the Creative Commons Attribution 4.0 License.
the Creative Commons Attribution 4.0 License.
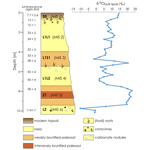
18O analyses of bulk lipids as novel paleoclimate tool in loess research – a pilot study
Jakob Labahn
Lucas Bittner
Philip Hirschmann
Christopher-Bastian Roettig
Diana Burghardt
Bruno Glaser
Slobodan B. Marković
Michael Zech
Labahn, J., Bittner, L., Hirschmann, P., Roettig, C.-B., Burghardt, D., Glaser, B., Marković, S. B., and Zech, M.: 18O analyses of bulk lipids as novel paleoclimate tool in loess research – a pilot study, E&G Quaternary Sci. J., 71, 83–90, https://doi.org/10.5194/egqsj-71-83-2022, 2022.
The analysis of the stable oxygen isotopes 18O and 16O has revolutionized paleoclimate research since the middle of the last century. Particularly, δ18O of ice cores from Greenland and Antarctica is used as a paleotemperature proxy, and δ18O of deep-sea sediments is used as a proxy for global ice volume. Important terrestrial archives to which δ18O as a paleoclimate proxy is successfully applied are speleothems, lake sediments, or tree rings. By contrast, δ18O applications to loess–paleosol sequences (LPSs) are scarce. Here we present a first continuous δ18O record (n=50) for the LPS Crvenka in Serbia, southeastern Europe, spanning the last glacial–interglacial cycle (since 145 ka). From a methodological point of view, we took advantage of a recently proposed paleoclimate/paleohydrological proxy based on bulk δ18O analyses of plant-derived lipids. The Crvenka δ18Obulk lipid values range between −10.2 ‰ and +23.0 ‰ and are systematically more positive in the interglacial and interstadial (paleo-)soils corresponding to marine oxygen-isotope stage (MIS) 1, 3, and 5, compared to the loess layers (MIS 2, 4, and 6). Our Crvenka δ18Obulk lipid record provides no evidence for the occurrence of interstadials and stadials comparable to the Dansgaard–Oeschger events known from the Greenland δ18Oice core records. Concerning the interpretation of our Crvenka δ18Obulk lipid record, plant-derived lipids such as fatty acids and alcohols are certainly strongly influenced by climatic factors such as temperature (via δ18Oprecipitation) and relative air humidity (via 18O enrichment of leaf water due to evapotranspiration). However, pool effects in the form of non-water-correlated lipids such as sterols or the input of root-derived lipids need to be considered, too. Similarly, the input of soil-microbial lipids and oxygen exchange reactions represent uncertainties challenging quantitative paleoclimate/paleohydrological reconstructions based on δ18Obulk lipid analyses from LPSs.
Die Analyse der stabilen Sauerstoffisotope 18O und 16O hat die Paläoklimaforschung seit Mitte des letzten Jahrhunderts revolutioniert. Insbesondere wird δ18O von Eisbohrkernen aus Grönland und der Antarktis als Paläo-Temperaturproxy sowie δ18O von Tiefseesedimenten als Proxy für das globale Eisvolumen verwendet. Wenngleich sich in terrestrischen Archiven, wie Speläothemen, Seesedimenten oder Baumringen, paläoklimatische Rekonstruktionen unter der Anwendung von δ18O als Proxy bewährt haben, wurden solche Analysen bislang in Löß-Paläobodensequenzen (LPS) selten durchgeführt. In dieser Studie präsentieren wir einen ersten kontinuierlichen δ18O Datensatz (n=50) für die LPS Crvenka in Serbien, der den letzten Glazial-Interglazial-Zyklus (∼ 145 ka) abdeckt. Die δ18O-Werte basieren auf der Analyse von pflanzlichen Lipiden, deren Anwendung als paläoklimatischer/hydrologischer Proxy vor Kurzem vorgeschlagen wurde.
Die δ18Obulk−lipid-Werte von Crvenka liegen zwischen −10.2 ‰ und +23.0 ‰ und sind in den interglazialen und interstadialen (Paläo-)Böden, die den marinen Sauerstoff-Isotopenstufen (MIS) 1, 3 und 5 entsprechen, systematisch positiver als in den Lößlagen (MIS 2, 4 und 6). Sie liefern keine Hinweise für das Auftreten von Interstadialen und Stadialen, die mit den aus den grönländischen δ18Oice−core bekannten Dansgaard-Oeschger-Ereignissen vergleichbar wären. In Bezug auf die Interpretation der δ18Obulk−lipid-Werte gilt es zu berücksichtigen, dass die Isotopie pflanzlicher Lipide, wie z.B. von Fettsäuren und Alkoholen, stark durch die Klimafaktoren Temperatur (über δ18O-Niederschlag) und relativer Luftfeuchtigkeit (über die 18O-Anreicherung des Blattwassers aufgrund von Evapotranspiration) beeinflusst werden. Weiter zu beachtende Faktoren stellen Einträge von Sterolen sowie von generell wurzel-bürtigen Lipiden dar (Pool-Effekte). In ähnlicher Weise bergen der Einfluss von bodenmikrobiellen Lipiden und Sauerstoffaustauschreaktionen Unsicherheiten, die quantitative paläoklimatische/hydrologische Rekonstruktionen auf der Grundlage von δ18Obulk−lipid-Analysen aus LPS erschweren können.
- Article
(5503 KB) -
Supplement
(109 KB) - BibTeX
- EndNote
The analysis of the stable oxygen isotopes 18O and 16O has revolutionized paleoclimate research since the middle of the last century. Particularly, the oxygen isotopic composition δ18O of ice cores from Greenland and Antarctica is used as a paleotemperature proxy (e.g., NGRIP members, 2004), and δ18O of deep-sea sediments is used as a proxy for global ice volume (e.g., Lisiecki and Raymo, 2005). Important terrestrial archives to which δ18O as a paleoclimate proxy is currently successfully applied are speleothems (e.g., Spötl et al., 2006), lake sediments (e.g., Bittner et al., 2021), or tree rings (e.g., Roden et al., 2000). In all these archives, the δ18O signal of paleoprecipitation (δ18Oprecipitation) plays a major role, for which four effects can be highlighted. (1) The “temperature effect” describes that the colder the temperature is, the more negative δ18Oprecipitation becomes. (2) The “amount effect” describes that δ18Oprecipitation becomes more negative with increasing precipitation amount. (3) The “altitude effect” describes that δ18Oprecipitation becomes more negative with increasing altitude. (4) The “source effect” explains that air masses derived from different moisture sources can have distinct and variable δ18Oprecipitation values (e.g., Dansgaard, 1964; Lachniet, 2009; Lemma et al., 2021).
In contrast to the aforementioned terrestrial archives, δ18O applications to loess–paleosol sequences (LPSs) are still scarce, although these represent unique and widespread occurring paleoenvironmental archives. For instance, Pustovoytov and Terhorst (2004) examined calcified root cells in an LPS using δ13C and δ18O analyses to determine climatic conditions based on the signal obtained during post-sedimentary Holocene soil formation, and Prud'homme et al. (2016) used δ18O of earthworm calcite granules from an LPS to reconstruct paleotemperatures. Other approaches with respect to isotope analyses in loess archives have lately focused on the study of biomarkers (e.g., R. Zech et al., 2013; Schäfer et al., 2016; Häggi et al., 2019). These biomarkers are primarily compounds derived from plants, such as lipids or sugars (monosaccharides). For example, M. Zech et al. (2013) applied compound-specific δ18O analysis of plant-derived sugar biomarkers to a permafrost LPS from Siberia. Similar to compound-specific δ2H analyses of leaf-wax-derived n-alkane biomarkers (R. Zech et al., 2013), δ18O of sugar biomarkers has a great potential to serve as a paleoclimate proxy in sedimentary archives (Zech et al., 2014). However, this latter approach could not be adopted so far due to the considerable carbonate amounts in classical LPSs. To sum up, no continuous δ18O records could be established for classical LPSs up to now.
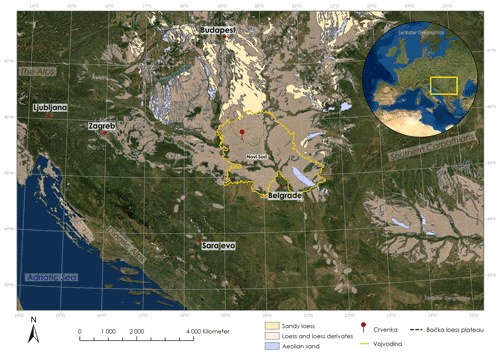
Figure 1Map of the Carpathian basin with loess deposits according to Lehmkuhl et al. (2021) and location of the Crvenka study area (created with ArcGIS Pro).
Recently, Silva et al. (2015) and Maxwell et al. (2018) proposed δ18O of plant-derived bulk lipids (δ18Obulk lipids) as a proxy for reconstructing ecosystem water balances. Lipids are important constituents of cuticular waxes and comprise, e.g., non-oxygen-bearing long-chain n alkanes as well as oxygen-bearing molecules such as long-chain alkanols and alkanoic acids. From an analytical point of view, total lipid extraction from plants, soils, or sediments for yielding bulk lipids is relatively simple and can be achieved, e.g., by Soxhlet, ultrasonic, or microwave extraction. Lipids are generally hydrophobic and thus not specifically prone to translocation by percolating water in soils. Moreover, they are relatively persistent against biodegradation and can thus be studied even over geological timescales in paleosols and sediments. According to our knowledge, δ18Obulk lipid analyses have hitherto not been applied to paleoenvironmental archives such as LPSs.
The aim of our pilot study presented here was therefore to test the applicability of δ18Obulk lipid analyses to LPSs. Given that abundant research, including numerical dating, has already been conducted there, we chose the late Middle Pleistocene–Holocene LPS Crvenka in Serbia (Marković et al., 2015; Stevens et al., 2011; R. Zech et al., 2013) and followed the following specific research questions and objectives:
-
How high are the bulk lipid contents in the LPS Crvenka, and are the extractable amounts sufficient for δ18Obulk lipid analyses?
-
In the case that a δ18Obulk lipid record can be established for the LPS Crvenka, does it show variations coinciding with the marine oxygen-isotope stage (MIS) or with interstadials known from Greenland δ18Oice core records?
-
Which factors need to be considered when interpreting δ18Obulk lipid records?
2.1 Study site and sampling
The LPS Crvenka (45∘39.750′ N, 19∘28.774′ E; 108 m a.s.l.) is located in the center of the Carpathian basin, southeastern Europe, in a brickyard exposure on the southwestern edge of the Bačka loess plateau in the Vojvodina region (see Fig. 1). According to the effective classification of Köppen, the current climate in the Carpathian basin is mainly classified as Cfb (i.e., C – temperate, f – without dry season, b – warm summers), but the regional climate can vary greatly due to its location in the border area between the Atlantic, continental, and Mediterranean climate zones. In January the average temperature is −0.1 ∘C, and in July it is 21.9 ∘C, while mean annual precipitation is 612 mm with a maximum in spring (Peel et al., 2007). We chose the LPS Crvenka (spanning about the last 145 kyr) for our δ18Obulk lipid pilot study due to previous detailed stratigraphic and pedologic descriptions, geochemical analyses, and numerical dating (Marković et al., 2015; Stevens et al., 2011; R. Zech et al., 2013). The chronostratigraphy can be summarized as follows: vertically, the profile extends over a depth of approximately 10 m (Fig. 2).
The oldest pale-yellow loess unit (“L2”) of the Crvenka LPS consists mainly of penultimate glacial silts and extends only over the lowest 100 cm of the profile. The overlying reddish-brown, clay-rich, and strongly brunified paleosol complex, with a thickness between 215–235 cm (“S1”), is correlated with MIS 5 and thus formed during the last interglacial. Above there is a loess package (L1) up to 8 m thick, which was deposited over the last glacial period. It is composed of two loess layers L1L1 (top) and L1L2 (bottom) separated by a weakly developed paleosol complex (“L1S1”). The lower light-yellow-grey L1L2 layer correlates with MIS 4 and is coarser-grained than the very porous L1L1 loess layer assigned to MIS 2. The interstadial, weakly developed paleosol complex (“L1S1”) corresponds roughly to MIS 3. The uppermost part of the profile consists of a black-to-reddish-brown Holocene soil (“S0”) that ranges in thickness from 50 to 80 cm and can be associated with MIS 1.
For our δ18Obulk lipid pilot study, we used 50 loess and paleosol samples that were taken by continuous sampling in ∼ 20 cm intervals by Zech et al. (2013) in 2009.
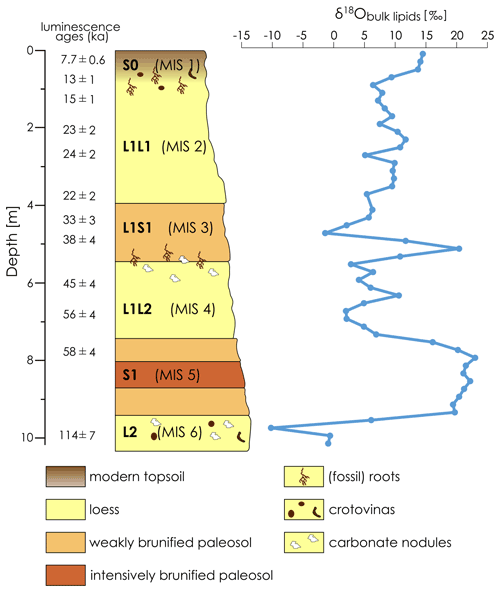
Figure 2Stratigraphy of the LPS Crvenka after R. Zech et al. (2013) and the δ18Obulk lipid record. Note that the luminescence ages of Stevens et al. (2011) are “preferred” ages and that a numerical age model could only be constructed for the last 50 to 60 kyr. Lower luminescence ages than expected for older sediments probably indicate post-depositional effects on the luminescence signal (Stevens et al., 2011).
2.2 Total lipid extraction and δ18Obulk lipid measurement
Total lipid extraction from about 20 g of sample material was performed using an ultrasonic bath for 15 min and 30 mL of DCM:MeOH (9:1) as a solvent. In preceding tests, we found that bulk lipid yields from loess–paleosol samples are much lower when, e.g., hexane is used as a solvent instead of DCM:MeOH (9:1) and that the δ18Obulk lipid results are less reproducible. The total lipid extracts were centrifuged at 2000 rpm for 15 min and were subsequently passed over pipette columns filled with glass fiber. The extraction and purification procedures were repeated two more times with 20 mL of solvent for each sample. After solvent reduction using rotary evaporation, the total lipid extracts were transferred into silver capsules with DCM. Bulk lipid yields were quantified by weighing the silver capsules before and after the transfer and solvent evaporation. The bulk δ18O measurements of the lipids were performed at the Institute of Groundwater Management of the Technische Universität (TU) Dresden using an EA IsoLink elemental analyzer coupled to a Delta V Plus IRMS (isotope ratio mass spectrometer; Thermo Fisher Scientific GmbH, Bremen, Germany). For calibration, the reference materials NBS 127, GISP, VSMOW2 (Vienna Standard Mean Ocean Water), CH3, and CH6 (all from the International Atomic Energy Agency, IAEA) were used. The standard deviation for replication measurements of these reference materials was on average 0.5 ‰ and never exceeded 1.2 ‰. All results are reported in the usual δ notation versus the Vienna Standard Mean Ocean Water (VSMOW).
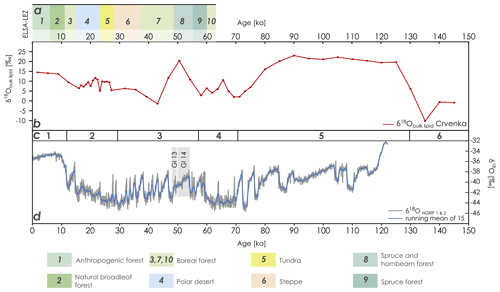
Figure 3Comparison of (a) the landscape evolution zones after Sirocko et al. (2016), (b) the Crvenka δ18Obulk lipid record (based on the MIS-based age–depth model of R. Zech et al., 2013), (c) the marine isotope stages after Lisiecki and Raymo (2005), and (d) the Greenland δ18O records (based on data of NGRIP members, 2004). GI 13 and 14 represent Greenland Interstadial 13 and 14, respectively. ELSA-LEZ: landscape evolution zones (LEZs) reconstructed from the Eifel Laminated Sediment Archive (ELSA). NGRIP: North Greenland Ice Core Project.
3.1 Bulk lipid contents and comparison of the Crvenka δ18Obulk lipid record with marine oxygen-isotope stages and Greenland δ18O records
The bulk lipid contents of the LPS Crvenka range from 0.023 to 0.140 mg g−1, and the absolute amounts range between 0.5 and 2.9 mg. These amounts allowed us to perform δ18Obulk lipid measurements for all samples: the δ18Obulk lipid values range between −10.2 ‰ and +23.0 ‰ and are systematically more positive in the interglacial (paleo-)soils compared to the loess layers (see Figs. 2 and 3). The modern Holocene soil, roughly corresponding with MIS 1, is characterized by δ18Obulk lipid values around +14 ‰, and the intensively brunified last interglacial paleosol complex V S1 coinciding with MIS 5 is characterized by δ18Obulk lipid values 20 ‰. MIS 5 substages a–e are generally not differentiated by pedogenetic or analytical features in the Serbian LPSs (Marković et al., 2015; R. Zech et al., 2013). It is therefore not surprising that our δ18Obulk lipid record does not indicate these substages either. The weakly developed interstadial paleosol complex L1S1, coinciding with MIS 3, shows a striking large fluctuation with a minimum δ18Obulk lipid value of −1.4 ‰ in the middle and a maximum δ18Obulk lipid value of +20.4 ‰ in the lower part. The δ18Obulk lipid values of the last glacial loess layers L1L1 and L1L2 (coinciding with MIS 2 and 4, respectively) range between +2.0 ‰ and +11.7 ‰. Loess layer L2, representing the penultimate glacial and coinciding with MIS 6, is characterized by the most negative δ18Obulk lipid values of up to −10.2 ‰.
In order to enable the comparison of our Crvenka δ18Obulk lipid record with Greenland δ18Oice core records, we adopted the MIS-scale age–depth model of R. Zech et al. (2013). Although the LPS Crvenka is one of the best luminescence-dated LPSs in the Carpathian basin (Stevens et al., 2011), we refrain from applying a luminescence-based age–depth model (see Fig. 2 for preferred ages according to Stevens et al., 2011) due to likely age underestimations. Figure 3 shows that the Crvenka δ18Obulk lipid record only partly resembles the Greenland δ18ONGRIP record. Particularly the Holocene and the time period from around 80 to 130 ka, including the Eemian interglacial, are characterized by more positive δ18Obulk lipid values. While one striking δ18Obulk lipid maximum occurs around 50 ka, our Crvenka δ18Obulk lipid record does not reflect the succession of stadials and interstadials known from the Greenland ice core records, i.e., the famous Dansgaard–Oeschger events. When comparing our southeastern European δ18Obulk lipid record with the mid-European landscape evolution zones (LEZs) identified by Sirocko et al. (2016) based on pollen and total carbon analyses from laminated Eifel maar sediments, LEZ 8 ranging from 49 to 55 ka is characterized by the unexpected dominance of thermophilous tree taxa (see Fig. 3a). This suggests that Greenland Interstadial 13 and 14 (see Fig. 3d; the latter is often referred to as the Glinde interstadial) were the warmest periods of MIS 3. However, the chronological resolution of MIS 3 in the LPS Crvenka is quite limited. Thus, similar to unresolved MIS 5 substages a–e, the absence of stadial–interstadial successions in our δ18Obulk lipid record in the LPS Crvenka does not necessarily mean that these climate variations did not occur in southeastern Europe. However, interestingly around this period very high δ18Obulk lipid values are found in our LPS as well.
The last glacial maximum (around 25 to 20 ka) is not characterized, as one might expect, by particularly negative δ18Obulk lipid values. Last but not least, the amplitude of the Crvenka δ18Obulk lipid values cover around double the amplitude compared to the δ18ONGRIP amplitude (Fig. 3). Possible explanations for these two findings, as well as all factors needing consideration when interpreting δ18Obulk lipid records from LPSs, are discussed in the following section.
3.2 Paleoclimatic interpretation of the Crvenka δ18Obulk lipid record
δ18O analyses of cellulose are a widely applied tool in paleoclimate research. Yet, its application to classical LPSs is still hampered by analytical challenges mainly associated with low cellulose and high carbonate contents, hindering the acid-hydrolytic extraction of (hemi-)cellulose-derived sugar biomarkers. In their groundbreaking study “Beyond the cellulose: Oxygen isotope composition of plant lipids as a proxy for terrestrial water balance”, Silva et al. (2015) observed a strong linear relationship between δ18Obulk lipid and δ18Ocellulose. This corroborates that δ18O values of many plant-derived lipids such as organic acids, carbonyls, alcohols, and esters are water-correlated, although this does not hold true for plant-derived sterols that contain atmospheric-derived oxygen (Schmidt et al., 2001). Accordingly, oxygen from fatty acids or acyl-derived alcohols is about +19 ‰ more positive compared to water. Such systematic offsets are usually described as biosynthetic fractionation factors and are certainly one important factor needing consideration when interpreting δ18Obulk lipid records from LPSs (Fig. 4).
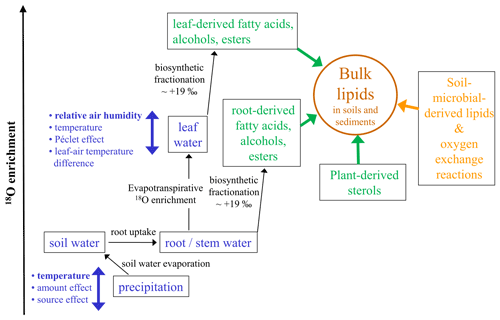
Figure 4Conceptual diagram illustrating the major factors influencing the oxygen isotopic composition of bulk lipids (δ18Obulk lipid) in soils and sediments (modified after Zech et al., 2014). Apart from climatic factors (blue colors) with, e.g., relative air humidity controlling the isotopic enrichment of leaf water by evapotranspiration, “pool effects” (green colors) particularly by the admixture of sterols and root-derived lipids need consideration. Last but not least, the buildup of soil-microbial-derived lipids is also a kind of a pool effect introducing uncertainty, too, and a part of the oxygen bound in lipids is prone to exchange reactions with soil water.
Concerning plant water in which the lipids are biosynthesized, it is generally accepted that the uptake of soil water by plants is not associated with discernible isotopic fractionation. Thus, in cases where significant evaporative enrichment of soil water can be excluded, δ18O of root and stem water reflects the mean isotopic composition of local precipitation. In high latitudes δ18Oprecipitation is primarily temperature-controlled. Hence, it is likely that the temperature effect on δ18Oprecipitation is one important factor controlling the glacial–interglacial variability of our Crvenka δ18Obulk lipid record, with more positive/negative values during the warm interglacials/cold glacials (Figs. 2, 3, and 4). However, on the one hand, apart from the temperature effect neither the amount effect nor the source effect can be excluded as possible factors that had influenced δ18Oprecipitation in the Crvenka study area during the past. Unfortunately, their quantitative assessment has been rather challenging so far. On the other hand, δ18Oprecipitation changes alone can certainly not explain the δ18Obulk lipid amplitude of our Crvenka record, ranging between −10.2 ‰ and +23.0 ‰. Indeed, leaf-(wax-)derived lipids do not reflect the isotopic composition directly of precipitation but instead of isotopically enriched leaf water (Fig. 4). This is well known for δ2H of leaf-wax-derived n alkanes, where due to evaporation leaf water can be isotopically enriched by up to ∼ 50 ‰ compared to xylem water (e.g., Zech et al., 2015). In the case of δ18O, leaf water enrichment of up to ∼ 25 ‰ was for instance reported by Zech et al. (2014). The main controlling factor for this enrichment is relative air humidity (RH), with lower RH values resulting in stronger 2H and 18O enrichment of leaf water. Further minor influencing factors are temperature, leaf–air temperature differences, and the so-called Péclet effect. The latter describes, in simplified terms, the mixing of water from the xylem and from evaporation sites within plant leaves. Water that flows to evaporation sites by transpiration is enriched in at the evaporation sites by back diffusion of the enriched water (cf. Farquhar and Lloyd, 1993; Ferrio et al., 2012).
Next to the climatic factors temperature and relative air humidity, also “pool effects” need to be considered when interpreting δ18Obulk lipid values in plants and soils. This is comparable to bulk δ2H results of soils and sediments, where pool effects exert influence, too (cf. Zech et al., 2015). On the one hand, as mentioned above δ18O of plant-derived sterols is not water-correlated, and thus sterols dilute the climate signal of the leaf-derived fatty acids and alcohols (Fig. 4). On the other hand, root and stem input of fatty acids and alcohols likely introduces a δ18O signal that does not carry the 18O enrichment of leaf water (Fig. 4). For instance, in the MIS 2 part of our Crvenka δ18Obulk lipid record, one might have expected more negative values in the case of a mere climate control (Figs. 2 and 3). Hence, postsedimentary Holocene root input of oxygen-bearing lipids into the L1L1 layer could possibly explain the absence of a more pronounced δ18Obulk lipid minimum. Moreover, bulk lipids extracted from soils and sediments not only are plant-derived but also contain soil-microbial-derived lipids. Variable and unknown contributions of such soil-microbial-derived lipids introduce another source of uncertainty that is difficult to quantify based on the current state of knowledge. In addition, a part of the oxygen bound in lipids is prone to exchange reactions with soil water (Fig. 4). For instance, using laboratory incubation studies with isotopically enriched water, Maxwell et al. (2018) found that 22 % of oxygen from bulk soil lipid extracts is exchangeable.
Our pilot study suggests that δ18O analyses of bulk lipids (δ18Obulk lipid) can successfully be applied to loess–paleosol sequences, since absolute bulk lipid yields for the LPS Crvenka ranged between 0.5 and 2.9 mg per 20 g of sample material. Our Crvenka δ18Obulk lipid record reflects the alternation between loess layers and (paleo-)soils (more negative and more positive δ18Obulk lipid values, respectively) and thus marine oxygen-isotope stage (MIS) 1 to 6. Yet, this does not involve MIS 5 substages a–e, which are not differentiated in the LPS Crvenka. Similarly, our Crvenka δ18Obulk lipid record does not provide evidence for the occurrence of interstadials and stadials comparable to the Dansgaard–Oeschger events known from the Greenland δ18Oice core records. This will require further and higher-resolution data in combination with more robust age models in the future.
Concerning the interpretation of our Crvenka δ18Obulk lipid record, plant-derived lipids such as fatty acids and alcohols are certainly strongly influenced by climatic factors such as temperature (via δ18Oprecipitation) and relative air humidity (via 18O enrichment of leaf water due to evapotranspiration). However, also pool effects in the form of non-water-correlated lipids such as sterols or the input of root-derived lipids should not be overlooked. Furthermore, also the input of soil-microbial lipids and oxygen exchange reactions represent uncertainties that are challenging quantitative paleoclimate/paleohydrological reconstructions based on δ18Obulk lipid analyses.
Underlying data can be found in the Supplement.
The supplement related to this article is available online at: https://doi.org/10.5194/egqsj-71-83-2022-supplement.
MZ developed the project idea in consultation with LB, CBR, and JL. The analyzed samples were taken during the fieldwork by RZ in cooperation with MZ. The laboratory work was mainly done by PH and DB with the participation of JL, who prepared the manuscript. SBM and BG, as well as all other co-authors, participated in the interpretation of the results and contributed to the completion of the manuscript.
The contact author has declared that neither they nor their co-authors have any competing interests.
Publisher’s note: Copernicus Publications remains neutral with regard to jurisdictional claims in published maps and institutional affiliations.
This article is part of the special issue “Quaternary research from and inspired by the first virtual DEUQUA conference”. It is a result of the vDEUQUA2021 online conference in September/October 2021.
We kindly thank Gerhard Gebauer and his team from the Laboratory of Isotope Biogeochemistry of the BayCEER at the University of Bayreuth as well as Tobias Krause (Institute of Soil Science and Site Ecology, TU Dresden), Erik Michalowski and Amelie Demmer (both Institute of Geography, TU Dresden), Doreen Degenhardt and Patricia Stock (both Institute of Groundwater Management, TU Dresden), and Marianne Zech for their support of laboratory work and isotope analyses. Special thanks go to Hans von Suchodoletz for his editorial handling, as well as to two anonymous reviewers for the valuable suggestions and their constructive reviews.
This paper is dedicated to Ludwig Zöller and Dominik Faust. Ludwig Zöller was born in 1953 and held the Chair of Geomorphology at the University of Bayreuth from 2002 until his retirement in 2018. Dominik Faust was born in 1954 and held the Chair of Physical Geography at the Technische Universität Dresden from 2000 until his retirement in 2020. Ludwig Zöller supervised the dissertation and habilitation of Michael Zech. Both Ludwig Zöller and Dominik Faust were important mentors of Michael Zech and opened the door towards loess research for Michael Zech and his team with their methodological expertise on biomarkers and stable isotopes.
This research has been supported by the Deutsche Forschungsgemeinschaft (grant nos. DFG ZE 844/14-1 and DFG FA 239/26-1).
This open-access publication was funded by the Technische Universität Dresden (TUD).
This paper was edited by Hans von Suchodoletz and reviewed by two anonymous referees.
Bittner, L., Gil-Romera, G., Grady, D., Lamb, H., Lorenz, E., Weiner, M., Meyer, H., Bromm, T., Glaser, B., and Zech, M.: The Holocene lake-evaporation history of the afro-alpine Lake Garba Guracha in the Bale Mountains, Ethiopia, based on δ18O records of sugar biomarker and diatoms, Quaternary Res., 105, 23–36, https://doi.org/10.1017/qua.2021.26, 2021.
Dansgaard, W.: Stable isotopes in precipitation, Tellus, 16, 436–468, https://doi.org/10.1111/j.2153-3490.1964.tb00181.x, 1964.
Farquhar, G. D. and Lloyd, J.: 5 – Carbon and Oxygen Isotope Effects in the Exchange of Carbon Dioxide between Terrestrial Plants and the Atmosphere, in: Stable Isotopes and Plant Carbon-water Relations, edited by: Ehleringer, J. R., Hall, A. E., and Farquhar, G. D., Academic Press, San Diego, 47–70, https://doi.org/10.1016/B978-0-08-091801-3.50011-8, 1993.
Ferrio, J. P., Pou, A., Florez-Sarasa, I., Gessler, A., Kodama, N., Flexas, J., and Ribas-Carbo, M.: The Péclet effect on leaf water enrichment correlates with leaf hydraulic conductance and mesophyll conductance for CO2, Plant, Cell Environ., 35, 611–625, https://doi.org/10.1111/j.1365-3040.2011.02440.x, 2012.
Häggi, C., Eglinton, T. I., Zech, W., Sosin, P., and Zech, R.: A 250 ka leaf-wax δD record from a loess section in Darai Kalon, Southern Tajikistan, Quaternary Sci. Rev., 208, 118–128, https://doi.org/10.1016/j.quascirev.2019.01.019, 2019.
Lachniet, M. S.: Climatic and environmental controls on speleothem oxygen-isotope values, Quaternary Sci. Rev., 28, 412–432, https://doi.org/10.1016/j.quascirev.2008.10.021, 2009.
Lehmkuhl, F., Nett, J. J., Pötter, S., Schulte, P., Sprafke, T., Jary, Z., Antoine, P., Wacha, L., Wolf, D., Zerboni, A., Hošek, J., Marković, S. B., Obreht, I., Sümegi, P., Veres, D., Zeeden, C., Boemke, B., Schaubert, V., Viehweger, J., and Hambach, U.: Loess landscapes of Europe – Mapping, geomorphology, and zonal differentiation, Earth-Sci. Rev., 215, 103496, https://doi.org/10.1016/j.earscirev.2020.103496, 2021.
Lemma, B., Bittner, L., Glaser, B., Kebede, S., Nemomissa, S., Zech, W., and Zech, M.: δ2Hn−alkane and δ18Osugar biomarker proxies from leaves and topsoils of the Bale Mountains, Ethiopia, and implications for paleoclimate reconstructions, Biogeochem., 153, https://doi.org/10.1007/s10533-021-00773-z, 2021.
Lisiecki, L. E. and Raymo, M. E.: A Pliocene-Pleistocene stack of 57 globally distributed benthic δ18O records, Paleoceanography, 20, https://doi.org/10.1029/2004PA001071, 2005.
Marković, S., Stevens, T., Kukla, G., Hambach, U., Fitzsimmons, K., Gibbard, P., Buggle, B., Zech, M., Guo, Z., Hao, Q., Wu, H., O'Hara-Dhand, K., Smalley, I., Újvári, G., Sümegi, P., Timar-Gabor, A., Veres, D., Sirocko, F., Vasiljevic, D., Vidojko, J., Zdzislaw, J., Anderss, S., Lehmkuhl, F., Kovács, J., and Svirčev, Z.: Danube loess stratigraphy – Towards a pan-European loess stratigraphic model, Earth-Sci. Rev., 148, 228–258, https://doi.org/10.1016/j.earscirev.2015.06.005, 2015.
Maxwell, T. M., Silva, L. C. R., and Horwath, W. R.: Predictable Oxygen Isotope Exchange Between Plant Lipids and Environmental Water: Implications for Ecosystem Water Balance Reconstruction, J. Geophys. Res.-Biogeosc., 123, 2941–2954, https://doi.org/10.1029/2018JG004553, 2018.
NGRIP members: High-resolution record of Northern Hemisphere climate extending into the last interglacial period, Nature, 431, 147–151, https://doi.org/10.1038/nature02805, 2004.
Peel, M. C., Finlayson, B. L., and McMahon, T. A.: Updated world map of the Köppen-Geiger climate classification, Hydrol. Earth Syst. Sci., 11, 1633–1644, https://doi.org/10.5194/hess-11-1633-2007, 2007.
Prud'homme, C., Lécuyer, C., Antoine, P., Moine, O., Hatté, C., Fourel, F., Martineau, F., and Rousseau, D.-D.: Palaeotemperature reconstruction during the Last Glacial from δ18O of earthworm calcite granules from Nussloch loess sequence, Germany, Earth Planet. Sci. Lett., 442, 13–20, https://doi.org/10.1016/j.epsl.2016.02.045, 2016.
Pustovoytov, K. and Terhorst, B.: An isotopic study of a late Quaternary loess-paleosol sequence in SW Germany, Revista mexicana de ciencias geológicas, ISSN 1026-8774, Vol. 21, No. 1, 2004 (Ejemplar dedicado a: VI International Symposium and Field Workshop on Paleopedology), 21, 88–93 pp., 2004.
Roden, J. S., Lin, G., and Ehleringer, J. R.: A mechanistic model for interpretation of hydrogen and oxygen isotope ratios in tree-ring cellulose, Geochim. Cosmochim. Acta, 64, 21–35, https://doi.org/10.1016/S0016-7037(99)00195-7, 2000.
Schäfer, I., Bliedtner, M., Wolf, D., Faust, D., and Zech, R.: Evidence for humid conditions during the last glacial from leaf wax patterns in the loess–paleosol sequence El Paraíso, Central Spain, Quaternary Int., 407, 64–73, https://doi.org/10.1016/j.quaint.2016.01.061, 2016.
Schmidt, H. L., Werner, R. A., and Rossmann, A.: 18O pattern and biosynthesis of natural plant products, Phytochemistry, 58, 9–32, https://doi.org/10.1016/s0031-9422(01)00017-6, 2001.
Silva, L. C. R., Pedroso, G., Doane, T. A., Mukome, F. N. D., and Horwath, W. R.: Beyond the cellulose: Oxygen isotope composition of plant lipids as a proxy for terrestrial water balance, Geochem. Perspect. Lett., 33–42, https://doi.org/10.7185/geochemlet.1504, 2015.
Sirocko, F., Knapp, H., Dreher, F., Foerster, M., Albert, J., Brunck, H., Veres, D., Dietrich, S., Zech, M., Hambach, U., Röhner, M., Rudert, S., Schwibus, K., Adams, C., and Sigl, P.: The ELSA-Vegetation-Stack: Reconstruction of Landscape Evolution Zones (LEZ) from laminated Eifel maar sediments of the last 60,000 years, Global Planet. Change, 148, 108–135, https://doi.org/10.1016/j.gloplacha.2016.03.005, 2016.
Spötl, C., Mangini, A., and Richards, D. A.: Chronology and paleoenvironment of Marine Isotope Stage 3 from two high-elevation speleothems, Austrian Alps, Quaternary Sci. Rev., 25, 1127–1136, https://doi.org/10.1016/j.quascirev.2005.10.006, 2006.
Stevens, T., Marković, S. B., Zech, M., Hambach, U., and Sümegi, P.: Dust deposition and climate in the Carpathian Basin over an independently dated last glacial–interglacial cycle, Quaternary Sci. Rev., 30, 662–681, https://doi.org/10.1016/j.quascirev.2010.12.011, 2011.
Zech, M., Tuthorn, M., Detsch, F., Rozanski, K., Zech, R., Zöller, L., Zech, W., and Glaser, B.: A 220ka terrestrial δ18O and deuterium excess biomarker record from an eolian permafrost paleosol sequence, NE-Siberia, Complete, 220–230, https://doi.org/10.1016/j.chemgeo.2013.10.023, 2013.
Zech, M., Mayr, C., Tuthorn, M., Leiber-Sauheitl, K., and Glaser, B.: Oxygen isotope ratios (18O/16O) of hemicellulose-derived sugar biomarkers in plants, soils and sediments as paleoclimate proxy I: Insight from a climate chamber experiment, Geochim. Cosmochim. Acta, 126, 614–623, https://doi.org/10.1016/j.gca.2013.10.048, 2014.
Zech, M., Zech, R., Rozanski, K., Gleixner, G., and Zech, W.: Do n-alkane biomarkers in soils/sediments reflect the δ2H isotopic composition of precipitation? A case study from Mt. Kilimanjaro and implications for paleoaltimetry and paleoclimate research, Isotop. Environ. Health Stud., 51, 508–524, https://doi.org/10.1080/10256016.2015.1058790, 2015.
Zech, R., Zech, M., Marković, S., Hambach, U., and Huang, Y.: Humid glacials, arid interglacials? Critical thoughts on pedogenesis and paleoclimate based on multi-proxy analyses of the loess–paleosol sequence Crvenka, Northern Serbia, Palaeogeogr. Palaeoclim. Palaeoecol., 387, 165–175, https://doi.org/10.1016/j.palaeo.2013.07.023, 2013.