. 2) also limited the amount of debris available for terminal moraine formation. The only exception is the upper Kappeler Tal where large escarpments served as debris sources. Thirdly, the upper parts of the valleys are very steep (Fig. 2). To summarise, the scarcity of terminal moraines is probably explained by an early deglaciation, the lack of sediments for moraine formation and the overall steep longitudinal profiles of the valleys. These factors led to the situation that the deglaciation in the western part of our study area was already underway when large areas around the Feldberg were still covered by glaciers, as already proposed by Giermann (1964).
The review of the little available indirect chronological information on Late Pleistocene glacier fluctuations in the Black Forest highlights fundamental disagreements. This is particularly true for the Heibeermoos mire. According to the data of the LUBW (LUBW, 2010; Fig. 14), the Laacher See Tephra was deposited in the centre of the mire, whereas Friedmann (1998/1999) suggested a significantly later onset of peat formation at 7303–6954 cal BP. Two explanations are invoked for this disagreement. Firstly, the unit of greyish sediments at a depth of 3.5 m in the centre of the mire was erroneously classified as tephra by LUBW (LUBW, 2010; coring site 1 in Fig. 14). This explanation is not unrealistic, as tephra was found in sediment cores from the mires in Seebachtal on top of detritus gyttja and below clayey gyttja supposedly younger than the cooling in Europe at 12.8 ka (Fig. 3). This succession of sediments matches well the palaeoclimatic context of the last glacial termination in the Alps and its forelands (Heiri et al., 2014). Hence, one would expect a similar succession of lithostratigraphic units in the mires in our study area. However, the Laacher See Tephra in the Katzensteig mire was found above sand and mud and below gyttja (Fig. 11). In the Heibeermoos mire it was supposedly identified in a unit of gyttja (Fig. 14). The peculiar succession of lithostratigraphic units in both mires calls the reliability of the data of the LUBW (2010) into question. Alternatively, the bulk samples for radiocarbon dating used by Friedmann (1998/1999) yielded erroneous results due to contamination of the sampled material. For example, root contamination may have caused the abnormally young radiocarbon ages (Törnqvist et al., 1992; Piotrowska et al., 2011).
The Laacher See Tephra has been used in the southern Black Forest as a chronological marker to infer timberline changes (Lang, 2006) and glacier fluctuations (Lang et al., 1984; Merkt, 1985; Lang, 2005). However, this approach requires the Laacher See Tephra to be unequivocally identified via geochemical analyses to prevent a silt layer being erroneously classified as tephra. A sufficient number of macrofossil samples for radiocarbon dating should be obtained from the mires presented above. Apart from potential problems associated with incomplete bleaching, OSL dating of lake sediments should be tested (e.g. Lukas et al., 2012b). The age of the basal sediments would be particularly interesting, as both mires are delimited by terminal moraines with large boulders suitable for TCN dating.
Obtaining chronological constraints from terminal moraines is considered the most important step in future research on Late Pleistocene glacier variations in the southern Black Forest. As the current subdivision of the last glaciation of the southern Black Forest relies on terminal moraines in Seebachtal and Zastler Tal (Fig. 1), it would be most desirable to determine their age with TCN dating. According to our own observations, boulders suitable for TCN dating are only present on the terminal moraines at the margin of Feldsee and in the uppermost part of Zastler Tal. As suitable boulders for TCN dating are found on half of the terminal moraines in Sankt Wilhelmer Tal and quartz-rich lithologies dominate the surrounding area, future efforts should be concentrated on exposure dating these landforms. Hence, the subdivision of the last glaciation presented above will be replaced by an event stratigraphy based on exposure ages from this valley.
To revisit the initial question of the timing of the last glaciation maximum, terminal moraines outside our study area, such as in the area of the Haslach palaeoglacier (Bangert et al., 1992), should be geomorphologically and, if possible, sedimentologically reinvestigated. In addition to TCN dating, luminescence depth profiles from boulders from moraines (Rades et al., 2018) should be simultaneously reconstructed to provide an additional line of evidence if the approach is successfully tested. As discussed for fluvial environments (Rixhon et al., 2017), we strongly advocate the application of multiple dating methods, as TCN dating has proven to be problematic for moraines in anthropogenically impacted areas (Boxleitner et al., 2018). Hence, luminescence depth profiles may help to circumvent problems if exposure ages from boulders turn out to be anomalously young.
The review of previous studies on the glaciation north-west of the Feldberg highlights disagreements between former studies regarding the location of ice-marginal positions, in particular for Zastler Tal. In this study, we both newly mapped ice-marginal landforms and rejected previously mentioned ice-marginal landforms. Detailed geomorphological mapping proved to be a prerequisite for the successful application of numerical dating methods. The application of a holistic approach involving both geomorphological field mapping and the analysis of remote sensing data enabled ice-marginal landforms north-west of the Feldberg to be mapped with an unprecedented level of detail. In two of the mapped valleys, 18 ice-marginal positions were identified, thereby reinforcing the hypothesis that the palaeoglaciers in the southern Black Forest were highly dynamic. The scarcity of ice-marginal positions in the Schauinsland area further west is attributed to the lack of high-altitude accumulation areas, the steep longitudinal profile of the formerly glaciated valleys and the lack of sediments available for terminal moraine formation.
The review of the few indirect chronological data on the last glaciation shows that the chronology of glacier fluctuations in the southern Black Forest is far from being understood. Most of the terminal moraines used for the subdivision of the last glaciation lack suitable boulders for TCN dating. Hence, the existing subdivision will be abandoned and replaced by a new event stratigraphy based upon exposure ages from Sankt Wilhelmer Tal. Suspected last-glaciation-maximum terminal moraines outside of our study area will be reinvestigated and numerically dated to determine the timing of this event. Recent innovative approaches in OSL dating, such as depth profiles from moraines, will simultaneously be tested to provide an independent age control. Due to contrasting results of earlier studies and to the sediments' location inside terminal moraines, it is intended that the sediments of the mires in our study area will be reinvestigated.
An overview of ice-marginal positions in Sankt Wilhelmer Tal, the Wittenbach cirque, the Katzensteig cirque and Zastler Tal; a description of the establishment of the DSM of the Katzensteig cirque; and detailed geomorphological maps of key areas are found in the Supplement. All other data are available from the corresponding author upon reasonable request.
The supplement related to this article is available online at: https://doi.org/10.5194/egqsj-69-61-2020-supplement.
FP and FMH designed the study. FMH, JPB, WM and FR performed geomorphological mapping. FMH led the writing of the manuscript with contributions from all other authors.
The authors declare that they have no conflict of interest.
This study was conducted during the PhD project of Felix Martin Hofmann, the bachelor's thesis projects of Florian Rauscher and Jan-Paul Bischoff, and a geological project of William McCreary. We thank the local farmers and landowners, particularly Martin Rudiger and Roland Klingele, for permission to perform geomorphological mapping on their properties. The forestry department of the Breisgau-Hochschwarzwald county is acknowledged for a forest access permit. We are indebted to Heinz-Otto Kühl (LUBW) for providing unpublished coring data from the Katzensteig and Heibeermoos mires. Johannes Miocic is thanked for the drone survey in the Katzensteig cirque. The support of Gerwin Wulf during this study is gratefully appreciated. We thank the staff at the State Geological Survey of Baden Württemberg, in particular Ulrike Wielandt-Schuster and Matthias Franz, for insightful discussions that helped to improve this manuscript. Wolfgang Wasser is acknowledged for providing maps of suspected glacial features in our study area. Stefan Winkler and the anonymous reviewer are thanked for their constructive comments on an earlier version of this manuscript. The constructive comments of the associate editor, Gilles Rixhon, were gratefully appreciated.
This research has been supported by the German Research Foundation (grant no. 426333515) and the Studienstiftung des Deutschen Volkes, through a PhD scholarship to Felix Martin Hofmann. The article processing charge was funded by the Baden-Württemberg Ministry of Science, Research and Art and the University of Freiburg in the funding programme Open Access Publishing.
This paper was edited by Christopher Lüthgens and Gilles Rixhon and reviewed by Stefan Winkler and one anonymous referee.
Balco, G.: Glacier Change and Paleoclimate Applications of Cosmogenic-Nuclide Exposure Dating, Annual Rev. Earth Pl. Sc., 48, 21–48, https://doi.org/10.1146/annurev-earth-081619-052609, 2020.
Bangert, V., Schreiner, A., and Etzold, A.: Geologische Karte von Baden-Württemberg 1:25000, Blatt 8115 Lenzkirch, Landesvermessungsamt Baden-Württemberg, Stuttgart, 1992.
Barr, I. D. and Lovell, H.: A review of topographic controls on moraine distribution, Geomorphology, 226, 44–64, https://doi.org/10.1016/j.geomorph.2014.07.030, 2014.
Boston, C. M. and Lukas, S.: Topographic controls on plateau icefield recession: insights from the Younger Dryas Monadhliath Icefield, Scotland, J. Quaternary Sci., 34, 433–451, https://doi.org/10.1002/jqs.3111, 2019.
Boston, C. M., Lukas, S., and Carr, S. J.: A Younger Dryas plateau icefield in the Monadhliath, Scotland, and implications for regional palaeoclimate, Quaternary Sci. Rev., 108, 139–162, https://doi.org/10.1016/j.quascirev.2014.11.020, 2015.
Boxleitner, M., Ivy-Ochs, S., Brandova, D., Christl, M., Egli, M., and Maisch, M.: Piecing together the Lateglacial advance phases of the Reussgletscher (central Swiss Alps), Geogr. Helv., 73, 241–252, https://doi.org/10.5194/gh-73-241-2018, 2018.
Bronk Ramsey, C.: Bayesian Analysis of Radiocarbon Dates, Radiocarbon, 51, 337–360, https://doi.org/10.1017/S0033822200033865, 2009.
Chandler, B. M. P., Lovell, H., Boston, C. M., Lukas, S., Barr, I. D., Benediktsson, Í. Ö., Benn, D. I., Clark, C. D., Darvill, C. M., Evans, D. J. A., Ewertowski, M. W., Loibl, D., Margold, M., Otto, J.-C., Roberts, D. H., Stokes, C. R., Storrar, R. D., and Stroeven, A. P.: Glacial geomorphological mapping: A review of approaches and frameworks for best practice, Earth-Science Rev., 185, 806–846, https://doi.org/10.1016/j.earscirev.2018.07.015, 2018.
Duprat-Oualid, F., Rius, D., Bégeot, C., Magny, M., Millet, L., Wulf, S., and Appelt, O.: Vegetation response to abrupt climate changes in Western Europe from 45 to 14.7k cal a BP: the Bergsee lacustrine record (Black Forest, Germany), J. Quaternary Sci., 32, 1008–1021, https://doi.org/10.1002/jqs.2972, 2017.
Duprat-Oualid, F., Peyron, O., Bégeot, C., Magny, M., Millet, L., and Rius, D.: Pollen-inferred quantification of the climate variability of the last 50 ka from the Bergsee record (Black Forest, Germany), Quaternary Sci. Rev., in press., 2020.
Erb, L.: Die Geologie des Feldbergs, in: Der Feldberg im Schwarzwald, edited by: Müller, K., L. Bielefelds Verlag KG, Freiburg i. Br., 22–96, 1948.
Florineth, D. and Schlüchter, C.: Alpine Evidence for Atmospheric Circulation Patterns in Europe during the Last Glacial Maximum, Quaternary Res., 54, 295–308, https://doi.org/10.1006/qres.2000.2169, 2000.
Friedmann, A.: Pollenanalytische Untersuchungen zur holozänen Vegetations- und Landschaftsgeschichte des westlichen Hochschwarzwalds, Ber. Naturf. Ges. Freiburg i. Br., 88/89, 57–84, 1998/1999.
Giermann, G.: Die würmeiszeitliche Vergletscherung des Schauinsland Trubelsmattkopf-Massivs (westlicher Südschwarzwald) Diploma thesis, University of Freiburg, Freiburg, 56 pp., 1959.
Giermann, G.: Die würmeiszeitliche Vergletscherung des Schauinsland-Trubelsmattkopf-Knöpflesbrunnen-Massivs (südlicher Schwarzwald), Ber. Naturf. Ges. Freiburg i. Br., 54, 197–208, 1964.
Hann, H. P., Schreiner, A., and Zedler, H.: Geologische Karte von Baden-Württemberg 1:25 000, Blatt 8113 Todtnau, Landesamt für Geologie, Rohstoffe und Bergbau, Freiburg i. Br., 2011.
Heiri, O., Koinig, K. A., Spötl, C., Barrett, S., Brauer, A., Drescher-Schneider, R., Gaar, D., Ivy-Ochs, S., Kerschner, H., Luetscher, M., Moran, A., Nicolussi, K., Preusser, F., Schmidt, R., Schoeneich, P., Schwörer, C., Sprafke, T., Terhorst, B., and Tinner, W.: Palaeoclimate records 60–8 ka in the Austrian and Swiss Alps and their forelands, Quaternary Sci. Rev., 106, 186–205, https://doi.org/10.1016/j.quascirev.2014.05.021, 2014.
Hemmerle, H., May, J.-H., and Preusser, F.: Übersicht über die pleistozänen Vergletscherungen des Schwarzwaldes, Ber. Naturf. Ges. Freiburg i. Br., 106, 31–67, 2016.
Hofmann, F. M., Alexanderson, H., Schoeneich, P., Mertes, J. R., Léanni, L., and ASTER Team: Post-Last Glacial Maximum glacier fluctuations in the southern Écrins massif (westernmost Alps): insights from 10Be cosmic ray exposure dating, Boreas, 48, 1019–1041, https://doi.org/10.1111/bor.12405, 2019.
Hüttner, R.: Das Quartär, in: Geologische Karte von Baden-Württemberg 1:25 000, Erläuterungen zu Blatt 8013 Freiburg-Südost, edited by: Geologisches Landesamt Baden-Württemberg, Landesvermessungsamt Baden-Württemberg, Stuttgart, 69–105, 1967.
Ivy-Ochs, S., Schlüchter, C., Kubik, P. W., Synal, H.-A., Beer, J., and Kerschner, H.: The exposure age of an Egesen moraine at Julier Pass, Switzerland, measured with the cosmogenic radionuclides 10Be, 26Al and 36Cl, Eclogae Geol. Helv., 89, 1049–1063, 1996.
Ivy-Ochs, S., Kerschner, H., Kubik, P. W., and Schlüchter, C.: Glacier response in the European Alps to Heinrich Event 1 cooling: the Gschnitz stadial, J. Quaternary Sci., 21, 115–130, https://doi.org/10.1002/jqs.955, 2006.
Ivy-Ochs, S.: Glacier variations in the European Alps at the end of the last glaciation, Cuadernos de Investigación Geográfica, 41, 219–315, https://doi.org/10.18172/cig.2750, 2015.
Kerschner, H. and Ivy-Ochs, S.: Palaeoclimate from glaciers: Examples from the Eastern Alps during the Alpine Lateglacial and early Holocene, Global Planet. Change, 60, 58–71, https://doi.org/10.1016/j.gloplacha.2006.07.034, 2008.
Klute, F.: Die Schneereste des Schwarzwaldes im Frühsommer und die Beziehungen ihrer Lage zu den Stellen ehemaliger Vergletscherung, Ber. Naturf. Ges. Freiburg i. Br., 19, 61–116, 1911.
Kuhlemann, J., Rohling, E. J., Krumrei, I., Kubik, P., Ivy-Ochs, S., and Kucera, M.: Regional Synthesis of Mediterranean Atmospheric Circulation During the Last Glacial Maximum, J. Science, 321, 1338–1340, https://doi.org/10.1126/science.1157638, 2008.
Lang, G.: Seen und Moore des Schwarzwaldes als Zeugen spätglazialen und holozänen Vegetationswandels. Stratigraphische, pollenanalytische und großrestanalytische Untersuchungen, Andrias, edited by: Staatliches Museum für Naturkunde Karlsruhe, Karlsruhe, 160 pp., 2005.
Lang, G.: Late-glacial fluctuations of timberline in the Black Forest (SW Germany), Veg. Hist. and Archaeobot., 15, 373–375, https://doi.org/10.1007/s00334-006-0048-8, 2006.
Lang, G., Merkt, J., and Streif, H.: Spätglazialer Gletscherrückzug und See- und Moorentwicklung im Südschwarzwald, Südwestdeutschland, Diss. Bot., 72 (Festschrift Max Welten), 213–234, 1984.
Leser, H. and Metz, B.: Vergletscherungen im Hochschwarzwald, Berliner Geogr. Abh., 47, 155–175, 1988.
Liehl, E.: Landschaftsgeschichte des Feldberggebietes, in: Der Feldberg im Schwarzwald: subalpine Insel im Mittelgebirge, edited by: Landesanstalt für Umweltschutz Baden-Württemberg Institut für Ökologie und Naturschutz, Karlsruhe, 1982.
LUBW: St. Wilhelm-Katzensteig, available at: http://www2.lubw.baden-wuerttemberg.de/public/abt2/dokablage/oac_77/558.htm (last access: 13 June 2020), 2009.
LUBW: Heibeermoos, http://www2.lubw.baden-wuerttemberg.de/public/abt2/dokablage/oac_77/559.htm (last access: 13 June 2020), 2010.
Luetscher, M., Boch, R., Sodemann, H., Spötl, C., Cheng, H., Edwards, R. L., Frisia, S., Hof, F., and Müller, W.: North Atlantic storm track changes during the Last Glacial Maximum recorded by Alpine speleothems, Nat. Commun., 6, 6344, https://doi.org/10.1038/ncomms7344, 2015.
Lukas, S.: Early-Holocene glacier fluctuations in Krundalen, south central Norway: palaeoglacier dynamics and palaeoclimate, Holocene, 17, 585–598, https://doi.org/10.1177/0959683607078983, 2007.
Lukas, S.: A test of the englacial thrusting hypothesis of `hummocky' moraine formation: case studies from the northwest Highlands, Scotland, Boreas, 34, 287–307, https://doi.org/10.1111/j.1502-3885.2005.tb01102.x, 2005.
Lukas, S. and Benn, D. I.: Retreat dynamics of Younger Dryas glaciers in the far NW Scottish Highlands reconstructed from moraine sequences, Scot. Geogr. J., 122, 308–325, https://doi.org/10.1080/14702540701235142, 2006.
Lukas, S. and Rother, H.: Moränen versus Till: Empfehlungen für die Beschreibung, Interpretation und Klassifikation glazialer Landformen und Sedimente, E&G Quaternary Sci. J., 65, 95–112, https://doi.org/10.3285/eg.65.2.01, 2016.
Lukas, S., Graf, A., Coray, S., and Schlüchter, C.: Genesis, stability and preservation potential of large lateral moraines of Alpine valley glaciers – towards a unifying theory based on Findelengletscher, Switzerland, Quaternary Sci. Rev., 38, 27–48, https://doi.org/10.1016/j.quascirev.2012.01.022, 2012a.
Lukas, S., Preusser, F., Anselmetti, F. S., and Tinner, W.: Testing the potential of luminescence dating of high-alpine lake sediments, Quat. Geochronol., 8, 23–32, https://doi.org/10.1016/j.quageo.2011.11.007, 2012b.
Lüthgens, C. and Böse, M.: From morphostratigraphy to geochronology – on the dating of ice marginal positions, Quaternary Sci. Rev., 44, 26–36, https://doi.org/10.1016/j.quascirev.2010.10.009, 2012.
Mackintosh, A. N., Anderson, B. M., and Pierrehumbert, R. T.: Reconstructing Climate from Glaciers, Annual Rev. Earth Pl. Sc., 45, 649–680, https://doi.org/10.1146/annurev-earth-063016-020643, 2017.
Meinig, R.: Die würmeiszeitliche Vergletscherung im Gebiet Breitnau-Hinterzarten-Neustadt (Schwarzwald), PhD thesis, University of Freiburg, Freiburg, 166 pp., 1966.
Meinig, R.: Halte und Eisrandbildungen des würmzeitlichen Bärental-Gletschers, Südschwarzwald, Late- and postglacial oscillations of glaciers: glacial and periglacial landforms, Trier, 1980, 257–282, 1980.
Merkt, J.: Exkursionspunkt: “Feldsee”. Bohrung Feldsee-Mitte, in: 22. wissenschaftliche Tagung in Freiburg i. Br. September 1985. Exkursionsführer 1. Südschwarzwald, Oberschwaben/Bodensee, südliches Oberrheingebiet, edited by: Schreiner, A. and Metz, B., Deutsche Quartärvereinigung, Hannover, 39–41, 1985.
Metz, B.: Geomorphologische Karte 1:25 000 der Bundesrepublik Deutschland. GMK 25 Blatt 21. 8114 Feldberg, GMK-Schwerpunktprogramm der Deutschen Forschungsgemeinschaft (DFG), Berlin, 1985a.
Metz, B.: Erläuterungen zur Geomorphologischen Karte 1:25 000 der Bundesrepublik Deutschland, GMK 25 Blatt 21, 8114 Feldberg, GMK Schwerpunktprogramm. Geomorphologische Detailkartierung in der Bundesrepublik Deutschland, edited by: Barsch, D., Fränzle, O., Leser, H., Liedtke, H., and Stäblein, G., Geo Center, Stuttgart, 1985b.
Metz, B.: Glaziale Formen und Formungsprozesse im Schwarzwald, in: Schwarzwald und Oberrheintiefland, edited by: Mäckel, R., and Metz, B., Freiburger Geographische Hefte, 36, Institut für Physische Geographie der Albert-Ludwigs-Universität Freiburg i. B, Freiburg i. Br., 51–74, 1997.
Metz, B. and Saurer, H.: Geomorphologie und Landschaftsentwicklung, in: Der Feldberg: subalpine Insel im Schwarzwald, edited by: Regierungspräsidium Freiburg, Jan Thorbecke Verlag der Schwabenverlag AG, Ostfildern, 14–62, 2012.
Monegato, G., Scardia, G., Hajdas, I., Rizzini, F., and Piccin, A.: The Alpine LGM in the boreal ice-sheets game, Sci. Rep.-UK, 7, 2078, https://doi.org/10.1038/s41598-017-02148-7, 2017.
Moran, A. P., Ivy-Ochs, S., Schuh, M., Christl, M., and Kerschner, H.: Evidence of central Alpine glacier advances during the Younger Dryas–early Holocene transition period, Boreas, 45, 398–410, https://doi.org/10.1111/bor.12170, 2016.
Pearce, D. M., Ely, J. C., Barr, I. D., and Boston, C. M.: Glacier reconstruction, in: Geomorphological Techniques, edited by: Cook, S., Clarke, L., and Nield, J., British Society for Geomorphology, London, 2017.
Pellitero, R., Rea, B. R., Spagnolo, M., Bakke, J., Ivy-Ochs, S., Frew, C. R., Hughes, P., Ribolini, A., Lukas, S., and Renssen, H.: GlaRe, a GIS tool to reconstruct the 3D surface of palaeoglaciers, Comput. Geosci., 94, 77–85, https://doi.org/10.1016/j.cageo.2016.06.008, 2016.
Piotrowska, N., Blaauw, M., Mauquoy, D., and Chambers, F. M.: Constructing deposition chronologies for peat deposits using radiocarbon dating, Mires Peat, 7, 10, 2011.
Protin, M., Schimmelpfennig, I., Mugnier, J.-L., Ravanel, L., Le Roy, M., Deline, P., Favier, V., Buoncristiani, J.-F., Aumaître, G., Bourlès, D. L., and Keddadouche, K.: Climatic reconstruction for the Younger Dryas/Early Holocene transition and the Little Ice Age based on paleo-extents of Argentière glacier (French Alps), Quaternary Sci. Rev., 221, 105863, https://doi.org/10.1016/j.quascirev.2019.105863, 2019.
Rades, E. F., Sohbati, R., Lüthgens, C., Jain, M., and Murray, A. S.: First luminescence-depth profiles from boulders from moraine deposits: Insights into glaciation chronology and transport dynamics in Malta valley, Austria, Rad. Meas., 120, 281–289, https://doi.org/10.1016/j.radmeas.2018.08.011, 2018.
Rahm, G.: Die Vergletscherung des Belchengebietes (Südschwarzwald) zur Würmeiszeit, E&G Quaternary Sci. J., 37, 31–40, https://doi.org/10.3285/eg.37.1.03, 1987.
Ramsay, A. C.: On the Glacial Origin of certain Lakes in Switzerland, the Black Forest, Great Britain, Sweden, North America, and elsewhere, Quaterly Journal of the Geological Society of London, 18, 185–204, 1862.
Reimer, P. J., Bard, E., Bayliss, A., Beck, J. W., Blackwell, P. G., Ramsey, C. B., Buck, C. E., Cheng, H., Edwards, R. L., Friedrich, M., Grootes, P. M., Guilderson, T. P., Haflidason, H., Hajdas, I., Hatté, C., Heaton, T. J., Hoffmann, D. L., Hogg, A. G., Hughen, K. A., Kaiser, K. F., Kromer, B., Manning, S. W., Niu, M., Reimer, R. W., Richards, D. A., Scott, E. M., Southon, J. R., Staff, R. A., Turney, C. S. M., and van der Plicht, J.: IntCal13 and Marine13 Radiocarbon Age Calibration Curves 0–50,000 Years cal BP, Radiocarbon, 55, 1869–1887, https://doi.org/10.2458/azu_js_rc.55.16947, 2013.
Reznichenko, N. V., Davies, T. R. H., and Winkler, S.: Revised palaeoclimatic significance of Mueller Glacier moraines, Southern Alps, New Zealand, Earth Surf. Process. Landforms, 41, 196–207, https://doi.org/10.1002/esp.3848, 2016.
Rixhon, G., Briant, R. M., Cordier, S., Duval, M., Jones, A., and Scholz, D.: Revealing the pace of river landscape evolution during the Quaternary: recent developments in numerical dating methods, Quaternary Sci. Rev., 166, 91–113, https://doi.org/10.1016/j.quascirev.2016.08.016, 2017.
Sawatzki, G.: Geologische Karte 1:25 000 von Baden-Württemberg. Erläuterungen zu Blatt 8124 St. Blasien, Landesvermessungsamt Baden-Württemberg, Stuttgart, 146 pp., 1992.
Schoeneich, P.: Comparaison des systèmes de légende français, allemand et suisse, in: Cartographie géomorphologique – Cartographie des risques. Actes de la Réunion annuelle de la Société Suisse de Géomorphologie, 19 au 21 juin 1992 aux Diablerets et à Randa, edited by: Schoeneich, P. and Reynard, E., Travaux et recherches, 9, Institut de Géographie Lausanne, Lausanne, 15–24, 1993.
Schreiner, A.: Geologische Karte von Freiburg i. Br. und Umgebung 1:50 000, 1st edn., Geologisches Landesamt Baden-Württemberg, Freiburg i. Br., 1977a.
Schreiner, A.: Quartär, in: Erläuterungen zur Geologischen Karte von Freiburg i. Br. und Umgebung 1:50 000, 1st edn., edited by: Geologisches Landesamt Baden-Württemberg, Landesvermessungsamt Baden-Württemberg, Stuttgart, 174–198, 1977b.
Schreiner, A.: Quartär, in: Geologische Karte von Baden-Württemberg 1:25 000. Erläuterungen zu Blatt 8114 Feldberg, 1st edn., edited by: Geologisches Landesamt Baden-Württemberg, Landesvermessungsamt Baden-Württemberg, Stuttgart, 67–95, 1981a.
Schreiner, A.: Quartär, in: Erläuterungen zur Geologischen Karte von Freiburg im Breisgau und Umgebung 1:50 000, 2nd edn., edited by: Geologisches Landesamt Baden-Württemberg, Landesvermessungsamt Baden-Württemberg, Stuttgart, 174–199, 1981b.
Schreiner, A.: Quartär, in: Geologische Karte 1:25 000 von Baden-Württemberg. Erläuterungen zu Blatt 8114 Feldberg, 2nd edn., edited by: Geologisches Landesamt Baden-Württemberg, Landesvermessungsamt Baden-Württemberg, Stuttgart, 67–95, 1990.
Schreiner, A.: Quartär, in: Geologische Karte von Baden-Württemberg 1:50 000. Freiburg i. Br. und Umgebung. Erläuterungen, 3rd edn., Geologisches Landesamt Baden-Württemberg, Freiburg i. Br., 174–199, 1996a.
Schreiner, A.: Geologische Karte von Baden-Württemberg 1:50 000. Freiburg i. Br. und Umgebung, 3rd edn., Geologisches Landesamt Baden-Württemberg, Freiburg i. Br., 1996b.
Schreiner, A.: Quartär, in: Geologische Karte von Baden-Württemberg 1:25 000. Erläuterungen zum Blatt 8014 Hinterzarten, edited by: Wimmenauer, W. and Schreiner, A., Landesvermessungsamt Baden-Württemberg, Stuttgart, 97–141, 1999.
Schreiner, A.: Quartär, in: Geologische Karte von Baden-Württemberg 1:25 000. Erläuterungen zu Blatt 8113 Todtnau, edited by: Landesamt für Geologie, Rohstoffe und Bergbau (LGRB), Freiburg i. Br., 42–92, 2011.
Schreiner, A. and Wimmenauer, W.: Geologische Karte von Baden-Württemberg 1:25 000. Blatt 8014 Hinterzarten, Landesamt für Geologie, Rohstoffe und Bergbau, Freiburg i. Br., 1999.
Schrepfer, H.: Zur Kenntnis der Eiszeit im Wutachgebiet, Mitteilungen des Bad. Landesvereins für Naturkunde und Naturschutz in Freiburg i. Br., 1, 469–473, 1925.
Schrepfer, H.: Glazialprobleme im westlichen Hochschwarzwald, Ber. Naturf. Ges. Freiburg i. Br., 31, 161–210, 1931.
Steinmann, G.: Die Spuren der letzten Eiszeit im hohen Schwarzwalde, in: Freiburger Universitätsprogramm zum siebzigsten Geburtstag seiner kgl. Hoheit des Großherzogs Friedrich, Akademische Verlagsbuchhandlung von J. C. B. Mohr (Paul Siebeck), Freiburg and Leipzig, 188–226, 1896.
Steinmann, G.: Die Bildungen der letzten Eiszeit im Bereiche des alten Wutachgebiets, Ber. d. Oberrhein. Geol. Ver., 35, 1–8, 1902.
Steinmann, G.: Die Eiszeit und der vorgeschichtliche Mensch, Aus Natur und Geisteswelt, 302, B. G. Teubner, Leipzig, 1910.
Stokes, C. R., Tarasov, L., Blomdin, R., Cronin, T. M., Fisher, T. G., Gyllencreutz, R., Hättestrand, C., Heyman, J., Hindmarsh, R. C. A., Hughes, A. L. C., Jakobsson, M., Kirchner, N., Livingstone, S. J., Margold, M., Murton, J. B., Noormets, R., Peltier, W. R., Peteet, D. M., Piper, D. J. W., Preusser, F., Renssen, H., Roberts, D. H., Roche, D. M., Saint-Ange, F., Stroeven, A. P., and Teller, J. T.: On the reconstruction of palaeo-ice sheets: Recent advances and future challenges, Quaternary Sci. Rev., 125, 15–49, https://doi.org/10.1016/j.quascirev.2015.07.016, 2015.
Telford, R. J., Heegaard, E., and Birks, H. J. B.: The intercept is a poor estimate of a calibrated radiocarbon age, Holocene, 14, 296–298, https://doi.org/10.1191/0959683604hl707fa, 2004.
Törnqvist, T. E., de Jong, A. F. M., Oosterbaan, W. A., and Van Der Borg, K.: Accurate Dating of Organic Deposits by AMS 14C Measurement of Macrofossils, Radiocarbon, 34, 566–577, https://doi.org/10.1017/S0033822200063840, 1992.
van den Bogaard, P. and Schmincke, H.-U.: Laacher See Tephra: A widespread isochronous late Quaternary tephra layer in central and northern Europe, GSA Bulletin, 96, 1554–1571, https://doi.org/10.1130/0016-7606(1985)96<1554:LSTAWI>2.0.CO;2, 1985.
Wagner, K.: Geographic Information Systems and Glacial Environments, in: Past Glacial Environments (Second Edition), edited by: Menzies, J. and van der Meer, J. J. M., Elsevier, 503–536, 2018.
Westoby, M. J., Brasington, J., Glasser, N. F., Hambrey, M. J., and Reynolds, J. M.: “Structure-from-Motion” photogrammetry: A low-cost, effective tool for geoscience applications, Geomorphology, 179, 300–314, https://doi.org/10.1016/j.geomorph.2012.08.021, 2012.
Wimmenauer, W. and Hüttner, R.: Geologische Karte von Baden-Württemberg 1:25 000. Blatt 8013 Freiburg, Landesvermessungsamt Baden-Württemberg, Stuttgart, 1968.
Wimmenauer, W., Liehl, E., and Schreiner, A.: Geologische Karte von Baden-Württemberg 1:25 000. Blatt 8114 Feldberg, 2nd edn., Landesvermessungsamt Baden-Württemberg, Stuttgart, 1990.
Winkler, S.: Investigating Holocene mountain glaciations: a plea for the supremacy of glacial geomorphology when reconstructing glacier chronologies, Erdkunde, 72, 215–234, https://www.doi.org/10.3112/erdkunde.2018.03.04, 2018.
Winkler, S. and Hagedorn, H.: Lateralmoränen – Morphologie, Genese und Beziehung zu Gletscherstandsschwankungen (Beispiele aus Ostalpen und West-/Zentralnorwegen), Z. Geomorph. Supp., 113, 69–84, 1999.
Zienert, A.: Die Würm-Vereisung und ihre Rückzugsstadien im Westteil des Hochschwarzwaldes, Z. Geomorphol., 17, 359–366, 1973.
- How to cite
- Abstract
- Kurzfassung
- Introduction
- Study area
- Late Pleistocene glacier fluctuations in the southern Black Forest
- Methods
- Results and interpretation
- Discussion
- Conclusions
- Data availability
- Author contributions
- Competing interests
- Acknowledgements
- Financial support
- Review statement
- References
- Supplement
- How to cite
- Abstract
- Kurzfassung
- Introduction
- Study area
- Late Pleistocene glacier fluctuations in the southern Black Forest
- Methods
- Results and interpretation
- Discussion
- Conclusions
- Data availability
- Author contributions
- Competing interests
- Acknowledgements
- Financial support
- Review statement
- References
- Supplement