the Creative Commons Attribution 4.0 License.
the Creative Commons Attribution 4.0 License.
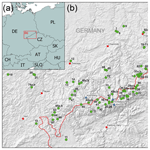
Holocene forest and land-use history of the Erzgebirge, central Europe: a review of palynological data
Martin Theuerkauf
Falk Hieke
Kaiser, K., Theuerkauf, M., and Hieke, F.: Holocene forest and land-use history of the Erzgebirge, central Europe: a review of palynological data, E&G Quaternary Sci. J., 72, 127–161, https://doi.org/10.5194/egqsj-72-127-2023, 2023.
The ongoing ecological conversion of mountain forests in central Europe from widespread Picea monocultures to mixed stands conceptually also requires a historical perspective on the very long-term, i.e. Holocene, vegetation and land-use dynamics. Detailed sources of information for this are palynological data. The Erzgebirge in focus here, with a maximum height of 1244 m a.s.l., represents an extreme case of extensive historical deforestation since the Middle Ages due to mining, metallurgy, and other industrial activities, as well as rural and urban colonisation. For this regional review we collected and evaluated 121 pollen diagrams of different stratigraphic, taxonomic, and chronological resolution. This number makes this region an upland area in central Europe with an exceptionally high density of palynological data. Using well-dated diagrams going back to the early Holocene, main regional vegetation phases were derived: the Betula–Pinus phase (ca. 11 600–10 200 cal yr BP), the Corylus phase (ca. 10 200–9000 cal yr BP), the Picea phase (ca. 9000–6000 cal yr BP), the Fagus–Picea phase (ca. 6000–4500 cal yr BP), the Abies–Fagus–Picea phase (ca. 4000–1000 cal yr BP), and the anthropogenic vegetation phase (ca. 1000–0 cal yr BP). Some diagrams show the presence or even continuous curves of potential pasture and meadow indicators from around 2000 cal BCE at the earliest. Even cereal pollen grains occur sporadically already before the High Medieval. These palynological indications of a local prehistoric human impact also in the higher altitudes find parallels in the (geo-)archaeologically proven Bronze Age tin placer mining and in the geochemically proven Iron Age metallurgy in the Erzgebirge. The pollen data show that immediately before the medieval clearing, i.e. beginning at the end of the 12th century CE, forests were mainly dominated by Fagus and Abies and complemented by Picea with increasing share towards the highest altitudes. According to historical data, the minimum of the regional forest cover was reached during the 17th–18th centuries CE. The dominance of Picea in modern pollen spectra is caused by anthropogenic afforestation in the form of monocultures since that time. Future palynological investigations, preferably within the framework of altitudinal transect studies, should aim for chronologically and taxonomically high-resolution and radiometrically well-dated pollen diagrams from the larger peatlands.
Der ökologische Umbau der Gebirgswälder in Mitteleuropa von vorherrschenden Picea-Monokulturen zu gemischten Beständen erfordert konzeptionell auch eine historische Perspektive auf die sehr langfristige, das heißt holozäne Vegetations- und Landnutzungsdynamik. Eine detailreiche Informationsquelle dafür sind palynologische Daten. Das hier im Fokus stehende, maximal 1244 m NN hohe Erzgebirge repräsentiert einen Extremfall flächendeckender historischer Entwaldung seit dem Mittelalter durch Bergbau, Metallurgie, weitere industrielle Aktivitäten sowie bäuerliche und städtische Kolonisation. Für dieses regionale Review wurden durch uns 121 Pollendiagramme unterschiedlicher stratigraphischer, taxonomischer und chronologischer Auflösung gesammelt und evaluiert. Diese Anzahl macht diese Region zu einem Gebirgsareal in Mitteleuropa mit einer besonders hohen Dichte an palynologischen Daten. Anhand zeitlich bis in das Frühholozän zurückreichender, gut datierter Diagramme wurden regionale Hauptvegetationsphasen abgeleitet: Betula–Pinus-Phase (ca. 11 600–10 200 cal yr BP), Corylus-Phase (ca. 10 200–9000 cal yr BP), Picea-Phase (ca. 9000–6000 cal yr BP), Fagus–Picea-Phase (ca. 6000–4500 cal yr BP), Abies–Fagus–Picea-Phase (ca. 4000–1000 cal yr BP) und anthropogene Phase (ca. 1000–0 cal yr BP). Einige Diagramme zeigen das Vorhandensein oder sogar kontinuierliche Kurven potenzieller Weide- und Wiesenindikatoren ab frühestens etwa 2000 cal BCE. Auch Getreidepollen kommen vereinzelt schon vor dem Hochmittelalter vor. Diese palynologischen Hinweise auf einen lokalen prähistorischen menschlichen Einfluss auch in den höheren Gebirgslagen finden Parallelen in dem (geo-)archäologisch nachgewiesenen bronzezeitlichen Zinn-Seifenbergbau und in der geochemisch nachgewiesenen eisenzeitlichen Metallurgie im Erzgebirge. Die Pollendaten zeigen, dass unmittelbar vor der mittelalterlichen Rodung, das heißt beginnend am Ende des 12. Jahrhunderts, in allen Höhenzonen des Gebirges die Wälder durch Fagus und Abies dominiert waren, ergänzt durch einen zunehmenden Anteil von Picea in den Hochlagen. Das Minimum der regionalen Waldbedeckung wurde nach historischen Daten im 17.–18. Jahrhundert erreicht. Die Dominanz von Picea in modernen Pollenspektren wurde durch anthropogene Aufforstungen in Form von Monokulturen seit dieser Zeit verursacht. Künftige palynologische Untersuchungen, bevorzugt im Rahmen von Höhentransekt-Studien, sollten auf chronologisch und taxonomisch hoch aufgelöste sowie radiometrisch gut datierte Pollendiagramme aus größeren Mooren zielen.
- Article
(15415 KB) - Full-text XML
-
Supplement
(543 KB) - BibTeX
- EndNote
With regard to their forests, the Erzgebirge (Czech: Krušne hoří, English: Ore Mountains; Fig. 1) represent a historical extreme in central Europe, both in environmental and scientific–academic terms. Against the background of harsh but not at all extreme natural conditions in this up to 1244 m a.s.l. high mountain range, extremely intensive mining and other industrial as well as agricultural activities from the 12th to the 18th centuries CE temporarily led to almost complete deforestation (Thomasius, 1994; Hempel, 2009). The extent of clearing is certainly unique in the central European uplands, even though similar medieval to early modern mountain forest and mining areas, such as Harz, Schwarzwald, Sudetes, Böhmerwald/Šumava, or Českomoravská vrchovina, were also affected by intensive overexploitation of its wood resources (Segers-Glocke and Witthöft, 2000; Hrubý et al., 2014; Rösch, 2015; Cembrzyński, 2019; Piekalski, 2020; Kozáková et al., 2022).
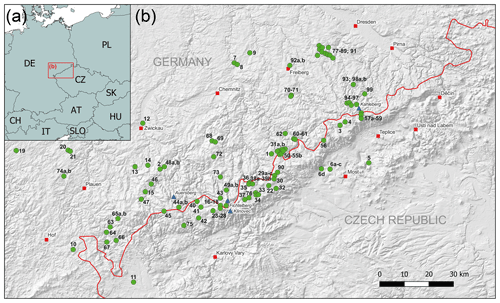
Figure 1Distribution map of pollen diagrams in the Erzgebirge. (a) Situation of the study area in central Europe. (b) Pollen diagrams collected from the study area (green dots are the pollen diagrams with IDs, blue triangles are prominent mountain tops, red squares are towns, and the red line is the state border). For decoding and referencing of the IDs see Table 1. The steep southern escarpment of Erzgebirge to the Ohře/Eger Graben and the North Bohemian Basin is clearly visible in the underlying digital elevation model, forming a diagonal, SW–NE trending structure. The digital elevation data are funded under the GMES preparatory action 2009 on Reference Data Access by the European Commission, DG Enterprise and Industry (https://sdi.eea.europa.eu/, last access: 13 June 2022).
After the re-establishment of now highly artificial (spruce) forests in the 18th and 19th centuries CE (Thomasius, 1994), two further anthropogenically triggered extremes followed in the 20th century CE: first, large parts of the Bohemian Erzgebirge were depopulated by the expatriation of the Germans after World War II. An open, intensively used landscape for centuries widely became a fallow or forested landscape here again (Bičík and Štěpánek, 1994; Zelinka et al., 2021). Second, from the 1960s to the 1990s large parts of the forests died back (German: Waldsterben) due to heavy industrial air pollution basically by sulfur dioxide emissions (Zimmermann et al., 2003; Grunewald and Bastian, 2015; Kupková et al., 2018). Together with the nearby Jizera/Izera Mountains, the Erzgebirge represented a part of the so-called “Black Triangle region”, which was an industrial pollution hotspot caused by lignite combustion for energy generation in the border area between east Germany, the Czech Republic, and Poland. Nowhere else in central Europe was the extent of forest dieback and other environmental effects of industrial air pollution so extreme as here (Kubíková, 1991; Schmidt, 1993; Scheithauer and Grunewald, 2007).
But the Erzgebirge and its forest also came up with – this time positively connoted – extremes from a scientific–academic point of view: the humanistic scholar Georgius Agricola (1494–1555) developed the mining sciences in the Erzgebirge. Various geoscientific disciplines were established at the Mining Academy founded in Freiberg in 1765, one of the oldest in the world. In 1713, the Saxon mining official Hans Carl von Carlowitz (1645–1714) developed the principle of sustainability in forestry due to the pressing regional timber shortage (Grober, 2012; von Carlowitz and von Rohr, 2012). Today sustainability, although more comprehensively defined, is the increasingly influential guiding principle of a meaningful global development. A little later, in 1811–1816, one of the oldest forest faculties in the world was founded in nearby Tharandt, which still influences the forest practice and the regional forest structure to this day.
Finally, at the beginning of the 20th century CE, the Bohemian and Saxon Erzgebirge became the subject of systematic, large-scale palynological investigations into the postglacial forest history (Rudolph and Firbas, 1924): in the early days of palynology and for the first time with such intensity in a central European low mountain range, with astonishing modernity to this day. In general, it can be said for the Erzgebirge that in the past extreme forest resource problems resulted in extreme forest scientific and academic innovation forces!
Now at the beginning of the 21st century CE, after around 200 years of relative climatic stability and after around 30 years of ecological restoration, the Erzgebirge is once again affected by a rapid change in its environment in general and especially in its forests: recent climate change with its extreme weather events, such as storms and droughts, but also initially rather unspectacular, long-term phenomena, such as the increase in temperatures and decrease in snow precipitation (Bednářová et al., 2014; Vlach et al., 2021), predictably lead to a more or less “catastrophic end” to the previous forest management system dominantly comprising spruce monoculture age class forests. The new forest crisis is expressed by large areas severely damaged (12 200 ha) and completely cleared (2600 ha) of mainly spruce forest between 2017 and 2020 by abiotic (drought, storm) and biotic factors (bark beetles; Gdulová et al., 2021). In general, the severe bark beetle infestation in spruces that occurred during the last few years has led to an unheard-of amount of damaged forests since the beginning of regulated forestry in Saxony some 200 years ago (SMEKUL, 2020). Although alternative forest management concepts, such as the principle of natural forest or permanent (mixed) forest, have a long local tradition in the region and beyond (Möller, 1922; Krutzsch, 1952), they could not prevail against the industrial engineering forest mainstream in the 20th century CE (Knapp et al., 2021). Only in the last two to three decades have the forests in the Erzgebirge been increasingly “rebuilt” from an ecological point of view; that is, a mixed mountain forest mainly consisting of beech, fir, and spruce has been replanted at the sites of previous spruce monocultures (Eisenhauer and Sonnemann, 2009; Staatsbetrieb Sachsenforst, 2017).
The underlying theoretical concept is based on both forest ecological observations and ecological–theoretical considerations in the region and beyond (e.g. Ellenberg, 1996; Küssner and Mosandl, 2002), as well as, but to a very limited extent, on forest historical analyses for the past few centuries (Thomasius, 1994; Hempel, 2009). However, the region also has a not yet fully exploited potential for very long-term analyses of forest dynamics depending on natural and anthropogenic processes: more than 120 pollen diagrams have been produced from the Erzgebirge since the 1920s (see Sects. 3 and 4). This number makes this region an upland area in central Europe with an exceptionally high density of palynological data on forest and land-use history.
Initiated by current research on the medieval and early modern mining and environmental history in the Erzgebirge (e.g. Derner, 2018; Hemker, 2018; Tolksdorf, 2018; Cappenberg et al., 2020; Kaiser et al., 2021), the opportunity arose to systematically compile the palynological data available from the region, make it accessible for further research, and carry out overview analyses on it. This review paper therefore focuses on data presentation and certain data reflection, especially in methodical terms, as well as on selected research questions about regional forest and land-use dynamics in the Holocene.
(1) What were the general trends in Holocene forest development in the Erzgebirge? (2) What were the distributional patterns of the main tree taxa (e.g. with respect to altitude and soil) in certain forest vegetation phases? (3) Are there any palynological signals that indicate an already pre-medieval anthropogenic influence on the mountain forests? (4) How were forests composed at different altitudes before extensive clearings in the High Middle Ages (12th–13th centuries CE)? (5) How is the deforestation and the subsequent replacement vegetation reflected palynologically? (6) Are there sediment sequences in the Erzgebirge that could reveal the vegetation development of the recent past, i.e. the last ca. 300 years?
Identified in early medieval sources as Miriquidi (also Mircwidu; old Low German for “dark forest”), the area was referred to as Böhmerwald, Böhmischer Wald, or Böhmisches Gebirge in the high and late Middle Ages up to the Early Modern Period (Hengst, 2006). It was not until the end of the 16th century CE that the name Erzgebirge came up, referring to the character of this area by ore mining (Peschel and Wetzel, 2010). The mountain range has formed a natural border between the territories of Saxony (Germany) and Bohemia (Czech Republic) for around 800 years (Thomasius, 1994; Lauterbach, 2018). For centuries the Erzgebirge played an outstanding role in the mining and other industries in central Europe and made important contributions to ores, metallurgical and other products as well as to technical and academic innovations. Parts of the Erzgebirge were therefore assigned as a UNESCO world heritage site Erzgebirge/Krušnohoří mining region in 2019 (ICOMOS, 2019; Hansell, 2022).
The Erzgebirge has a larger German/Saxon and a smaller Czech/Bohemian part with a total area of ca. 5300 km2. The around 130 km long and 40 km wide Erzgebirge extends from the Elbsandsteingebirge in the northeast to the Elstergebirge (Vogtland) in the southwest (Bramer et al., 1991; Fig. 1). In the north the Erzgebirge Basin and the Mulde-Lösshügelland (loess hills) and in the south the Eger/Ohře Graben and the North Bohemian Basin/Mostecká pánev limit the mountains. The transition from the foothills to the mountains is around 300 m a.s.l. The Erzgebirge is divided into an eastern, central, and western part with different characteristics, especially in terms of geology, geomorphology, and climate.
The Erzgebirge is a Hercynian fault-block mountain range with a steep and high escarpment in the southeast and a gentle slope in the northwest, forming a half-horst (German: Pultscholle) as a whole. As a basement unit, similar to Schwarzwald and Harz, it was folded by the Variscan mountain formation in the Carboniferous, eroded in the Permian, and raised in the Tertiary (Sebastian, 2013; Vilímek and Raška, 2016). In addition to the dominant metamorphic rocks (mainly gneiss but also phyllite, clay, and mica schist), there are plutonites (granite, rhyolite), locally also Cretaceous sediments (mainly sandstone), and tertiary volcanites (basalt, phonolite). Plateaus with deeply incised and terraced valleys that follow the northern slope of the mountains characterise the area. The crest region in particular has wide flat or only flat sloping areas, which formed the topographical prerequisite of Holocene peatland formation. The highest summits are the Klínovec/Keilberg (1244 m a.s.l.) and the Fichtelberg (1215 m a.s.l.) in the central Erzgebirge (Fig. 1). The Pleistocene inland ice reached the edge of the Erzgebirge from the north in the Elsterian glaciation (ca. 500–300 ka) around Freiberg and Chemnitz (Sebastian, 2013). A possible, very local glaciation of the higher elevations of the mountains remains uncertain (Käubler, 1969).
Periglacial debris layers (cover beds) with a high proportion of loess cover almost the entire Erzgebirge and are predominantly the substrates for Holocene soil formation (Kleber et al., 2013). Cambisols and Podzol–Cambisols dominate, supplemented by Podzols, Stagnosols, Gleysols, Fluvisols, and Anthrosols (Sebastian, 2013). Characteristic of the higher elevations of the Erzgebirge are mires, which are mainly formed as raised bogs (i.e. rain-fed mires) and swamp mires (fens; Fig. 2g, h). They are mostly found at watershed positions and in spring basins. In terms of the ecological type, mesotrophic and oligotrophic acidic mires dominate. The area of the peat deposits that existed in the Saxon Erzgebirge up to the end of the 19th century CE is estimated to be 60 km2 (today 42 km2; Zinke, 2002). The original mire area for the entire Erzgebirge is estimated to be 100 km2 (Firbas, 1952). All of the mires in the area were drained, and many were used for peat extraction. For about 30 years, the mires have been the focus of restoration measures in both Saxony and Bohemia (Sächsische Landesstiftung Natur und Umwelt, 2007).
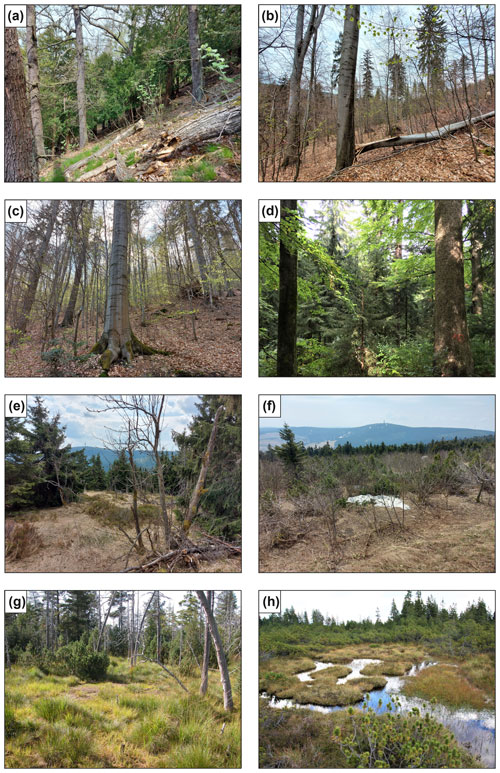
Figure 2Photographs of typical near-natural forest communities and mires in the Erzgebirge. (a) West-exposed slope covered with oak, beech, and yew in the nature reserve Müglitzhang bei Schlottwitz, eastern Erzgebirge, ca. 300 m a.s.l. (photo: K. Kaiser). (b) West-exposed slope covered with beech, maple, fir, and spruce in the nature reserve Weißtannenbestand Pöbeltal, eastern Erzgebirge, ca. 570 m a.s.l. (photo: K. Kaiser). (c) East-exposed slope covered with beech, maple, fir, and spruce in the nature reserve Hofehübel Bärenfels, eastern Erzgebirge, ca. 660 m a.s.l. (photo: K. Kaiser). (d) North-exposed slope covered with beech, spruce, and fir in the nature reserve Riedert, western Erzgebirge, ca. 720 m a.s.l. (photo: F. Jacob). (e) South-exposed slope covered with a patchy stand of spruce and mountain ash/rowan in the nature reserve Fichtelberg, central Erzgebirge, ca. 1170 m a.s.l. (photo: K. Kaiser). (f): Summit plateau of Fichtelberg covered with mountain ash/rowan, dwarf mountain pine, and low individuals of spruce, central Erzgebirge, ca. 1210 m a.s.l. (photo: K. Kaiser). The view is directed towards the highest mountain top of Erzgebirge, the Klínovec/Keilberg (1244 m a.s.l.). (g) Mothäuser Heide mire, central Erzgebirge, ca. 770 m a.s.l. (photo: Wikimedia/K. Keßler). (h) Kleiner Kranichsee mire, western Erzgebirge, ca. 930 m a.s.l. (photo: Wikimedia/K. Keßler).
The Erzgebirge belongs entirely to the Elbe/Labe Catchment, with the mountain crest separating the Mulde from the Eger/Ohře sub-catchments. Zwickauer Mulde and Freiberger Mulde are the main rivers, while Schwarzwasser, Chemnitz, and Zschopau are the most important tributaries. Characteristic of these rivers are deeply incised and steep valleys (Bramer et al., 1991). Since the Middle Ages, the hydrological system in the region has been intensively reshaped through anthropogenic measures, following the energetic needs of mining, metallurgy, and other purposes. The result is a large number of ponds, weirs, ditches, aqueducts, and water pipe tunnels (Wagenbreth and Wächtler, 1988). The Erzgebirge has a number of larger dam lakes, most of which were built in the 20th century CE for flood protection and drinking water production (Landestalsperrenverwaltung des Freistaates Sachsen, 2017).
The Erzgebirge shows a climatic differentiation between a wetter western and a drier eastern part. The average annual precipitation also declines from 1000–1200 mm in the crest area to about 750 mm at the mountain foot (Bramer et al., 1991). Average January and July temperatures show a strong vertical gradient from the mountain foot to the crest at only a short horizontal distance (Chemnitz, 420 m a.s.l.: annual average 7.9 ∘C, July 16.6 ∘C, January −1.2 ∘C; Fichtelberg, 1215 m a.s.l.: annual average 2.9 ∘C, July 11.2 ∘C, January −5.1 ∘C; https://www.dwd.de/DE/Home/home_node.html, last access: 15 April 2022). The Erzgebirge shows a number of impressive weather extremes. These include over the last 100 years a snow depth of 335 cm on 24 March 1944 and a wind peak of 216 km h−1 on 3 January 1976 on the Fichtelberg (1215 m a.s.l.), a temperature minimum of −33.5 ∘C on 1 February 1998 in Kühnhaide (723 m a.s.l.), and an extreme rain event with 312 mm on 12–13 August 2002 in Zinnwald (877 m a.s.l.; https://www.dwd.de/DE/Home/home_node.html, last access: 15 April 2022).
The Erzgebirge contributes to three ecological altitude levels (Ellenberg, 1996), whose original forest vegetation was reconstructed for the High Middle Ages by Hempel (2009) and whose potentially natural vegetation (PNV) was characterised by Schmidt et al. (2002; Fig. 3). In view of the PNV on dry, i.e. zonal locations, oak–beech forests dominate on the submontane level (ca. 300–500 m a.s.l.), fir–spruce–beech forests dominate on the montane level (ca. 500–900 m a.s.l.), and first beech–spruce forests then spruce forests with a high proportion of mountain ash/rowan dominate in the high-montane level (ca. 900–1200 m a.s.l.; Fig. 2a–f). Some substantial differences between original forest vegetation and PNV are discussed in Sect. 6.6. In the central Erzgebirge, the tree line is at ca. 1200 m a.s.l. on the summits of Fichtelberg and Klínovec/Keilberg (Fig. 2e, f), which were in historical times anthropogenically deforested and later reforested (Heynert, 1964). Thus, the highest mountain peaks in the region protrude just a few metres above this assumed tree line. At particular locations such as block heaps, wind lanes, or cold-air-collecting basins, the tree line can very locally drop to ca. 900 m a.s.l. (Heynert, 1964). Damp and wet, i.e. azonal, sites are characterised by different tree species such as ash, black alder, downy birch, and bog pine (Fig. 2g, h). These general orographic–geobotanical features are confronted with special local deviations. Beech, for example, locally reaches altitudes of ca. 1000 m a.s.l., larger (natural) spruce stands come down to ca. 700 m a.s.l., and very deep fir occurrences can also be found well below 300 m a.s.l. Currently, vegetation of the Saxon Erzgebirge is predominantly open (ca. 60 % of open land), the largest area being intensively used for agriculture and forestry, and densely populated and industrialised, especially in the stream and river valleys. Agriculture is characterised in the lower altitudes by cultivation of cereals, oilseeds, and fodder crops. Livestock farming dominates in the upper elevations. Forest has been preserved along steep valley flanks and partly in the watershed areas. With respect to forest structure, planted spruce monocultures dominate (ca. 80 %). The immediate vicinity of Erzgebirge is characterised by climatically very advantageous and settlement-favourable areas such as the Saxon Elbe Valley and the North Bohemian Basin landscapes, both since the Middle Ages even with cultivation of thermophilic crops such as grapes and hops.
Detailed information on the prehistoric and historic settlement and land-use history of the Erzgebirge is given in Sect. 6.4 to 6.6.
Research on regional forest history using palynology began in the Erzgebirge in the 1920s with the investigation of several peatlands along the mountain crest, such as Großer Kranichsee, Gottesgab/Boží Dar, Sebastiansberg/Hora Svatého Šebestiána, and Georgenfeld near Zinnwald/Cínovec (Fig. 1, Table 1). This work was carried out by the botanists Karl Rudolph and Franz Firbas at the German Charles-Ferdinand University in Prague and is considered a pioneer study of palynology in central Europe (Rudolph and Firbas, 1922, 1924; Rudolph, 1928; Firbas, 1952; Beug, 1965, 2003; Tolksdorf et al., 2018). Inspired by this, a short time later further palynological work was carried out in the peat bogs of the Saxon and Bohemian Erzgebirge (Frenzel, 1930; Schmeidl, 1940).
Table 1Pollen diagrams from the Erzgebirge. Further metadata per pollen diagram are given in Table S1 in the Supplement.
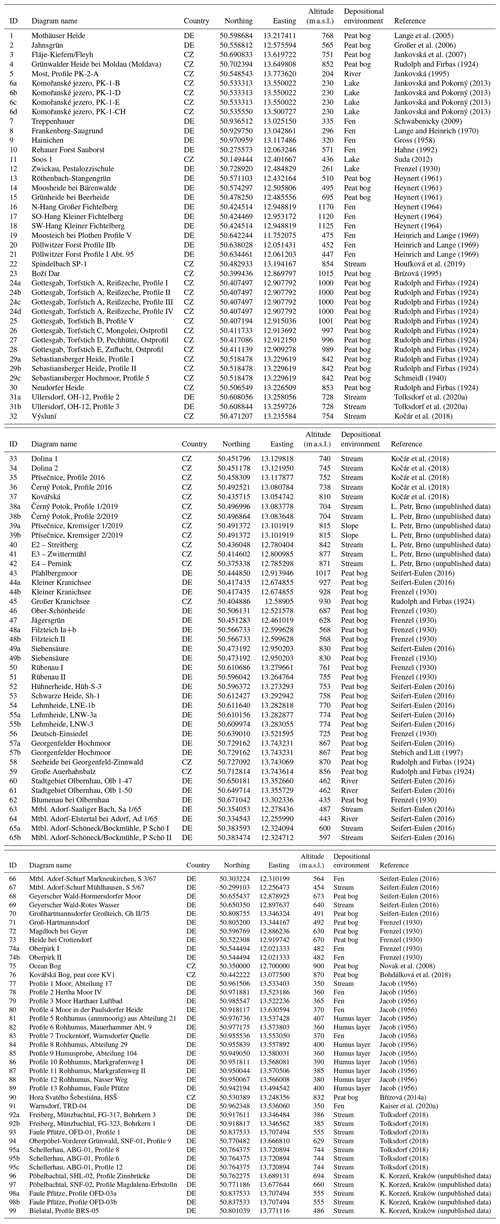
In the 1950s to 1970s, some pollen analyses with a geobotanical, geological, and archaeological focus in the eastern and western Erzgebirge and in the neighbouring Vogtland followed (Jacob, 1956; Gross, 1958; Heynert, 1961, 1964; Heinrich and Lange, 1969; Lange and Heinrich, 1970). On the southern foothills of the Erzgebirge around Most/Brüx, extensive palynological investigations have been carried out since the 1970s in the former lake Komořanské jezero/Kommerner See, which has now been completely devastated by lignite mining (see overviews in Jankovská and Pokorný, 2013; Houfková et al., 2017).
With the political changes in 1989–1990, a strong revival of palynological research began on both the German and the Czech side of the Erzgebirge, which was primarily aimed at questions on the history of the environment, mires, mining, and settlements. In addition to some radiocarbon-dated pollen diagrams, such as from the raised bogs Georgenfeld (Stebich and Litt, 1997), Fláje/Fleyh (Jankovská et al., 2007), Boží Dar/Gottesgab (Veron et al., 2014), and Kovářská/Schmiedeberg (Bohdálková et al., 2018), as well as the deserted villages Spindelbach (Houfková et al., 2019) and Ullersdorf (Tolksdorf et al., 2020a), further diagrams are available but without radiocarbon data (e.g. Großer et al., 2006; Schwabenicky, 2009; Schlöffel, 2011). The Mothäuser Heide pollen diagram presented in detail below (Lange et al., 2005; Theuerkauf et al., 2007; Edom et al., 2011) is also a result of this research period. In addition, there are 20 pollen profiles that were only published in 2016 (Seifert-Eulen, 2016), which were mainly obtained from raised bogs between the 1960s and 2000s.
Comprehensive palynological data were finally produced in the course of the interdisciplinary ArchaeoMontan project, which was dedicated to research into medieval mining history in the Erzgebirge from 2012 to 2018 (Derner, 2018; Hemker, 2018; Tolksdorf, 2018). From this project context, there are now several pollen diagrams and a large number of individual samples that come from alluvial sediment sequences and fens in stream valleys. These diagrams are radiocarbon dated, mostly combined with macro-botanical and anthracological, as well as often also with geochemical–sedimentological data, and above all illuminate the medieval to modern development of vegetation and land use in the central and eastern Erzgebirge. The follow-up project ArchaeoForest (2019–2023) is aimed at the forest history of the last millennium in the Erzgebirge and combines new palynological results, anthracological data from charcoal kilns, dendrochronological material from an archaeological context, and historical sources (Cappenberg et al., 2020).
To sum up, the Erzgebirge can be called a “cradle” of palynological vegetation history in central Europe. With currently more than 120 pollen diagrams, this area has now been examined rather in depth with 100 years of research by means of palynological analyses (Fig. 1, Table 1). While the early palynological work had its starting point primarily in the academic sphere, later project-related investigations by various non-academic institutions prevailed. Recently, however, there has been a revival of academic palynological interest in the region, particularly on the Czech side (e.g. Abraham, 2014; Abraham et al., 2016; Bobek et al., 2019; Marešová, 2022). It shall be noted, however, that most of the existing pollen diagrams cover only (short) sections of the Holocene, usually with low sample resolution and no or only very few independent dating control by radiocarbon ages.
4.1 Pollen diagrams and their metadata
At present, i.e. the end of 2022, a total of 121 pollen diagrams from the Erzgebirge, its immediate foothills, and the Vogtland are available. We here include the Vogtland mountain area, adjoining to the west, because it is difficult to separate from the Erzgebirge in terms of nature and landscape history. Two-thirds of the diagrams are located in Saxony (n=80) and one third in Bohemia (n=41; Fig. 1, Table 1, Table S1 in the Supplement). This compilation includes all palynological records with at least three vertically superimposed pollen spectra. Thus, in addition to classic pollen diagrams, which often comprise several-metre-thick (mostly peat) and in some cases several tens of pollen spectra, very short pollen diagrams of a few centimetres to decimetres are also available from the region and will be considered for this review. However, the number of the latter with 17 diagrams (14 %) is quite small compared to the classic diagrams (n=104, 86 %).
The taxonomic resolution of the older pollen diagrams prepared before about 1950 is limited; i.e. mostly only tree pollen types are included. Only younger records also include herbal pollen. However, published versions even of many recent records often include only selected arboreal and non-arboreal taxa. Only very few records (ca. 10) have been stored in digital repositories.
Of all diagrams, 27 % were published from 1924 to 1940; only 20 % between the 1950s and 1980s; and 53 % after 1989–1990, many of them (35 %) between 2010 and 2020 (Fig. 4a).
The majority of the pollen diagrams, i.e. 35 %, originates from sites between 700 and 900 m a.s.l., 33 % from 300–500 m a.s.l., 18 % from 500–700 m a.s.l., and only 14 % from above 900 m a.s.l. (Fig. 4b). The highest pollen diagrams (IDs 16–18) come from the slope of the Fichtelberg at 1125–1170 m a.s.l. (Heynert, 1964), while the immediate summit positions on this mountain and on the neighbouring Klínovec/Keilberg have not been studied yet.
With regard to the palynologically examined depositional environment, two types dominate: peat bogs (42 %), i.e. rain-fed mires at various topographic positions (Slobodda, 1998; Böhm, 2006; Wendel, 2010) and, with increasing importance in the last decade, river and stream valleys (31 %; Fig. 4c). The latter is mainly represented by flood loam stratigraphies. The rest of the sites (27 %) are distributed among (palaeo-)lakes; fens; slopes (colluvial deposits); and organic soil surfaces, i.e. raw humus layers.
The depth of most pollen diagrams is very small with a value between 50 and 100 cm (24 %), followed by the depth ranges 100–200 cm (23 %) and 200–300 cm (18 %; Fig. 4d). The longest pollen diagram so far, at 790 cm, comes from the peat bog Mothäuser Heide in the central Erzgebirge (Lange et al., 2005; Theuerkauf et al., 2007; ID 1 in Fig. 1). The largest peat thickness is reported from the raised bog Pod novoveským vrchem near Hora Svatého Šebestiána/Sebastiansberg with 10.5 m. This is also the largest known peat thickness in the Czech Republic (https://www.kr-ustecky.cz/, last access: 8 March 2022).
More than a third of the pollen diagrams (40 %) are young; i.e. the base is palynologically dated to the Subatlantic biozone (ca. 2400–0 cal yr BP; Fig. 4e). The second most frequent onset of sedimentation, on the other hand, dates to the Boreal (ca. 10 640 to 9220 cal yr BP; 24 %), followed by the Preboreal (ca. 11 560 to 10 640 cal yr BP, 14 %) and Atlantic (ca. 9220–5660 cal yr BP, 11 %). Remarkably, 7 % of pollen diagrams already started in the Late Glacial, the oldest of which date to the Allerød (ca. 13 350–12 700 cal yr BP).
Micro-charcoal analysis, which is a powerful tool to reconstruct natural and anthropogenic fire dynamics (e.g. Bobek et al., 2019), has only been applied in 27 pollen diagrams (22 %), most of them of a young age with low depth (Conedera et al., 2009; Knapp et al., 2013).
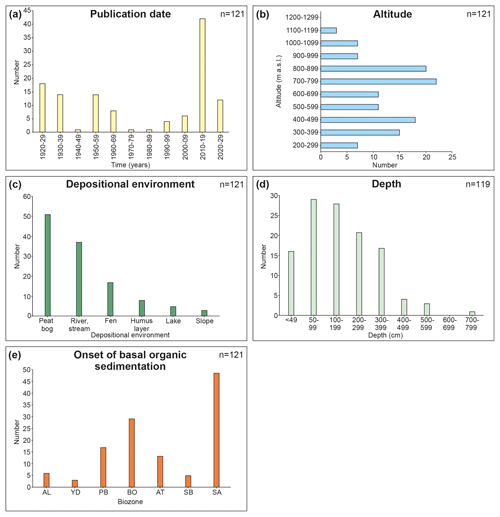
Figure 4Metadata of pollen diagrams from the Erzgebirge. The respective data and further information are given in Tables 1 and S1. (a) Publication date, (b) altitude, (c) depositional environment, (d) depth, (e) and onset of basal organic sedimentation (AL, Allerød; YD, Younger Dryas; PB, Preboreal; BO, Boreal; AT, Atlantic; SB, Subboreal; SA, Subatlantic).
4.2 Chronological control by radiocarbon data
Most pollen records from the Erzgebirge are poorly dated. So far only 118 radiocarbon dates from 43 pollen diagrams are available, with usually only one to three dates per record (Table S1). The majority of 78 profiles (64 %) has no independent age control; i.e. dating is solely based on palynostratigraphy. The latter is calibrated to supra-regional vegetation phenomena or, more recently, to regional historical events (such as the extensive clearing of mountain forests assumed for the 12th and 13th centuries CE; e.g. Hempel, 2009; Kenzler, 2012). Accordingly, the zonation suggested by Firbas (1949, 1952), which is based on the not unproblematic Blytt–Sernander scheme (Birks and Seppä, 2010), must also be used for the regional Holocene biozone stratigraphy (comprising terms such as Preboreal, for instance), which was used anyway in the older regional literature and is therefore necessary for a comparison.
The radiocarbon dates are from bulk peat (41 %), plant macro-remains (17 %), gyttja and organic detritus (15 %), wood (14 %), and charcoal (13 %). Most of the dates were obtained by accelerator mass spectrometry (AMS). The ages dominantly cluster in the Subatlantic biozone, followed by clustering in the Atlantic, Subboreal (5660 to 2400 cal yr BP), and Boreal (Fig. 5). Seven Late Glacial dates (Younger Dryas, 12 700–11 560 cal yr BP; Allerød) are particularly noteworthy, while only one date originates from the earliest Holocene (Preboreal).
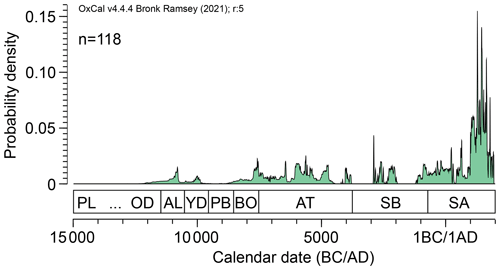
Figure 5Temporal distribution of calibrated radiocarbon ages from pollen diagrams from the Erzgebirge using the OxCal programme (https://c14.arch.ox.ac.uk/oxcal.html, last access: 18 January 2022; Bronk Ramsey, 2017).
On the Saxon side of Erzgebirge, the pollen diagrams with both the highest number of radiocarbon dates ( five each) and a multi-millennial record are those of Georgenfelder Hochmoor (ID 57b in Fig. 1, 867 m a.s.l., nine radiocarbon dates; Stebich and Litt, 1997), Pfahlbergmoor (ID 43, 1017 m a.s.l., seven dates; Seifert-Eulen, 2016), and Mothäuser Heide (ID 1, 768 m a.s.l., six dates; Lange et al., 2005; Theuerkauf et al., 2007). For the Bohemian side, diagrams are available from Boží Dar (ID 23, 1015 m a.s.l., eight dates; Veron et al., 2014) and Komořanské jezero (ID 6d, 230 m a.s.l., six dates; Jankovská and Pokorný, 2013).
In addition, a general comment on the use of absolute chronological terms in this paper is that we deliberately do not use a uniform terminology here but one that is adapted to the context and thus to the usual application. When addressing the dating of pollen diagrams and Holocene vegetation phases, which is a natural–scientific data aspect, we refer to calibrated radiocarbon dates (cal yr BP). However, when contextualising prehistorical or historical anthropogenic developments, calendar years (years BCE/CE or centuries CE) are used, following common practice in the historical sciences.
4.3 Further palaeobotanical data sources from the region
In addition to pollen and archaeological–historical data, there are further natural–scientific data sources from the Erzgebirge that provide palaeobotanical information on Holocene forest and land-use history. Some findings from peat stratigraphy, anthracology, dendrochronology, and botanical macro-remain analysis, briefly outlined below, are largely data sources that have mainly been used regionally no earlier than the 2010s. Accordingly, in contrast to the pollen data and with a certain exception to subfossil wood and dendrochronology, there are no older datasets available.
Since the beginning of palynological and peat stratigraphical studies, special attention has been paid to ”tree stump horizons” that have often been exposed or drilled in the raised bogs of Erzgebirge (Schreiber, 1921; Rudolph and Firbas, 1924; Frenzel, 1930; Schmeidl, 1940; Seifert-Eulen, 2016). This refers to subfossil tree remains found in situ (mostly stumps, rarely trunks), representing mostly Pinus mugo, Picea, and Betula. They occur at certain positions in the mire stratigraphy, usually as a single horizon, rarely as several superimposed horizons. In the early phase of regional mire research, i.e. in the first half of the 20th century CE, many peatlands were exposed by peat cuttings, which enabled an excellent investigation of the local stratigraphy and thus also the observation of these tree stump horizons in a frontal view. The peat horizon in which the tree remains were embedded was mostly heavily decomposed. By contrast, the peat below and above this horizon was much less decomposed. The palynostratigraphical dating of the tree stump horizons yielded different ages from the early Holocene to the late Holocene with a certain accumulation at the turn from Subboreal to Subatlantic (Frenzel, 1930). Already Rudolph and Firbas (1924) paralleled this striking change (i.e. hiatus) in the peat stratigraphy with the so-called Grenzhorizont (“border horizon”) or, more recently, with the Rekurrenzfläche (“recurrence surface”) or Schwarztorf–Weißtorf-Kontakt (“black peat–white peat contact”), representing a widespread peat marker horizon in northwestern Germany and beyond (Weber, 1926; Behre, 2008). Such horizons, indicating a temporary cessation of peat formation and afforestation, have also been detected in raised bogs in other low mountain ranges in central Europe, for instance in the Harz, Rhön, and Fichtelgebirge (Overbeck and Griéz, 1954; Firbas et al., 1958; Willutzki, 1962; Beug et al., 1999). Most of these were dated to the late Holocene and partially paralleled with the peatland stratigraphy in northwestern Germany and the Netherlands. In fact, the dates available from the northwestern German plain show a maximum age range from 2300 BCE to 700 CE with a data cluster between 800 BCE and 100 CE (Behre, 2008). This sequence of more and less decomposed peat layers is currently interpreted as mostly locally caused phases of weaker and stronger peat growth, respectively (Lang, 1994). Furthermore, in the peatlands of this lowland region there are numerous tree stump horizons under and in peat sequences that date back to the mid- and late Holocene (Achterberg et al., 2016). With regard to the state of knowledge in the Erzgebirge, it should be noted that neither dendrological and dendrochronological nor radiometric investigations have been carried out on these tree stump horizons. Botanical macro-remain, charcoal, and pedological data are also missing. As a result, their dating and genesis cannot be presented in detail at present, and a systematic reference to regional peatland development or even to the supra-regional climate development cannot be established thus far.
Recently, a so-called “forest-clearing horizon” with the preservation of subfossil wood as well as charcoal and wooden chips was discovered during a geoarchaeological re-evaluation of the abandoned medieval settlement site of Warnsdorf in the Tharandt Forest (ca. 360 m a.s.l., ID 91; Kaiser et al., 2020a; Fig. 1). A larger in situ root of Pinus, dating 997–1155 CE, was embedded in a peat layer covered by anthropogenic sediments. Additional pollen and plant macro-remain data from the peat together with the subfossil wood and sedimentological data allow for insights into the local vegetation and land-use history of this site during the High Medieval Period. Although the Pinus root exposed no clear-cutting marks, wooden chips from Abies in the same horizon indicate intentional tree cutting (Spehr, 2002). In general, Pinus, Abies, Picea, and Betula were documented as subfossil wood. In addition, Fagus, Quercus, and Corylus were detected in the local pollen record. Similar forest-clearing horizons could also be studied in the Harz and in the Bohemian–Moravian Highlands. This generally constitutes a new and promising geoarchaeological–palaeopedological feature for central Europe, documenting the medieval clearing of mountain forests by human exploitation (Kaiser et al., 2020a).
In the Erzgebirge, as in all other low mountain ranges and in the lowlands of central Europe (e.g. Ludemann, 2010; Schmidt et al., 2016; Rutkiewicz et al., 2019; Kaiser et al., 2020b; Raab et al., 2022), there are countless remains of historical charcoal production. Remains of so-called charcoal kilns or charcoal hearths are detectable as circular and thin accumulations of charcoal several metres in diameter. Small plateaus were usually dug into slopes for this purpose. Initial remote sensing investigations using lidar data showed spatial densities of 40–81 charcoal kilns per square kilometre for three test areas in the central Erzgebirge, which are comparable with data from other German mountains such as the Harz, Schwarzwald, and Kellerwald (Tolksdorf et al., 2020a). Since the 2010s, systematic anthracological investigations have been carried out in these charcoal kilns on both the Saxon and the Bohemian sides of the Erzgebirge. These include botanical determinations of the tree remains and their dating by radiocarbon analysis and dendrochronology (Tolksdorf et al., 2015; Kočár et al., 2018; Tolksdorf, 2018; Tolksdorf et al., 2020a; Neubauer, 2022). The ages range from the end of the 12th to the 18th centuries CE. Timber species determinations show a clear shift in taxa (from Fagus and Abies to Picea and pioneer deciduous tree species) and a reduction in timber diameters over this period (Tolksdorf et al., 2021).
Dendrochronological dating has been used regularly in the Erzgebirge for several decades, mostly in the context of studies on building history, archaeology, forest ecology, and dendro-geomorphology (e.g. Spitzer, 2010; Hoffmann and Heußner, 2013; Tolksdorf and Schröder, 2016; Vejpustková et al., 2017; Vilímek et al., 2018). In particular, the ubiquitous use of construction wood since the Middle Ages for ore mines, ore processing facilities, and water management facilities has subfossilised huge amounts of wood in the region. After their discovery, there is enormous potential here for palaeoecological investigations, which has already been used to some extent. For instance, from the archaeologically investigated medieval mines of Dippoldiswalde (375 m a.s.l.) and Niederpöbel (500 m a.s.l.) in the eastern Erzgebirge, around 3400 botanically determined timbers are available to date (Cappenberg et al., 2020). Most common taxa are Abies (n=2103, 62 %), Picea (n=444, 13 %), and Fagus (n=333, 10 %), with a minor share also Acer, Pinus, Quercus, and Ulmus that occur (together n=520, 15 %). Of these, the dendrochronological dates (n=1112) show a total age interval from the 12th to the 13th century CE, cumulating in the middle of the latter. In addition to similarities, i.e. the usage preference of Abies at the beginning of local mining, differences at both locations are visible, i.e. a different role and dating of Picea and deciduous tree species.
A rather new data source for the Erzgebirge is macro-remain analysis, which has increasingly become available in the last decade, resulting from (geo-)archaeological investigations (e.g. Kočár et al., 2018; Tolksdorf, 2018; Kaiser et al., 2021; Herbig, 2022). The preferably determined herb and shrub species supplement the knowledge on late Holocene vegetation structure in various ecological details. This applies above all in view of the local human impact, e.g. on the deforestation and establishment of a replacement vegetation. In addition, there is sometimes remarkable evidence on the regional use of plants, e.g. as food. In the course of local archaeological studies, remains of figs (Ficus carica) and grapes (Vitis vinifera) were found in Kovářská/Schmiedeberg in Bohemia (810 m a.s.l.; Kočár et al., 2018) and remains of figs, rice (Oryza sativa), and pepper (Piper nigrum) in Freiberg in Saxony (400 m a.s.l.; Schubert and Herbig, 2017). This late medieval to early modern evidence proves both that local elites were supplied with quite luxurious goods and that there were long-distance trade connections, sometimes as far as the Mediterranean region and beyond.
5.1 Methodological aspects
Pollen data cannot be interpreted directly in terms of past plant abundances because single taxa produce pollen in very different amounts, and different pollen types may be dispersed differently. As a result, taxa may be over-/underrepresented in the pollen record. Methods to account for that bias have become available over the past decades, e.g. REVEALS (Sugita, 2007a) and ROPES (Theuerkauf and Couwenberg, 2018) for pollen records from larger lakes and LOVE (Sugita, 2007b) and MARCO POLO (Mrotzek et al., 2017) for pollen records from small forest hollows. Hitherto, such methods have not been applied for the interpretation of pollen records from the Erzgebirge. Moreover, most of the methods require pollen productivity estimates (PPEs), which have not been available from this area to date.
All these methods are suited for the interpretation of a single pollen record. To interpret the larger number of pollen records from the Erzgebirge, we here apply the extended downscaling approach (EDA; Theuerkauf and Couwenberg, 2017; Abraham et al., 2023). This approach explores whether the distribution of plant taxa in the past has been determined by abiotic landscape patterns, e.g. the underlying soils, including site water and relief conditions. The EDA is a forward-modelling approach that determines the most likely vegetation composition with a given landscape pattern, e.g. represented by the soil pattern. We apply the EDA for four distinct pollen stratigraphic phases of the Holocene, which are also well distinguishable in the early pollen records (see Sect. 5.3): the Corylus phase, the Picea phase, the Fagus–Picea phase, and finally the Abies–Fagus–Picea phase. For each phase, we include raw pollen counts from 12 and 15 pollen records from higher altitudes, i.e. in between ca. 400 and 1000 m a.s.l. (Table S2). EDA was not applied to the Late Glacial and very early Holocene periods because in the Erzgebirge the number of records from these particular periods is too low and dating too uncertain. As most of the early records from the 1920s to 1960s do not include herbal pollen, EDA analysis only includes the tree taxa. PPEs are derived by applying ROPES with a pollen record from Lake Černé jezero in the Šumava Mountains (Carter et al., 2018). As a landscape pattern for Erzgebirge we use the soil pattern from the Soil Map of Germany on the scale 1:200 000 for the Saxonian part (https://www.boden.sachsen.de/, last access: 7 January 2022) and the European Soil Database for the Bohemian part (https://esdac.jrc.ec.europa.eu/content/european-soil-database-v20-vector-and-attribute-data, last access: 7 January 2022). For comparison of pollen diagrams, we, whenever raw counts were available, recalculated pollen percentage values based on an upland pollen sum (excluding pollen types attributable to taxa growing on peatlands, such as grass type or Calluna).
5.2 Overview
The, so far, the best overview of the Holocene vegetation history of the higher altitudes of the Erzgebirge is provided by the pollen diagram Mothäuser Heide (ID 1, 768 m a.s.l.), which is also one of the best dated, long pollen records from the region (Lange et al., 2005; Theuerkauf et al., 2007; Edom et al., 2011; Figs. 1, 6, 7). For the early and mid-Holocene, the very early analysed, high-resolution pollen diagram Sebastiansberg (ID 29c, 842 m a.s.l.; Schmeidl, 1940; Fig. 1) is also useful, although limited to tree pollen. For the early Holocene, the pollen diagram Pfahlbergmoor (ID 43, 1017 m a.s.l.; Seifert-Eulen, 2016; Fig. 1) also provides a record of herb pollen.
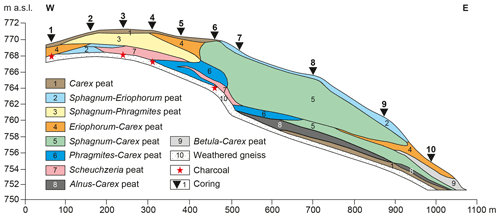
Figure 6Cross-section through the Mothäuser Heide mire, central Erzgebirge (from Edom, et al., 2011, adapted).
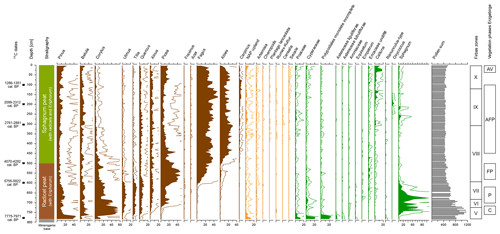
Figure 7Typical pollen diagram from a mire in the montane ecological zone of the central part of the Erzgebirge: Mothäuser Heide (ID 1), 768 m a.s.l. (selected taxa; Lange et al., 2005; Theuerkauf et al., 2007, adapted). The units of the diagram axes below are percentages, except for the pollen sum, which shows counts. Pollen percentages have been calculated based on an upland pollen sum, including trees and shrubs (brown) and upland herbs (orange). The Firbas zones refer to Firbas (1949). Abbreviations for the vegetation phases of the Erzgebirge: C, Corylus phase; P, Picea phase; FP, Fagus–Picea phase; AFP, Abies–Fagus–Picea phase; AV, anthropogenic vegetation).
The latter diagram shows that, like in the northern lowlands, Pinus and Betula were the first tree taxa present in the early Holocene (Firbas, 1952). Corylus was the first thermophilic, deciduous taxon that expanded in the early Holocene, soon followed by Ulmus, Quercus, Tilia, and finally Alnus. As radiocarbon dates from this early period are not available, it yet remains unknown whether the expansion of thermophilic taxa started at ca. 10 500 cal yr BP, as in the northern lowlands (Giesecke et al., 2011), or with some delay. This Corylus-dominated forest phase (see below for details) lasted for about 500–1000 years. The available pollen data are insufficient to evaluate whether the forests of that period were fully closed or if open spots remained. The Pfahlbergmoor diagram shows still clearly elevated pollen percentages of grass pollen during that period, which may indicate some degree of forest openness. However, the grass pollen may also originate from local wetland vegetation.
Forest composition in the Erzgebirge then changed at ca. 9000 cal yr BP due to the expansion of Picea (see Picea phase below), at ca. 6000 cal yr BP due to the expansion of Fagus (see Fagus–Picea phase below), and finally at ca. 4200 cal yr BP due to the expansion of Abies (see Abies–Fagus–Picea phase below; Fig. 7).
Despite the widespread historic evidence of forest clearance also at higher altitudes of the Erzgebirge for the last millennium (e.g. Kenzler, 2012; Tolksdorf, 2018), some long pollen records from larger peatlands in the high altitudes show no clear signal of increased forest openness. In the Mothäuser Heide diagram, for instance, pollen percentages of open indicators such as Poaceae, Artemisia, or Plantago lanceolata remain low. One possible explanation is that the youngest peat layers are missing due to earlier drainage of the peatland (see Sect. 6.4). Nonetheless, Secale pollen is continuously present in low percentages (0.5 %–1 %) in the top 1.5 m of the record comprising the last ca. 2000 years (Fig. 7). Whether these pollen finds indicate that local cereal cultivation in the mountains already started 2000 years ago or rather originated from distant transport from the adjacent southern lowlands, being only ca. 20 km away, remains unclear.
In general, the clearing and occupation period from around the late 12th to the 15th–16th centuries CE saw a drastic decrease in Fagus and Abies; an increase in non-arboreal pollen; and an increase in Picea, Pinus, and other pioneer tree species (Fig. 7). In the last ca. 300–400 years, many diagrams reflect very open landscape conditions, with a renewed forest increase in the last ca. 150 years.
A conceivable establishment of a summary pollen diagram and anthropogenic impact phases for the Erzgebirge, as published in the synthesis of many diagrams, for example, for the Šumava Mountains, Czech Republic, or the Auvergne Mountains (Miras et al., 2018; Kozáková et al., 2022) and the Morvan Mountains, both in France (Jouffroy-Bapicot et al., 2013), respectively, has failed so far due to the lack of digitally available palynological raw data (pollen counts) and well radiometrically dated diagrams.
5.3 Main Holocene vegetation phases of Erzgebirge
5.3.1 Vegetation phases
Betula–Pinus phase, ca. 11 600–10 200 cal yr BP
The pattern and timing of vegetation succession after the onset of the Holocene remain yet unclear because the first millennium of the Holocene is represented in very few, no, or poorly dated diagram sections only. The existing pollen records have been interpreted as the onset of the Holocene triggered immediate forest expansion in the Erzgebirge, starting with a short Betula-dominated phase and followed by a short Pinus-dominated phase. A basal radiocarbon date from the pollen diagram Pfahlbergmoor (ID 43, 1017 m a.s.l.; Seifert-Eulen, 2016; Fig. 1) may instead suggest that early Holocene forest expansion was delayed by at least several centuries. Moreover, pollen of open vegetation indicators, such as Juniperus and Artemisia, is still well present during the Betula- and Pinus-rich sub-phases. Hence, patches of open vegetation likely also existed during these sub-phases. Besides Betula and Pinus, further tree taxa that are poorly represented in the pollen records, such as Populus or Sorbus, may also have played some role.
Corylus phase, ca. 10 200–9000 cal yr BP
In this phase, forests of the Erzgebirge were dominated by deciduous tree taxa. Altitudinal trends are not recognisable for this period (Fig. 8).
Picea phase, ca. 9000–6000 cal yr BP
Forest composition then changed around 9000 cal yr BP due to the expansion of Picea in the Erzgebirge. Still, the forests of the following Picea phase were dominated by deciduous tree taxa, i.e. mostly Tilia, Ulmus, Quercus, Corylus, and Fraxinus. The altitudinal gradient suggests that Picea was increasingly abundant in higher altitudes, while Corylus and Quercus were less abundant (Fig. 8).
Fagus–Picea phase, ca. 6000–4500 cal yr BP
At around 6000 cal yr BP, Fagus expanded in the Erzgebirge, while Ulmus and Tilia and later Quercus sharply declined. The decline in Ulmus likely corresponds to the mid-Holocene elm decline well known from sites across central and northwestern Europe (Giesecke et al., 2017). The altitudinal gradients show that Picea was present in the highest altitudes of the mountains, whereas Fagus and other deciduous taxa were the most abundant in the lower and mid-altitudes (Fig. 8).
Abies–Fagus–Picea phase, ca. 4500–1000 cal yr BP
At around 4500 cal yr BP, forest composition again changed due to the expansion of Abies. Until about 1000 cal yr BP, Abies and Fagus became the dominating tree taxa in the Erzgebirge. Picea existed more frequently than before and was mainly limited to the highest altitudes (Fig. 8).
Anthropogenic vegetation, ca. 1000–0 cal yr BP
The past millennium is generally poorly represented in pollen records from the large Erzgebirge peatlands. Older pollen records mostly lack herbal pollen and hence are unsuited for exploring the role of anthropogenic vegetation. Younger records suffer from the decomposition of upper peat layers due to modern drainage. Hence, the past millennium is generally represented with a few samples in longer peat sequences only. Only the pollen diagram Kleiner Kranichsee shows a somewhat higher resolution (ID 44a, 927 m a.s.l.; Seifert-Eulen, 2016; Fig. 1). Moreover, due to the lack of radiocarbon dates, dating mostly relies on biostratigraphy. The widespread prominent increase in synanthropic pollen types, i.e. Cerealia, Secale, Plantago lanceolata, and Rumex acetosella, is attributed to the high medieval colonisation of that area in the late 12th to 13th centuries CE (e.g. Großer et al., 2006). Even in the highest altitudes of the Erzgebirge does the sum of synanthropic indicators arrive at about 10 % and the sum of all terrestrial open indicators (including grasses) at 30 %–50 %, indicating largely open conditions and widespread agricultural activity. At lower altitudes the sum of synanthropic pollen types arrives at about 20 %, indicating even more open vegetation and stronger agricultural activity.
While the increase in synanthropic pollen types indicates a decline in forest cover, changes in tree pollen composition indicate additional changes in forest composition. Clearly lower pollen percentages in Fagus and Abies and higher percentages in Pinus and Picea indicate a shift in overall forest composition towards Pinus and Picea. Whether, with overall declining forests, Pinus and Picea indeed expanded cannot be estimated with the data available. The increasing values of Pinus could possibly also partly be attributed to Pinus mugo on the peatlands in higher altitudes and widespread Pinus sylvestris plantation in lower altitudes since the late 18th century CE. The observation that Abies and Fagus, but not Picea, declined due to more intense land use possibly reflects that Abies and Fagus formerly occupied sites more suitable for land-use activities, i.e. better drained and climatically favourable sites.
5.3.2 EDA analysis
For the early Holocene Corylus phase, EDA analysis suggests that the forests on the widespread loamy soils were dominated by Corylus, Tilia, and Ulmus, whereas on the sandy soils Corylus, Tilia, and Betula were most abundant (Fig. 9). For the areas with debris-rich soils, a higher cover of Fraxinus is indicated. Pinus as the only coniferous taxon of that phase was probably mostly rare, covering only about 10 % of the main substrate types.
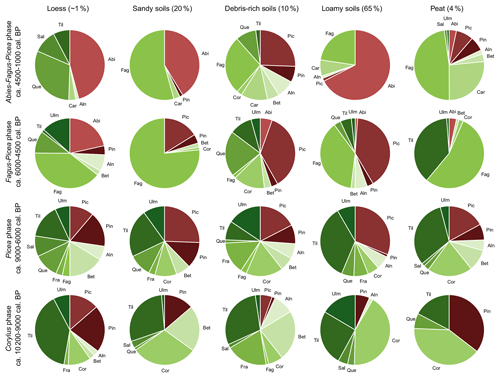
Figure 9Results of the extended downscaling approach (EDA) analysis, showing the forest composition for typical soil sites for four Holocene vegetation phases in the Erzgebirge. The numbers behind the soil types above show their share in the region. Using the example of Abies during the Abies–Fagus–Picea phase, ca. 4500–1000 cal yr BP, it becomes clear what a strong influence the soil type has on the modelled forest composition. Abies dominates the loamy soils with 70 % and shows a much lower share with 45 % and 42 % at the sites with loess and sandy soils, respectively. Abies has a very low share of 5 % in peat soils and does not occur on debris-rich soils.
For the following Picea phase, EDA analysis indicates small differences in forest composition between sandy and loamy soils, with Tilia being somewhat more abundant on loamy soils (ca. 35 %) than on sandy soils (ca. 20 %; Fig. 9). Coniferous trees, mostly Picea, probably covered about one-third of the mountains. Pinus was also present, except on loamy soils.
For the Fagus–Picea phase, EDA analysis suggests that Fagus became the dominant tree taxon on sandy soils, possibly covering ca. 75 % of those areas (Fig. 9). Other deciduous tree taxa largely disappeared from these sites. Picea and Pinus played only a minor role. For loamy soils, a co-dominance of Fagus and Picea is indicated; other tree taxa were rare. EDA analysis indicates dominance of Picea, Quercus, Corylus, and Tilia on debris-rich soils; Fagus was likely rare here. For the rare sites with thicker loess substrate, first stands of Abies are also indicated.
For the Abies–Fagus–Picea phase, EDA analysis indicates co-dominance of Fagus and Abies on sandy and loamy soils, with a somewhat higher cover of Fagus on sandy soils and a somewhat higher cover of Abies on loamy soils (Fig. 9). Other deciduous taxa and Picea likely remained limited to other sites, i.e. debris-rich soils.
5.3.3 Local vegetation differentiation and conceptual summary
For the area around the Mothäuser Heide pollen diagram (ID 1; Fig. 1), the spatial distribution of the vegetation can be reconstructed with the help of the EDA analysis for four vegetation phases. The diagram itself is at 768 m a.s.l., while the surrounding area has an altitude range of ca. 620 to 800 m a.s.l. This area is characterised by a rather flat relief, loamy soil substrates at dry to wet sites, and peat at very wet sites. The current local climate parameters (1980–2010 period) show an annual average temperature of 4.9 ∘C and an annual precipitation of 937 mm (weather station Kühnhaide, 723 m a.s.l.; https://wetter-kühnhaide.de/, last access: 15 April 2022). The distribution of past vegetation is shown schematically (Fig. 10). Only those species for which clear connections with the respective site types were observed are shown. Occurrence of other species in small proportions (e.g. Acer) are likely. In general, there were clear changes in the vegetation over time on all site types, with the exception of the stream sites, which were initially dominated by Corylus and later by Alnus (Theuerkauf et al., 2007).
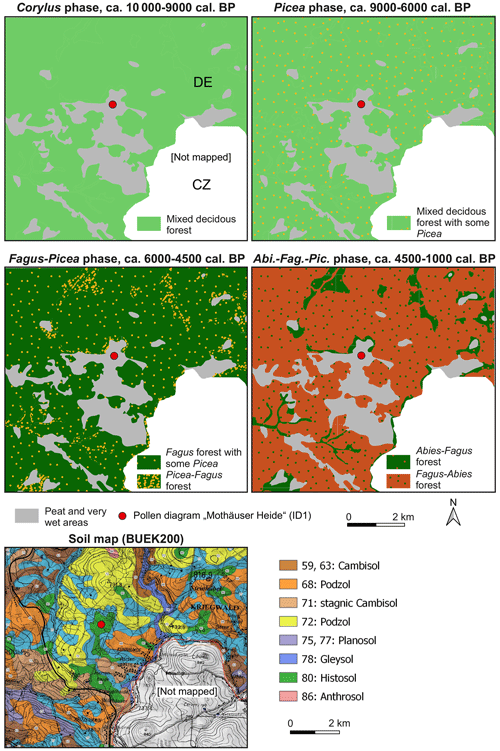
Figure 10Palaeovegetation maps of the Mothäuser Heide mire for four Holocene vegetation phases based on the extended downscaling approach (EDA) analysis. The mixed deciduous forest in the 10 200–9000 cal yr BP phase was dominated by Corylus, Tilia, and Ulmus, while on the same sites in the 9000–6000 cal yr BP phase Tilia, Ulmus, Quercus, Corylus, and Fraxinus dominated, supplemented by Picea. Below is a soil map in the same section as the palaeovegetation maps above (Soil Map of Germany 1:200 000; BGR, modified).
The outlines of the Holocene vegetation history described in the section above are presented as a conceptual pictorial model for a general, i.e. “normal zonal”, site in the montane ecological zone in Fig. 11. All previous concepts for the phase structure of the Holocene vegetation history, including the new suggestion here, are listed in Table 2.
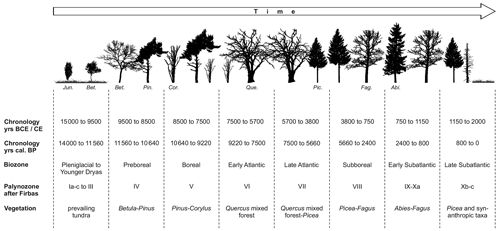
Figure 11Conceptual model of the late Pleistocene and Holocene forest vegetation development in the montane ecological zone of the Erzgebirge using a pictorial timeline. The trees shown symbolise the eponymous vegetation phases and do not reflect the complete mixing ratios of the tree taxa that were actually present (abbreviations for woody taxa: Jun., Juniperus communis; Bet., Betula spec.; Pin., Pinus sylvestris; Cor., Corylus avellana; Que., Quercus spec.; Pic., Picea abies; Fag., Fagus sylvestris; Abi., Abies alba).
Table 2Late Pleistocene and Holocene forest vegetation phases in the Erzgebirge after different authors.
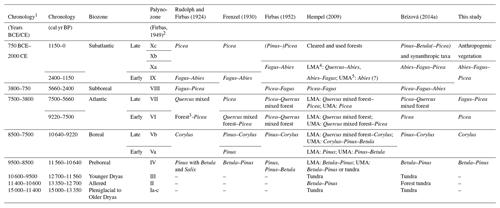
1 Correlation of chronology, biozone, and pollen zone is based on Giesecke et al. (2012, adapted). 2 Modified after Lang (1994). 3 Consisting of Quercus, Tilia, Ulmus, Fraxinus, and Acer. 4 LMA, lower mountain area (ca. 300–800 m a.s.l.). 5 UMA, upper mountain area (ca. 800–1200 m a.s.l.).
6.1 General considerations
Despite a sometimes-high density of pollen diagrams in the landscapes of central Europe, regionally oriented diagram compilations and syntheses on the Holocene vegetation history are rare. A remarkably early example relates to Bohemia, where 130 pollen diagrams were compiled and evaluated already in the 1920s (Rudolph, 1928). More recent examples from central Europe include the Island of Rügen/Baltic Sea (n=40; Lange et al., 1986), the Berlin area (n=73; Behre et al., 1996), the eastern Alps and their forelands (n=301; Wahlmüller, 1993), Hrubý Jeseník (n=16; Dudová et al., 2018), Harz (n=37; Beug et al., 1999), Schwarzwald (n=101; Lang, 2005), Bayerischer Wald (n=22; Stojakowits and Friedmann, 2019), the Northern Calcareous Alps of Germany and Austria (n=32; Friedmann et al., 2022), Šumava (n=30; Kozáková et al., 2022), and the entire territory of Bohemia and Moravia (n=40; Bobek et al., 2019). Although some data in online repositories are available (e.g. https://epdweblog.org/, last access: 3 June 2022; https://www.pangaea.de/, last access: 3 June 2022; https://botany.natur.cuni.cz/palycz/, last access: 3 June 2022), these databases are usually incomplete for the regions and do not provide any synthetic analyses on the regional vegetation history “at the push of a button”, so to speak. For the analysis of a specific region, the only option that remains is to compile the palynological data, including the accompanying radiocarbon data, as completely as possible and, if available, to open up further data sources such as macro-remain analyses, dendrochronological and anthracological analyses, or historical data on vegetation and land-use dynamics.
For the Erzgebirge so far 121 pollen diagrams have been produced. This is somewhat surprising at first, since even scholars familiar with the regional data material noted in the 2000s and 2010s that the regional palynological database, particularly new pollen diagrams, would be rather small (e.g. Lange et al., 2005; Sadovnik et al., 2013). This “astonishing growth” since then is certainly due to the fact that in this paper not only classical pollen diagrams were sought and found, but also pollen diagrams from a broad disciplinary perspective, including palynology, geology, geomorphology, archaeology, and peatland studies; this compilation was done often providing “unconventional” palynological data. Looking at the point in time when the diagrams were created, it becomes clear that despite an undoubted revival of regional palynology after 1989–1990 (53 % of the diagrams), there was already a broad base of older pollen diagrams before (47 % of the diagrams). However, it must be emphasised that many of the 121 diagrams are of little use for a well-elaborated vegetation history because (1) most of them were not independently dated by radiocarbon ages and can hardly be dated by pollen stratigraphy; (2) many published diagrams are of poor quality and incomplete, and the pollen counts are not available digitally; and (3) many diagrams are strongly influenced by local or aquatic pollen input.
The following Discussion section is largely based on the questions that we formulated in the introductory section. All in all, of course, this review is to be understood as an overview of the all material available on the Holocene vegetation history from a palynological perspective. Further questions on details of the regional forest history such as a fine differentiation of the local vegetation according to altitude, soil, water balance, and exposure; the possible reflection of natural disturbance events (e.g. storms, fires, biotic calamities); or the analysis of the long-term diversity of plant taxa in the regional mountain forests are, thus, reserved for future studies. These studies should then be done in conjunction with newly created, taxonomically as well as chronologically high-resolution pollen diagrams, including age control by radiocarbon dating.
Nevertheless, as can be seen from the larger text volume of Sect. 6.5 and 6.6, we would like to place a certain emphasis on the conditions in the Middle Ages and in the Early Modern Period. This can be explained by the reason for this review, i.e. by corresponding projects with a focus on this time. The natural forests of the medieval past could serve as a useful model for the necessary ecological forest conversion of the future (Piovesan et al., 2018; Morel and Nogué, 2019; Słowiński et al., 2019).
6.2 Critical reflection of the EDA results
We in this study for the first time applied EDA analysis in a larger mid-mountain range. Smaller mountain regions have already been investigated using EDA in the North Bohemian sandstone areas (Abraham et al., 2023). The primary goal was to explore major vegetation patterns in relation to soil conditions. Such patterns are indeed apparent, e.g. the higher cover of Fagus on sandy soils and the higher cover of first Picea and later Abies on loamy soils during the Fagus–Picea and the Abies–Fagus–Picea phase. However, in particular results for rarer taxa should not be over-interpreted because of limitations of the data available. We for each phase could include only 12–15 pollen records, whereas a number of 20 or more is suggested for robust results (Theuerkauf and Couwenberg, 2017). Moreover, most pollen records are characterised by low sample resolution and low pollen counts, which implies high error margins, particularly for rarer taxa and the rare soil types. Due to the often-lacking herbal pollen, we had to limit EDA analysis to tree taxa. This limitation is the most relevant for the Corylus phase, when the vegetation of the Erzgebirge may still have been partly open. During the three later periods, patches of open vegetation were likely rare.
Previous syntheses on the Holocene vegetation history in the Erzgebirge (e.g. Rudolph and Firbas, 1924; Firbas, 1952; Hempel, 2009; Seifert-Eulen, 2016) have only marginally dealt with the potential dependence of palaeovegetation on soil patterns. Their focus was mostly on the larger-scale vegetation reconstruction as a function of altitude. An exception is the palynological research of Jacob (1956), who has already demonstrated a clear dependence of the palaeovegetation on the site pattern through a large number of pollen diagrams for the low-lying Tharandt Forest (ca. 190–460 m a.s.l.).
One key problem in all quantitative approaches, including EDA, for the interpretation of pollen records is the selection of a suitable pollen dispersal model. In the absence of other options, we have used a Lagrangian stochastic model adjusted for a lowland setting. Even though the northern part of the Erzgebirge is rather flat, this dispersal model is not ideal for the given mountain setting. Finally, our analysis has been limited to soil patterns, yet other environmental parameters are certainly relevant in the mountain region as well, namely the (micro-)relief. Including these parameters will be a worthwhile next step for palynological research, at least when more pollen data from the region become available.
6.3 Drivers of natural forest dynamics
The early Holocene forest expansion, including first the cold-adapted tree taxa Betula and Pinus and later the warm-adapted tree taxa Corylus, Ulmus, Quercus, Tilia, and Alnus, has been climatically triggered by the warming of the Holocene. As precise dating of early Holocene pollen records in the Erzgebirge is still lacking, it remains open whether the tree taxa expanded later than in the surrounding lowlands, i.e. whether the higher altitude caused some delay in warming.
Drivers of the later major changes in forest composition, i.e. formed by Picea (ca. 9000 cal yr BP), Fagus (ca. 6000 cal yr BP), and Abies (ca. 4500 cal yr BP), are less clear. All three events match the overall Holocene spread of these taxa across central Europe (Giesecke and Brewer, 2018), suggesting that differential expansion in the Erzgebirge is due to the more or less rapid Holocene expansion of these taxa and is not related to specific climatic events. This hypothesis might be tested by comparison of the exact timing of expansion in the western, central, and eastern Erzgebirge, thus comprising a distinct spatial gradient. Fagus, for example, expanded much earlier in the central European Šumava Mountains to the southwest (Kozáková et al., 2022) than in the Hrubý Jeseník Mountains to the east (Dudová et al., 2018), reflecting an eastward migration of this taxon. Hence, for the Erzgebirge one would expect that Fagus accordingly expanded from west to east. However, sample resolution and dating of the available pollen records are insufficient to prove such a trend at the moment.
Moreover, in the Mothäuser Heide (ID 1) record, the expansion of Fagus at ca. 5900–5800 cal yr BP appears synchronous to the decline of Ulmus and Tilia, which may point to four different explanations. First, under the assumption that the Ulmus decline in the Erzgebirge is analogous to the well-known Ulmus decline period in the lowlands of northwestern Europe (ca. 6350–5280 cal yr BP; Parker et al., 2002), i.e. has also been caused by a pathogen, the expansion of Fagus may have been triggered by the demise of Ulmus. Secondly, the synchronous Ulmus decline and Fagus expansion are purely incidental. Thirdly, the decline of Ulmus (and Tilia) in the Erzgebirge has not been caused by a pathogen attack but by the expansion of Fagus, thus by species competition. Finally, the decline of Ulmus and the expansion of Fagus may have been triggered by the same event, such as climate fluctuation. Again, the available pollen records are insufficient to prove these above-mentioned hypotheses. Therefore, other possible natural disturbance factors that could also have had a strong impact on the regional forest vegetation in the past, such as insect outbreak, drought, storm, or fire (e.g. Kulakowski et al., 2017; Bobek et al., 2019; Schafstall et al., 2022), have not yet been evaluated for the study area.
Altitudinal analysis of the pollen records suggests that altitude had only limited effects on forest composition above 300–400 m a.s.l., except that during all times pollen proportions of Picea were the highest in the altitudes above ca. 1000 m a.s.l. (Fig. 8). Picea was likely always most abundant along the highest altitudes.
6.4 Prehistoric human impact
So far, there are only a few indications of an already pre-medieval anthropogenic influence on the mountain forests from the pollen diagrams available in the Erzgebirge. Some diagrams (e.g. Mothäuser Heide, ID 1) show the presence or even continuous curves of potential pasture and meadow indicators such as Artemisia, Rumex, and Plantago from around 2000 cal BCE at the earliest. Even cereal pollen occurs sporadically already before the High Medieval, i.e. before ca. 1150 CE. However, there are usually no previous or synchronous drastic declines in tree taxa or tree species shifts that would indicate corresponding forest clearings. Moreover, the low percentages of the synanthropic taxa (Mothäuser Heide, ID 1: 1.4 % maximum for the period 3000–1000 cal yr BP) do not rule out long-distance input from the surrounding low-lying areas, some of which, particularly in the Elbe/Labe River valley to the east, in the Lösshügelland to the north and in the Ohře Graben and the North Bohemian Basin to the south, have been intensively populated from the Neolithic onwards (Blažek et al., 1995; Christl and Simon, 1995; Kenzler, 2012). In general, there are no in-depth studies on recent pollen precipitation for the Erzgebirge that would allow for an assessment of the possible far-distance pollen transport even in (pre-)historic times. In particular, peat profiles at crest sites close to the steep southern slope of the mountain range have a potentially increased exposure to supra-regional pollen input. Corresponding studies in the Schwarzwald in SW Germany urge caution in the interpretation of those taxa, which occur in low percentages (Hölzer and Hölzer, 2014).
An interesting coincidence of synanthropic taxa (reflecting land use), heavy metal deposition (mining and metallurgy), and charcoal (fire) is shown in the pollen and geochemical data from the Kovářská bog at 870 m a.s.l. in the central Bohemian Erzgebirge (Bohdálková et al., 2018). Already from ca. 950 cal BCE has there been evidence of taxa that indicate grain cultivation, grazing/meadow management, and clearing of forests. Although these findings suggest local human activities in the mountains themselves during the Bronze Age, early Iron Age, and Roman Age, the corresponding archaeological findings from the immediate vicinity of the pollen diagram (up to ca. 5–10 km distance) are still missing.
This leads to the general question on the evidence of prehistoric anthropogenic activities in the study area, indicated by archaeological finds and place names. In the lower eastern Saxon Erzgebirge southeast of Freiberg, at an altitude of ca. 300 m a.s.l., both late Neolithic and Iron Age artefacts have been found (Kreienbrink et al., 2020). However, individual prehistoric finds, such as stone axes or metal objects, have been found at various locations throughout the mountains, including the crest area (Christl and Simon, 1995). In the neighbouring Vogtland area, however, up to at least about 400 m a.s.l. a larger number of Bronze Age sites, including evidence of synchronous metallurgy (Tolksdorf et al., 2019), exist. At the Erzgebirge historic Slavic place names usually occur below 300 m a.s.l. Only very occasionally is there evidence of place names at higher altitudes, such as the town name Zöblitz at 600 m a.s.l. in the central part (Wenzel, 2016). But the majority of historical place names are of German origin and date from the Middle Ages or Modern Period. Also, in the Bohemian Erzgebirge there have so far only been single prehistoric stray finds (Blažek et al., 1995). Kenzler (2012) summarises that there have been no permanent settlements in the mountains for the entire prehistory. According to the available records, the Erzgebirge were only visited for raw material extraction, hunting, and crossing. However, this interpretation can also be due to the state of research, as findings on prehistoric settlements or intensive use of other low mountain ranges in central Europe suggest (e.g. Rösch, 2013; Kozáková et al., 2015, 2022; Henkner et al., 2018; Dreslerová et al., 2020; Wiezik et al., 2022).
In view of the early human impact, the archaeological indications of Bronze Age tin placer mining around 2000–1300 cal BCE from the western and eastern Erzgebirge from altitudes of 900 and 740 m a.s.l., respectively, are particularly remarkable (Bartelheim and Niederschlag, 1997; Tolksdorf et al., 2020b). These mining and probably even metallurgic activities were inevitably associated with (ultra?)local forest clearings and also required transport routes, which have been at least partially free from vegetation. To date, however, there are no pollen diagrams near these early mining sites with a potential palynological record of these local dynamics. Available diagrams in greater distance to these sites (IDs 3, 4, 57b, 44a, 44b, 45; Fig. 1) show no pollen signals specifically for the period 2000–1300 cal BCE that would indicate deforestation or other anthropogenic activities in the area. However, geochemical and palynological evidence of local early mining in the Bronze Age, early Iron Age, and Roman Age is also available from the Bohemian Erzgebirge (Veron et al., 2014; Bohdálková et al., 2018). In summary, it is very likely that prehistoric mining in the Erzgebirge, which has only just initially been researched, led to local forest clearings and the development of replacement vegetation communities at certain sites and routes. Whether other local land-use activities also took place at least seasonally (e.g. grazing by transport animals and livestock, hunting, timber extraction, and honey production) can only be assumed analogous to other prehistoric and early medieval mountain areas in central Europe (e.g. Rösch, 2000; Valde-Nowak, 2013; Schreg, 2014; van der Knaap et al., 2020; Hrubý, 2021).
In addition, it should be mentioned that the Erzgebirge were already a transit area in the prehistory, specifically since the Neolithic, connecting today's central and northern Germany with the Bohemian Basin. Corresponding paths crossed the mountain crest area at various points (Hauswald and Simon, 1995; Ruttkowski, 2002; Kenzler, 2012). Along these routes and in local camps, the latter potentially necessary as rest stops for shelter, changing horses, and procuring food and fodder (Bell, 2020), the natural forest vegetation has certainly been anthropogenically modified.
6.5 Forest structure and human impact in the Middle Ages
Already the early palynological works on the Erzgebirge have suggested that before the high to late medieval clearings (late 12th to early 16th centuries CE), the montane zone was densely forested, mainly by Fagus and Abies with a certain amount of Picea (Rudolph and Firbas, 1924; Frenzel, 1930; Firbas, 1952; Jacob, 1956). Following that, Picea was more abundant or even dominant in the crest area. Locally, i.e. in the mires there, Pinus mugo likely played an important role.
For the low-lying Tharandt Forest (ca. 190–460 m a.s.l.) it could be shown, using a spatial approach based on a total of 22 pollen profiles (IDs 77–89, Fig. 1), which is unique for the study area thus far, that the tree species composition described above was much more spatially complex in detail (Jacob, 1956). Different locations here, i.e. differences in relief, exposure, substrate/soil, and water balance, led to a close-meshed pattern of different forest vegetation. Indeed, Fagus and Abies occurred extensively on slopes and plateaus. Picea grew in the floodplains of streams together with Alnus glutinosa and Betula pubescens. In addition, Picea occurred in further cool and damp to wet locations (steep northern slopes, ravines, swamps, and mires). Pinus sylvestris, Quercus species, and Betula pendula inhabited rocky outcrops, sandy soils, and sunny slopes. Based on the data available at this time, the distribution pattern of Fraxinus excelsior, Ulmus species, Acer species, and Populus species remains somewhat unclear. These tree species obviously did not play a significant role over a large area, but they were mixed in. Corylus avellana was common in bright places. The variance of shrubs was not determined. In addition to this site-related control of the tree species distribution, there were also dynamic biotic and abiotic effects, e.g. as a result of the life cycle of the trees, competition, or calamities (e.g. insect infestation, fire, relief instability/soil erosion; Leuschner and Ellenberg, 2017). Although the example of the Tharandt Forest, which has been very well examined palynologically, represents a forest lying low in the Erzgebirge, it can be assumed that a similarly complex spatial differentiation also applied to the higher-lying areas in the region. This is indicated by the record of current site-related tree species differences in near-natural forest protection areas on higher altitudes in that region (Sächsisches Staatsministerium für Umwelt und Landwirtschaft, 2008).
Already the early works indicate the end of a large-scale Fagus and Abies dominance and the formation of replacement vegetation in the 12th–13th centuries CE according to the still-valid basic outlines of the medieval settlement history in the region. The current dominance of Picea was interpreted as the result of natural reforestation after abandonment of intensive land use in the late Middle Ages and in modern times but above all of reforestation since the 18th century CE. This has been clearly proven both by older investigations and by more recent forest historical investigations (e.g. Kienitz, 1936; Nožička, 1962; Thomasius, 1994). However, the temporal resolution of the last ca. 1000 years in the older pollen diagrams was normally low, and the general focus was mostly on the entire Holocene, neglecting a detailed characterisation of the vegetation dynamics in the Middle Ages and afterwards.
Even from the more recently analysed pollen diagrams do only a few have a sufficiently large thickness of sediments originating from the last ca. 1000 years, i.e. enabling a higher temporal resolution, and an independent chronological control by radiocarbon dates. Of these, the diagram SPI-1, Spindelbach (ID 22, ca. 850 m a.s.l.; Houfková et al., 2019; Marešová, 2022; Fig. 1), located on the crest area of the Bohemian site of central Erzgebirge, is particularly suitable for characterising the local forest vegetation immediately before the start of the medieval clearing and the moment of deforestation, as well as the development of replacement vegetation. This alluvial–colluvial sequence is 82 cm thick and dated by four radiocarbon ages. The dominance of pollen from the climax tree species Fagus (max 15 %), Abies (max 12 %), and Picea (max 10 %), accompanied by a high amount of fern spores (max 40 %), indicates that the broader area around the sampling site was still forested at the beginning of the 14th century CE. The area was cleared around 1350 CE for the establishment of an agrarian settlement (called Spindelbach after written medieval sources), as reflected by a decrease in the sum of tree pollen to below 12 %, the increase in non-arboreal pollen up to 79 %, and the emergence of various synanthropic taxa. According to the pollen data, livestock breading and grain cultivation have been the main economic bases in the village area. Moreover, as at the same time five mining villages were established in an only 5 km radius around Spindelbach, human-induced deforestation likely affected the tree species composition even in the broader area. In the period about 1450–1480 CE, the village declined and finally was abandoned. Its desertion corresponded to an epoch of regional economic and demographic stagnation, overexploitation of the mountain forests, and an era of harmful weather events (Houfková et al., 2019), which are probably connected with the onset of the Little Ice Age. Immediately after abandonment, forest regeneration started again, reflected by a renewed increase in Picea (max 10 %), and together with Betula (max 20 %) formed a secondary forest. The sum of tree pollen soon reached a maximum of ca. 60 %. Even though Picea was restored by natural succession, Fagus and Abies never achieved their former abundance, attaining relative maxima of ca. 7 % and 2 %, respectively. In the mountains, Picea is generally considered a pioneer tree species that benefits from sites that have previously been cleared by humans, while the natural re-establishment of Fagus and Abies requires more sensitive conditions (e.g. close proximity to parent trees, microclimatic protection, shade; Ellenberg, 1996).
The reflection of the local deforestation of a largely still-native forest in the high-lying profile Spindelbach, which dates back to the second half of the 14th century CE, is quite late compared to the historically known medieval settlement and land-use history in the Erzgebirge at other sites (e.g. Blažek et al., 1995; Kenzler, 2012; Tolksdorf, 2018; Cappenberg et al., 2020). Forest clearing and subsequent agricultural or industrial activities (mining, metallurgy, glass, and charcoal production) can generally be expected at many other, but often much lower, locations since the second half of the 12th century CE (Fig. 12). For example, the start of silver mining in the eastern Erzgebirge at Freiberg (ca. 380 m a.s.l.) dates to the year 1168 CE (Wagenbreth and Wächtler, 1988), at Dippoldiswalde (ca. 375 m a.s.l.) to the last quarter of the 12th century CE (Schubert, 2017), and at Niederpöbel (ca. 530 m a.s.l.) to the period 1180–1240 CE (Cappenberg et al., 2020); fortifications and village settlements in the central Erzgebirge around Zöblitz (ca. 600 m a.s.l.) and in Ullersdorf (730 m a.s.l.) date from the late 12th to 13th centuries CE (Billig and Geupel, 1992; Tolksdorf et al., 2020a); and the Teufelsschloss castle in the western Erzgebirge near Eibenstock (ca. 650 m a.s.l.) dates to the late 12th century CE/around 1200 CE (Billig and Geupel, 1992).
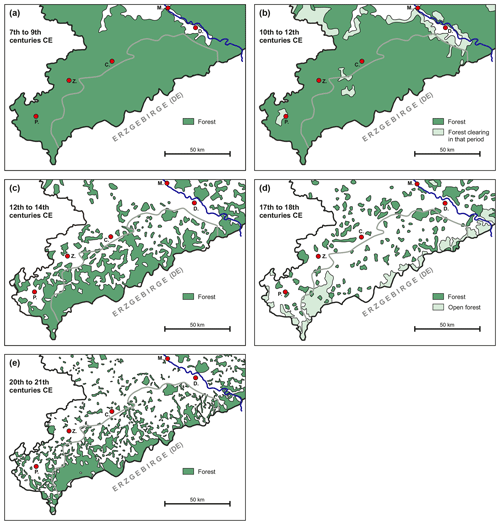
Figure 12Historical forest cover dynamics of the Erzgebirge (Saxon part) and surroundings (spatial data: Hempel, 2009, modified). (a) The 7th to 9th centuries CE, (b) 10th to 12th centuries CE, (c) 12th to 14th centuries CE, (d) 17th to 18th centuries CE, and (e) 20th to 21st centuries CE.
This early settlement/land-use period, dated independently of history and archaeology records, and the, thus, inevitable forest-clearing phase of the late 12th to 13th century CE are also represented in several pollen diagrams (IDs 32–37; Fig. 1) obtained from medieval to modern stream valley sediments around the abandoned mining town Přísečnice/Preßnitz (ca. 720 m a.s.l.), located on the Bohemian side of the central Erzgebirge (Kočár et al., 2018). These diagrams show a somewhat earlier deforestation (13th century CE) caused by agriculture and mining in comparison to the nearby pollen diagram SPI-1, Spindelbach (ID 22), described above. Although the proportions of the main tree species vary significantly in these six diagrams, they are basically the same at the moment of local clearing, namely Fagus, Abies, and Picea. In some cases, there were not a complete clearing and agricultural or industrial use of the sites but only a forest thinning with an increase in Picea and a decrease in Fagus and Abies.
Thus, based on the current knowledge from all available pollen diagrams – although these are not always available in the “right” place, and targeted palynological transect studies are also still missing – it can be assumed that in the Erzgebirge from the submontane (ca. 300–500 m a.s.l.), via the montane (ca. 500–900 m a.s.l.) to the high-montane zone (ca. 900–1200 m a.s.l.), forests with Fagus and Abies, and to a minor degree Picea, existed until the high medieval clearing. Other tree species (e.g. Acer, Ulmus, Pinus, Fraxinus, Alnus) also grew in smaller proportions on average but with potentially greater local participation. In the 20th century CE, there were still mostly small-scale locations with Fagus and Acer in the upper reaches of the Erzgebirge, i.e. up to ca. 1000–1100 m a.s.l. (Heynert, 1964). Also, isolated occurrences of Abies were still reported in the 19th–20th centuries CE up to about 1000 m a.s.l. (Firbas, 1952). This means that these tree species of the former “hercynic mixed mountain forest” (Drude, 1902) had, from today's perspective, an almost unimaginable, much wider distribution up to the High Medieval than indicated by their current sparse distribution “in the endless spruce sea”. Natural (mixed) Picea forests, however, regularly had a very small proportion at the highest altitudes (> ca. 900 m a.s.l.) and here in special locations such as wet sites or those with shallow soils as well as rocky cliffs and other sites with extreme conditions that restrict competition from other tree species (Heynert, 1964; Schmidt et al., 2002; Hempel, 2009).
Of course, for potential tree species distribution, in addition to anthropogenic processes, climatic developments during the Middle Ages and afterwards must also be considered. So far, however, there has been no regional climate reconstruction for the Erzgebirge for the last ca. 1000 years (Tolksdorf and Scharnweber, 2018). Therefore, only the quantitative reconstructions generally available for central Europe (e.g. Glaser, 2008; Büntgen et al., 2011; Luterbacher et al., 2016) can be transferred to this area. This applies to the Medieval Climate Anomaly (ca. 900–1200 CE, relatively warm) and Little Ice Age (ca. 1250–1700 CE, relatively cold) main thermal phases on the one hand and to exceptional dry (ca. 950–1700 CE, ca. 1490–1540 CE) and wet periods (ca. 1050–1150 CE, ca. 1600–1800 CE) on the other hand. At higher altitudes in particular, a combination of cooler and wetter conditions could potentially benefit Picea and disadvantage Fagus and Abies. However, historical sources show that the Erzgebirge was still suitable for the cultivation of robust field crops at the end of the Little Ice Age, even at higher altitudes. Although the upper zones of the Erzgebirge can generally be considered agriculturally unfavourable areas, cereals, potatoes, and garden fruits could be cultivated even in the rather cold 18th to 19th centuries CE (i.e. the very late part of the Little Ice Age) for subsistence economy up to the mountain crest area at around 1000 m a.s.l. (Sigismund, 1859). Even today, fields with grain, rape, and forage grass reach up to an altitude of ca. 950 m a.s.l., for example in Oberwiesenthal. Thus, the last ca. 1000 years in the Erzgebirge have most probably enabled the potential existence of the climatically more demanding Fagus, several other deciduous tree species, and Abies at all times and almost everywhere, except in mires and other extreme sites. Therefore the expansion of the climatically less demanding Picea in the Early Modern Period and afterwards, which can actually be determined in pollen diagrams and in historical sources, was a consequence of succession after repeated anthropogenic disturbance or direct planting and not of climatic cause (see from a central European perspective Seim et al., 2022).
The information given above shows that medieval vegetation dynamics have been studied in detail at least for some sites at different altitudes. For Saxony, Hempel (2009) presented a map of the “natural vegetation at around 1000 (−1200) CE” based on the state of knowledge on Holocene vegetation development available at that time (Fig. 13). It is based on geobotanical, palynological, archaeological, historical, and onomastic data. For the Erzgebirge this map shows along an altitudinal gradient Abies-dominated forests in the lower position; Fagus-dominated forests in the middle position; and Picea-dominated forests in the upper position, there together with large mire areas. In fact, these three tree species are mixed in different proportions, sometimes with a higher proportion of other trees (Acer, Pinus). Compared to the map of the potentially natural vegetation according to Schmidt et al. (2002; Fig. 3) – for a definition and discussion of both methodological concepts see, for example, Jaroslav (1998), Chiarucci et al. (2010), and Loidi et al. (2010) – in addition to similarities (Picea forests in the crest area, Fagus forests in the middle altitude), it is striking that Abies-dominated forests are mapped for the lowest mountain positions and for the northward-adjoining loess hill and glacial morainic landscape. The map of the potentially natural vegetation, however, shows Quercus–Fagus forests or Carpinus–Quercus forests, as they also occur at least on a small scale in the present. This somewhat unusual “invasion” of the mountain tree Abies in the lowlands is explained by Hempel (2009) in terms of vegetation ecology: Abies, in the mountains with somewhat similar demands as Fagus, would be a more competitive tree species on the prevailing compacted and thus seasonally waterlogged (i.e. stagnant water) loess and morainic soils in the hills and lowlands near the mountains. Geobotanical, historical, onomastic, and to a lesser extent also pollen analytical arguments (e.g. Jacob, 1971) are provided for this. In general, however, this hypothesis still needs to be verified.
6.6 Modern forest dynamics
While at least some local pollen records cover the Middle Ages and the Early Modern Period, pollen data from the past ca. 300 years are largely missing. On the one hand, this has to do with the obviously little palynological interest in this period so far. On the other hand, younger peat layers were lost due to drainage and subsequent oxidation in almost all Erzgebirge mires. This means that the last few centuries often evade potential analysis in mires.
One of the few studies attempting to palynologically resolve the last few hundreds of years is the one from the Ocean Bog mire (ID 75, 900 m a.s.l.; Fig. 1) in the western part of the Erzgebirge in Bohemia (Novak et al., 2008). This pollen diagram, only 36 cm thick and dated using 210Pb, has been interpreted to show a (near-)natural (or already regenerated?) forest at the end of the 17th/beginning of the 18th century CE, consisting of Fagus, Abies, and Picea, as well as other tree species (e.g. Pinus, Betula, Alnus). The sum of tree pollen in that time reached 90 %. In the first half of the 19th century CE there was a shift from a closed- to an open-canopy forest (arboreal pollen– AP – ca. 70 %) caused by local human impact. Fagus and Abies almost disappeared, and Picea increased sharply. The strongest open character was reached around the beginning of the 20th century CE (AP ca. 45 %). More recent events, such as the forest dieback in the second half of the 20th century CE (decreasing Picea), the emergence of invasive plant species (detection of Ambrosia), and local wetland regeneration (increasing Sphagnum) are also reflected very well in this diagram. This example underlines the potential of high-resolution pollen analysis of young peat layers in at least some Erzgebirge peatlands.
From the Early Modern Period, the proportion and diversity of synanthropic taxa increased significantly compared to the Middle Ages. Of course, the altitude must be considered here because the lower altitudes (< 500 m a.s.l.) are climatically more suitable than the upper altitudes (> 500 m a.s.l.) for diversified agriculture with a correspondingly richer use of cultivated plants. The sum of synanthropic taxa reaches up to ca. 10 % maximum in higher altitudes and 15 %–20 % maximum in the lower altitudes, respectively. In addition, there is a wider spectrum of palynologically proven plants (e.g. Cannabis, Linum). Forest management from the 18th century CE onwards (Thomasius, 1994) also left traces in the pollen precipitation. On the one hand, the proportions of Picea and Pinus, as tree species that are particularly intensively planted in the region, increased significantly (Picea max 67 %; Pinus max 50 %). On the other hand, single pollen from new tree species imported from other regions were often found (e.g. Larix, Juglans, Pseudotsuga, Castanea).
The settlement and industrial development in the Erzgebirge, particularly mining and metallurgy, caused a massive consumption of wood resources, especially from the turn of the 15th/16th centuries CE onwards. At the same time, the human population grew, many mining towns were founded, and more forest areas were cleared for agricultural use (e.g. Wilsdorf et al., 1960; Thomasius, 1994; Cembrzyński, 2019; Claire, 2022). This leads to a drastic reduction in the forest area up to the mountain crest. The minimum of the forest area was reconstructed for the late 17th to 18th centuries CE (Hempel, 2009; Fig. 12). During this time, the highest mountain top in the Saxon Erzgebirge, the Fichtelberg (1215 m a.s.l.), was also forest-free (Sächsisches Staatsministerium für Umwelt und Landwirtschaft, 2008). However, this development was neither temporally nor spatially homogeneous at the expense of the forest area. An economic crisis hit the region already as early as the 14th century CE (Derner et al., 2016), and the Thirty Years' War during the first half of the 17th century CE also led in phases to a decrease in the pressure of use and to a renewed increase in forest cover (Wetzel, 2010). In these phases, pioneer forests were able to develop again in areas formerly used for agriculture or industry, which was accompanied by an increase in, for example, Picea and Betula in pollen diagrams. Larger areas of forest were probably still present in the western Erzgebirge in the late Middle Ages (Hempel, 2009). From the 18th to the 20th century CE, government measures in particular led to a re-expansion of the forest areas in the entire region, in particular through the establishment of Picea monocultures (Fig. 12). Forest losses due to emission damages in the 1960s to 1990s, mainly in the crest region, have since been compensated for. On the Bohemian side alone ca. 40 000 ha was reforested (Šrámek et al., 2008).
However, the current and coming years' climate-change-related forest damages, especially at Picea (due to droughts and subsequent bark beetle infestation), could surpass anything documented from the 20th century CE in the Erzgebirge (Kupková et al., 2018; SMEKUL, 2020; Gdulová et al., 2021). This regional development fits into the dynamics for central Europe with a huge loss of Picea stands since the 2010s (e.g. Neumann et al., 2017; Bałazy et al., 2019; Schuldt et al., 2020). In Germany alone, the area with tremendous tree losses amounts to ca. 500 000 ha between 2018 and 2021, which corresponds to ca. 5 % of the German forest cover (DLR German Aerospace Center, 2022).
Efforts have been made in the Erzgebirge for decades to achieve ecological forest conversion, mainly including the conversion of Picea monocultures to mixed stands involving Fagus, other deciduous trees, Abies, and Picea (Eisenhauer and Sonnemann, 2009; Staatsbetrieb Sachsenforst, 2017). On the one hand, however, the speed of active forest conversion is currently not keeping pace with the dying of forest stands. On the other hand, a lack of mother trees for deciduous trees and Abies as well as excessive game populations slow down natural forest change (Fuchs et al., 2021). In the future, Fagus, other deciduous trees, and possibly also Abies in the Erzgebirge will probably benefit from the rising temperatures resulting from climate change and expand upwards. Picea, however, will probably continue to come under massive pressure to exist, especially due to drought stress and competition from other tree species, and will even disappear over large areas in the lower altitudes as a still-dominant tree (e.g. Kölling et al., 2007; Kašpar et al., 2021).
Understanding the status and anticipating possible future changes in central European mountain forests are facilitated by a systematic historical perspective. For the Erzgebirge, the review of available pollen records provides insights into the vegetation history both on a millennial scale (i.e. whole Holocene) and on a centennial scale (i.e. last centuries). The 121 pollen diagrams compiled for this represent an exceptionally high data density for a low mountain range in central Europe. On the one hand, this results from a remarkably long palynological research tradition since the 1920s. On the other hand, projects on environmental history in particular have led to a significant increase in pollen data since the 1990s. From the evaluation, analysis, and discussion of the regional data, we draw the following conclusions.
Although all pollen diagrams may help to reveal the presence/absence of the major tree taxa, only the more recent diagrams also ensure a resolution of the other taxa categories (i.e. rare trees, shrubs, herbs, grasses, spores). To date, only a few diagrams have chronological control by radiocarbon ages. This makes it necessary to create, in the future, more taxonomically and chronologically high-resolution as well as radiometrically well-dated pollen diagrams in the region, preferably along site sequences/toposequences using larger peatlands.
The six main regional vegetation phases identified for the Holocene (Betula–Pinus phase, Corylus phase, Picea phase, Fagus–Picea phase, Abies–Fagus–Picea phase, anthropogenic vegetation phase) largely correspond to existing older schemes for the Erzgebirge and other low mountain ranges in northern central Europe.
For four vegetation phases, clear differences in the proportion of the main tree taxa can be seen on the basis of the present analyses with regard to both the soil and the altitude. For example, in the Abies–Fagus–Picea phase (ca. 4500–1000 cal yr BP) on the prevailing loamy soils and up to about 1000 m a.s.l., Abies (max ca. 50 %) dominates followed by Fagus (max ca. 40 %). During most times pollen proportions of Picea were the highest in the highest altitudes, i.e. above ca. 1000 m a.s.l.
Some pollen diagrams located up to an altitude of ca. 900 m a.s.l. show the presence or even continuous curves of potential pasture and meadow indicators from around 2000 cal BCE at the earliest. Even cereal pollen occurs sporadically already before the High Medieval. However, these signals cannot (currently) be interpreted as unequivocal indicators of widespread prehistoric land use, since no supplementing studies on the influence of a potential long-distance pollen input are available from the area. However, archaeological and geochemical findings show that local mining and metallurgical activities took place even at higher altitudes in the Erzgebirge in the Bronze Age and the Iron Age.
The pollen records show that immediately before the extensive clearing in the High Middle Ages (12th/13th centuries CE) from the submontane (ca. 300–500 m a.s.l.), via the montane (ca. 500–900 m a.s.l.) to the high-montane zone (> ca. 900 m a.s.l.), mixed forests of Fagus and Abies with a certain proportion of Picea grew in most sites in the Erzgebirge. Other tree species (e.g. Acer, Ulmus, Pinus, Fraxinus, Alnus) also grew in smaller proportions on average but with potentially greater local participation. Natural (mixed) Picea forests, however, regularly had a very small proportion only at the highest altitudes (> ca. 900 m a.s.l.).
Despite the large number of pollen diagrams, the moment of local clearing (late 12th to mid-14th centuries CE) and the development of replacement vegetation has so far only been precisely dated and botanically reflected in detail in a few cases. Although the proportions of the main tree species vary significantly in these diagrams, they are basically the same at the moment of clearing, namely Fagus, Abies, and Picea. In some cases, there was not a complete clearing with following agricultural, settlement, or industrial use of the sites but only a forest thinning with an increase in Picea and a decrease in Fagus and Abies.
A few records make it clear that, despite modern disturbances in the geoarchives due to peat extraction, drainage, or overbuilding, there is a high palynological potential for in-depth research also into the more recent vegetation and environmental history of the region, i.e. covering the last ca. 300 years.
Data relating to this paper can be found in the Supplement or are available from the corresponding author upon reasonable request.
The supplement related to this article is available online at: https://doi.org/10.5194/egqsj-72-127-2023-supplement.
This study was designed by KK and MT. KK and MT wrote the paper; FH provided contributions. All authors read, commented on, and approved the paper.
The contact author has declared that none of the authors has any competing interests.
Publisher’s note: Copernicus Publications remains neutral with regard to jurisdictional claims in published maps and institutional affiliations.
The authors would like to thank Inge Eckert (Freiberg) for creating Fig. 1. To estimate PPEs from a mountainous area with the ROPES approach, Petr Kuneš (Prague) kindly provided pollen data from the Černé jezero pollen record (Šumava). We owe proofreading of a previous draft of the paper to Mary Teresa Lavin-Zimmer (Potsdam). We would like to thank Jutta Lechterbeck (Stavanger) and the anonymous reviewer for reviewing our paper. Furthermore, we would like to thank a really large number of German and Czech colleagues from the fields of botany, geosciences, archaeology, and forest sciences who with their findings, discussions, and inspirations made this review paper possible. With particular gratitude, we think of all those who have been researching the Holocene landscape history of the Erzgebirge with great dedication and success for over a 100 years. Our contribution is based on the solid foundation they have laid.
Publication costs of the paper were supported within the funding programme Open-Access-Publikationskosten of the Deutsche
Forschungsgemeinschaft (DFG) (grant no. 491075472).
The article processing charges for this open-access publication were covered by the Helmholtz Centre Potsdam – GFZ German Research Centre for Geosciences.
This paper was edited by Ingmar Unkel and reviewed by Jutta Lechterbeck and one anonymous referee.
Abraham, V.: Palynological synthesis for the Czech Republic, Ph.D. thesis, Charles University in Prague, Faculty of Science, Department of Botany, 163 pp., 2014.
Abraham, V., Kuneš, P., Petr, L., Svitavská-Svobodová, H., Kozáková, R., Jamrichová, E., Švarcová, M. G., and Pokorný, P.: A pollen-based quantitative reconstruction of the Holocene vegetation updates a perspective on the natural vegetation in the Czech Republic and Slovakia, Preslia, 88, 409–434, https://www.preslia.cz/P164Abraham.pdf (last access: 25 April 2022), 2016.
Abraham, V., Man, M., Theuerkauf, M., Pokorný, P., Bobek, P., and Novák, J.: Spatially explicit, quantitative reconstruction of past vegetation based on pollen or charcoal data as a tool for autecology of trees, Landscape Ecol., 38, 1747–1763, https://doi.org/10.1007/s10980-023-01652-8, 2023.
Achterberg, I., Frechen, M., Bauerochse, A., Eckstein, J., and Leuschner, H. H.: The Göttingen tree-ring chronologies of peat-preserved oaks and pines from Northwest Germany, Z. Dtsch. Ges. Geowiss., 168, 9–19, https://doi.org/10.1127/zdgg/2016/0042, 2016.
Bałazy, R., Zasada, M., Ciesielski, M., Waraksa, P., and Zawiła-Niedźwiecki, T.: Forest dieback processes in the Central European Mountains in the context of terrain topography and selected stand attributes, For. Ecol. Manage., 435, 106–119, https://doi.org/10.1016/j.foreco.2018.12.052, 2019.
Bartelheim, M. and Niederschlag, E.: Ein Befund zur vorgeschichtlichen Zinngewinnung im Erzgebirge?, Archäologie Aktuell, 4, 60–66, 1997.
Bednářová, E., Böhm, A.-K., Feske, N., Gemballa, R., Hahn, A., Hájková, L., Hanel, M., Hoy, A., Krofta, K., Küchler, W., Kučera, J., Kyselý, J., Lippert, S.-A., Petzold, R., Schöder, L., Seják, J., Skalák, P., Štěpánek, P., Urban, A., Vizina, A., and Zahradniček, P.: Der Klimawandel im böhmisch-sächsischen Grenzraum, Změna klimatu v česko-saském pohraničí, Sächsisches Landesamt für Umwelt, Landwirtschaft und Geologie, Dresden, 140 pp., 2014.
Behre, K.-E.: Landschaftsgeschichte Norddeutschlands, Umwelt und Siedlung von der Steinzeit bis zur Gegenwart, Wachholtz, Neumünster, ISBN 3529024996, 300 pp., 2008.
Behre, K.-E., Brande, A., Küster, H., and Rösch, M.: Germany, in: Palaeoecological Events During the Last 15 000 Years: Regional Syntheses of Palaeoecological Studies of Lakes and Mires in Europe, edited by: Berglund, B. E., Birks, H. J. B., Ralska-Jasiewiczowa, M., and Wright, H. E., Wiley, Chichester, 507–551, ISBN 0471958409, 1996.
Bell, M.: Making one's way in the world: the footprints and trackways of prehistoric people, Oxbow Books, Oxford, https://doi.org/10.2307/j.ctv138wsp4, 2020.
Beug, H.-J.: Franz Firbas, 1902–1964, Taxon 14, 77–83, https://www.jstor.org/stable/1216456 (last access: 15 March 2022), 1965.
Beug, H.-J.: Zum Gedenken an Franz Firbas (1902-1964) anlässlich seines 100. Geburtstages, E&G Quaternary Sci. J., 52, 1–3, https://doi.org/10.3285/eg.52.1.01, 2003.
Beug, H.-J., Henrion, I., and Schmüser, A.: Landschaftsgeschichte im Hochharz, Die Entwicklung der Wälder und Moore seit dem Ende der letzten Eiszeit, Papierflieger, Clausthal-Zellerfeld, ISBN 3897202565, 454 pp., 1999.
Bičík, I. and Štěpánek, V.: Post-war changes of the land-use structure in Bohemia and Moravia: Case study Sudetenland, GeoJournal, 32, 253–259, https://doi.org/10.1007/BF01122117, 1994.
Billig, G. and Geupel, V.: Entwicklung, Form und Datierungen der Siedlungen in der Kammregion des Erzgebirges, Siedlungsforschung: Archäologie – Geschichte – Geographie, 10, 173–194, 1992.
Birks, H. J. B. and Seppä, H.: Late-Quaternary palaeoclimatic research in Fennoscandia – A historical review, Boreas, 39, 655–673, https://doi.org/10.1111/j.1502-3885.2010.00160.x, 2010.
Blažek, J., Černá, E., and Velímský, T.: Zur Siedlungsgeschichte der böhmischen Seite des Erzgebirges, Germania, 73, 463–479, https://doi.org/10.11588/ger.1995.92672, 1995.
Bobek, P., Svobodová-Svitavská, H., Pokorný, P., Šamonil, P., Kuneš, P., Kozáková, R., Abraham, V., Klinerová, T., Švarcová, M. G., Jamrichová, E., Krauseová, E., and Wild, J.: Divergent fire history trajectories in Central European temperate forests revealed a pronounced influence of broadleaved trees on fire dynamics, Quaternary Sci. Rev., 222, 105865, https://doi.org/10.1016/j.quascirev.2019.105865, 2019.
Bohdálková, L., Bohdálek, P., Břízová, E., Pacherová, P., and Kuběna, A. A.: Atmospheric metal pollution records in the Kovářská Bog (Czech Republic) as an indicator of anthropogenic activities over the last three millennia, Sci. Total Environ., 633, 857–874, https://doi.org/10.1016/j.scitotenv.2018.03.142, 2018.
Böhm, A. K.: Hochmoore im Erzgebirge – Untersuchungen zum Zustand und Stoffaustragsverhalten unterschiedlich degradierter Flächen, Ph.D. thesis, Technische Universität Dresden, 200 pp., 2006.
Bramer, H., Hendl, M., Marcinek, J., Nitz, B., Ruchholz, K., and Slobodda, S.: Physische Geographie. Mecklenburg-Vorpommern, Brandenburg, Sachsen-Anhalt, Sachsen, Thüringen, Hermann Haack, Gotha, ISBN 3730108859, 627 pp., 1991.
Brízová, E.: Reconstruction of the vegetational evolution of the Bozí Dar peat bog during Late Glacial and Holocene, Geolines, 2, p. 10, 1995.
Brízová, E.: Das Erzgebirge – Umwelt und Bergbau. in: Silberrausch und Berggeschrey, Archäologie des mittelalterlichen Bergbaus in Sachsen und Böhmen, edited by: Smolnik, R., Beier and Beran, Unterweißbach, 185–192, ISBN 3941171992, 2014a.
Brízová, E.: Erzgebirgische Moorgebiete als ein Archiv zum Studium der menschlichen Auswirkungen auf die Natur, in: ArchaeoMontan 2013. Krusna krajina – Erz(gebirgs)-landschaft – Ore Landscape. Internationale Fachtagung Kadaň 26. bis 28. September 2013, edited by: Smolnik, R., Arbeits- und Forschungsberichte zur sächsischen Bodendenkmalpflege Beiheft, 28, 199–207, Dresden, ISBN 978-3-943770-14-8, 2014b.
Bronk Ramsey, C.: Methods for summarizing radiocarbon datasets, Radiocarbon, 59, 1809–1833, https://doi.org/10.1017/RDC.2017.108, 2017.
Büntgen, U., Tegel, W., Nicolussi, K., McCormick, M., Frank, D., Trouet, V., Kaplan, J. O., Herzig, F., Heussner, K.-U., Wanner, H., Luterbacher, J., and Esper, J.: 2500 years of European climate variability and human susceptibility, Science, 331, 578–582, https://doi.org/10.1126/science.1197175, 2011.
Cappenberg, K., Schubert, M., and Hemker, C.: Landschaftsveränderungen in Montanregionen, Mindestens 900 Jahre “human impact” im Erzgebirge, Mitteilungen der Deutschen Gesellschaft für Archäologie des Mittelalters und der Neuzeit, 33, 179–192, https://doi.org/10.11588/dgamn.2020.0.77903, 2020.
Carter, V. A., Chiverrell, R. C., Clear, J. L., Kuosmanen, N., Moravcová, A., Svoboda, M., Svobodová-Svitavská, H., van Leeuwen, J. F. N., van der Knaap, W. O., and Kuneš, P.: Quantitative palynology informing conservation ecology in the Bohemian/Bavarian forests of Central Europe, Front. Plant Sci., 8, 2268, https://doi.org/10.3389/fpls.2017.02268, 2018.
Cembrzyński, P.: The ecology of mining, Human-environmental relations in the Medieval and Early Modern mining in Central Europe, Kwartalnik Historii Kultury Materialnej, 67, 17–39, https://doi.org/10.23858/KHKM67.2019.1.002, 2019.
Chiarucci, A., Araújo, M. B., Decocq, G., Beierkuhnlein, C., and Fernández-Palacios, J. M.: The concept of potential natural vegetation: an epitaph?, J. Veg. Sci., 21, 1172–1178, https://doi.org/10.1111/j.1654-1103.2010.01218.x, 2010.
Christl, A. and Simon, K.: Nutzung und Besiedlung des sächsischen Erzgebirges und des Vogtlandes bis zur deutschen Ostkolonisation, Germania, 73, 441–479, 1995.
Claire, S.: The Ore Mountains mining area in Bohemia: A reservoir of silver resources in Central Europe in the sixteenth century, Global Environ., 15, 47–70, https://doi.org/10.3197/ge.2022.150103, 2022.
Conedera, M., Tinner, W., Neff, C., Meurer, M., Dickens, F. A., and Krebs, F.: Reconstructing past fire regimes: methods, applications, and relevance to fire management and conservation, Quaternary Sci. Rev., 28, 555–576, https://doi.org/10.1016/j.quascirev.2008.11.005, 2009.
Derner, K. (Ed.): Středověké hornictvi a hutnictvi na Přisecnicku ve středním Krušnohoří. Mittelalterlicher Bergbau und Hüttenwesen in der Region Pressnitz im mittleren Erzgebirge, Veröffentlichungen des Landesamtes für Archäologie Sachsen, 68, Landesamt für Archäologie Sachsen, Dresden, ISBN 978-3-943770-40-7, 496 pp., 2018.
Derner, K., Hrubý, P., and Schubert, M.: Mittelalterliche Silberproduktion im wettinischen und premyslidischen Regierungsraum: Neue archäologische Untersuchungen, Der Anschnitt, Z. Kunst Kultur im Bergbau, 68, 216–241, 2016.
DLR German Aerospace Center: Concern about German forests. Satellite data reveal extensive losses in the forest inventory, 21 February 2022, https://www.dlr.de/content/en/articles/news/2022/01/20220221_concern-about-german-forests.html (last access: 30 December 2022), 2022.
Dreslerová, D., Kozáková, R., Metlička, M., Brychová, V., Bobek, P., Čišecký, Č., Demján, P., Lisá, L., Pokorná, A., Michálek, J., Strouhalová, B., and Trubač, J.: Seeking the meaning of a unique mountain site through a multidisciplinary approach. The Late La Tène site at Sklářské Valley, Šumava Mountains, Czech Republic, Quaternary Int., 542, 88–108, https://doi.org/10.1016/j.quaint.2020.03.013, 2020.
Drude, O.: Der Hercynische Florenbezirk. Grundzüge der Pflanzenverbreitung im mitteldeutschen Berg- und Hügellande vom Harz bis zur Rhön, bis zur Lausitz und dem Böhmer Walde, Pflanzenverbreitung in Mitteleuropa nördlich der Alpen No. 1, Verlag von Wilhelm Engelmann, Leipzig, 671 pp., 1902.
Dudová, L., Hájek, M., Petr, L., and Jankovská, V.: Holocene vegetation history of the Jeseníky Mts: Deepening elevational contrast in pollen assemblages since late prehistory, J. Veg. Sci., 29, 371–381, https://doi.org/10.1111/jvs.12612, 2018.
Edom, F., Dittrich, I., Keßler, K., Münch, A., Peters, R., Theuerkauf, M., and Wendel, D.: Klimatische Stabilität von Mittelgebirgsmooren. Auswirkungen des Klimawandels auf wasserabhängige Ökosysteme – Teilprojekt Erzgebirgsmoore, Schriftenreihe des LfULG, Heft 1/2011, Sächsisches Landesamt für Umwelt, Landwirtschaft und Geologie, Dresden, 1–80, 2011.
Eisenhauer, D. R. and Sonnemann, S.: Waldbaustrategien unter sich ändernden Umweltbedingungen – Leitbilder, Zielsystem und Waldentwicklungstypen, Waldökologie, Landschaftsforschung und Naturschutz, 8, 71–88, 2009.
Ellenberg, H.: Vegetation Mitteleuropas mit den Alpen in ökologischer, dynamischer und historischer Sicht, Ulmer, Stuttgart (5th Edition), ISBN 3800134284, 1095 pp., 1996.
Firbas, F.: Spät- und nacheiszeitliche Waldgeschichte Mitteleuropas nördlich der Alpen. Erster Band: Allgemeine Waldgeschichte, Gustav Fischer, Jena, 480 pp., 1949.
Firbas, F.: Spät- und nacheiszeitliche Waldgeschichte Mitteleuropas nördlich der Alpen. Zweiter Band: Waldgeschichte der einzelnen Landschaften, Gustav Fischer, Jena, 256 pp., 1952.
Firbas, F., Münnich, K. O., and Wittke, W.: C14-Datierungen zur Gliederung der nacheiszeitlichen Waldentwicklung und zum Alter von Rekurrenzflächen im Fichtelgebirge, Flora, 146, 512–520, 1958.
Frenzel, H.: Entwicklungsgeschichte der sächsischen Moore und Wälder seit der letzten Eiszeit auf Grund pollenanalytischer Untersuchungen, Abhandlungen des Sächsischen Geologischen Landesamts, 9, 1–119, 1930.
Friedmann, A., Stojakowits, P., and Korch, O.: History of vegetation and land-use change in the northern Calcareous Alps (Germany/Austria), in: Mountain Landscapes in Transition. Effects of Land Use and Climate Change, edited by: Schickhoff, U., Singh, R. B., Mal, S., Sustainable Development Goals Series, 601–612, https://doi.org/10.1007/978-3-030-70238-0_28, 2022.
Fuchs, Z., Vacek, Z., Vacek, S., and Gallo, J.: Effect of game browsing on natural regeneration of European beech (L.) forests in the Krušné hory Mts. (Czech Republic and Germany), Central European Forestry Journal, 67, 166–180, https://doi.org/10.2478/forj-2021-0008, 2021.
Gdulová, K., Marešová, J., Barták, V., Szostak, M., Cervenka, J., and Moudrý, V.: Use of TanDEM-X and SRTM-C data for detection of deforestation caused by bark beetle in central European mountains, Remote Sens., 13, 3042, https://doi.org/10.3390/rs13153042, 2021.
Giesecke, T. and Brewer, S.: Notes on the postglacial spread of abundant European tree taxa, Veg. Hist. Archaeobot., 27, 337–349, https://doi.org/10.1007/s00334-017-0640-0, 2018.
Giesecke, T., Bennett, K. D., Birks, H. J. B., Bjune, A. E., Bozilova, E., Feurdean, A., Finsinger, W., Froyd, C., Pokorný, P., Rösch, M., Seppä, H., Tonkov, S., Valsecchi, V., and Wolters, S.: The pace of Holocene vegetation change – testing for synchronous developments, Quaternary Sci. Rev., 30, 2805–2814, https://doi.org/10.1016/j.quascirev.2011.06.014, 2011.
Giesecke, T., Wolters, S., Jahns, S., and Brande, A.: Exploring Holocene changes in palynological richness in northern Europe – Did Postglacial immigration matter?, PLoS ONE, 7, e51624, https://doi.org/10.1371/journal.pone.0051624, 2012.
Giesecke, T., Brewer, S., Finsinger, W., Leydet, M., and Bradshaw, R. H.: Patterns and dynamics of European vegetation change over the last 15,000 years, J. Biogeogr., 44, 1441–1456, https://doi.org/10.1111/jbi.12974, 2017.
Glaser, R.: Klimageschichte Mitteleuropas: 1200 Jahre Wetter, Klima, Katastrophen, Wissenschaftliche Buchgesellschaft, Darmstadt, ISBN 3896786040, 277 pp., 2008.
Grober, U.: Sustainability: A Cultural History, Green Books, Totnes/Devon, ISBN 0857840452, 224 pp., 2012.
Gross, H.: Zwei bemerkenswerte begrabene Moorböden aus dem Gebiet von Hainichen in Sachsen, Berichte der Geologischen Gesellschaft, 3, 209–218, 1958.
Großer, K. H., Wolters, S., and Schaarschmidt, J.: Das Hochmoor bei Jahnsgrün im Erzgebirge, Naturschutzarbeit in Sachsen, 48, 41–52, 2006.
Grunewald, K. and Bastian, O.: Ecosystem assessment and management as key tools for sustainable landscape development: a case study of the Ore Mountains region in Central Europe, Ecol. Model., 295, 151–162, https://doi.org/10.1016/j.ecolmodel.2014.08.015, 2015.
Hahne, J.: Untersuchungen zur spät und postglazialen Vegetationsgeschichte im nordöstlichen Bayern (Bayerisches Vogtland, Fichtelgebirge, Steinwald), Flora, 187, 169–200, 1992.
Hansell, F.: Mineral extractive industries in the context of European world heritage cultural landscape conservation and management: The case study of the Erzgebirge/Krušnohoří mining region, in: 50 Years World Heritage Convention: Shared Responsibility – Conflict & Reconciliation, edited by: Albert, M. T., Bernecker, R., Cave, C., Prodan, A. C., and Ripp, M., Heritage Studies, Springer, Cham, 321–333, https://doi.org/10.1007/978-3-031-05660-4_25, 2022.
Hauswald, K. and Simon, K.: Der Kulmer Steig vor dem Mittelalter. Zu den ältesten sächsisch-böhmischen Verkehrswegen über das Osterzgebirge, Arbeits- und Forschungsberichte zur sächsischen Bodendenkmalpflege, 37, 9–98, 1995.
Heinrich, W. and Lange, E.: Ein Beitrag zur Kenntnis der Waldgeschichte des Thüringisch-Sächsischen Vogtlandes, Feddes Repertorium, 80, 437–462, 1969.
Hemker, C.: New insights on mining archaeology in the Erzgebirge (Ore Mountains), World of Mining – Surface & Underground, 70, 409–416, 2018.
Hempel, W.: Die Pflanzenwelt Sachsens von der Späteiszeit bis zur Gegenwart, Weissdorn-Verlag, Jena, ISBN 9783936055573, 248 pp., 2009.
Hengst, K.: Erzgebirge/Krušné hory im Spiegel der Sprache seit etwa 3000 Jahren. “Hercynia silva – Fergunna – Miriquidi – Böhmerwald – Erzgebirge”. in: Erzgebirge – Heimat und domov. 8. Deutsch-Tschechischen Begegnungsseminar Gute Nachbarn – Schlechte Nachbarn?, edited by: Mehnert, E. and Lang, P., Frankfurt am Main, 71–79, ISBN 3631550278, 2006.
Henkner, J., Ahlrichs, J., Fischer, E., Fuchs, M., Knopf, T., Rösch, M., Scholten, T., and Kühn, P.: Land use dynamics derived from colluvial deposits and bogs in the Black Forest, Germany, J. Plant Nutr. Soil Sci., 18, 240–260, https://doi.org/10.1002/jpln.201700249, 2018.
Herbig, C.: “O mensch zart, bedenck der blumen art”. Pflanzliche Großreste aus archäologischen Befunden als Indizien für Ernährung, Landwirtschaft und Umwelt in mittelalterlichen Bergstädten im Erzgebirge, in: Leben und Tod in einer Bergstadt. Die Welterbe-Bestandteile Dippoldiswalde, Freiberg, Annaberg und Marienberg im Spiegel archäologischer, archäobotanischer und anthropologischer Studien, edited by: Smolnik, R., Veröffentlichungen des Landesamtes für Archäologie Sachsen, 78, Landesamt für Archäologie Sachsen, Dresden, 137–167, ISBN 978-3-943770-63-6, 2022.
Heynert, H.: Zur Ursprünglichkeit des Tannen-Höhenkiefernwaldes im westlichsten Sächsischen Erzgebirge und seinem Vorlande, Drudea, 1, 5–24, 1961.
Heynert, W.: Das Pflanzenleben des hohen Westerzgebirges. Ein Beitrag zur Geobotanik des Westerzgebirges, Theodor Steinkopff, Dresden and Leipzig, 141 pp., 1964.
Hoffmann, Y. and Heußner, K.-U.: Die Errichtung der romanischen Kirche zu Raschau und der Zeitpunkt der bäuerlichen Kolonisation im oberen Erzgebirge, Sächsische Heimatblätter, 59, 253–261, 2013.
Hölzer, A. and Hölzer, A.: Untersuchungen zum Rezentpollenniederschlag im Nordschwarzwald im Bereich der Hornisgrinde, standort.wald – Mitteilungen des Vereins für forstliche Standortskunde und Forstpflanzenzüchtung, 48, 63–76, 2014.
Houfková, P., Bešta, T., Bernardová, A., Vondrák, D., Pokorný, P., and Novák, J.: Holocene climatic events linked to environmental changes at Lake Komořany Basin, Czech Republic, The Holocene, 27, 1–14, https://doi.org/10.1177/0959683616683250, 2017.
Houfková, P., Horák, H., Pokorná, A., Bešta, T., Pravcová, I., Novák, J., and Klír, T.: The dynamics of a non-forested stand in the Krušné Mts.: the effect of a short-lived medieval village on the local environment, Veg. Hist. Archaeobot., 28, 607–621, https://doi.org/10.1007/s00334-019-00718-5, 2019.
Hrubý, P.: Erzbergbau und Edelmetallproduktion im böhmischen Königreich während des 13. Jhs. im Kontext der europäischen Montanarchäologie, Veröffentlichungen des Landesamtes für Archäologie Sachsen, 71, Landesamt für Archäologie Sachsen, Dresden, ISBN 978-3-943770-55-1, 248 pp., 2021.
Hrubý, P., Hejhal, P., Malý, K., Kočár, P., and Petr, L.: Centrální Českomoravská vrchovina na prahu vrcholného středověku: archeologie, geochemie a rozbory sedimentárních výplní niv, Masarykova Univerzita, Brno, ISBN 978-80-210-7126-1, 270 pp., 2014.
ICOMOS: International Council on Monuments and Sites: Advisory Body Evaluation. Erzgebirge/Krušnohoří (Germany/Czechia) No 1478, https://whc.unesco.org/en/list/1478/documents/ (last access: 27 January 2022), 2019.
Jacob, H.: Waldgeschichtliche Untersuchungen im Tharandter Gebiet, Feddes Repertorium Beiheft, 137, 183–275, 1956.
Jacob, H.: Pollenanalysen aus dem Gebiet des ehemaligen Göttwitzer Sees bei Mutzschen, Kr. Grimma. Ein Beitrag zur Entwicklung von Vegetation und Klima seit der Bronzezeit, Arbeits- und Forschungsberichte zur sächsischen Bodendenkmalpflege, 19, 159–175, 1971.
Jankovská, V.: Pollenanalysen der mittelalterlichen Ablagerungen in dem Moster Gebiet. Památky Archeologické, 86, 132–154, 1995.
Jankovská, V. and Pokorný, P.: Reevaluation of the palaeoenvironmental record of the former Komořanské jezero lake: Late-Glacial and Holocene palaeolimnology and vegetation development in north-western Bohemia, Czech Republic, Preslia, 85, 265–287, 2013.
Jankovská, V., Kunes, P., and van der Knaap, W. O.: Flaje-Kiefern (Krušné Hory Mountains): Late Glacial and Holocene vegetation development, Grana, 46, 214–216, https://doi.org/10.1080/00173130701526341, 2007.
Jaroslav, M.: Reconstructed natural versus potential natural vegetation in vegetation mapping-a discussion of concepts, Appl. Veg. Sci., 1, 173–176, https://doi.org/10.2307/1478946, 1998.
Jouffroy-Bapicot, I., Vannière, B., Gauthier, É., Richard, H., Monna, F., and Petit, C.: 7000 years of vegetation history and land-use changes in the Morvan Mountains (France): A regional synthesis, The Holocene, 23, 1888–1902, https://doi.org/10.1177/0959683613508161, 2013.
Kaiser, K., Hrubý, P., Tolksdorf, J. F., Alper, G., Herbig, C., Kočár, P., Petr, L., Schulz, L., and Heinrich, I.: Cut and covered: Subfossil trees in buried soils reflect medieval forest composition and exploitation of the central European uplands, Geoarchaeology, 35, 42–62, https://doi.org/10.1002/gea.21756, 2020a.
Kaiser, K., Schneider, T., Küster, M., Dietze, E., Fülling, A., Heinrich, S., Kappler, C., Nelle, O., Schult, M., Theuerkauf, M., Vogel, S., de Boer, A. M., Börner, A., Preusser, F., Schwabe, M., Ulrich, J., Wirner, M., and Bens, O.: Palaeosols and their cover sediments of a glacial landscape in northern central Europe: Spatial distribution, pedostratigraphy and evidence on landscape evolution, Catena, 193, 104647, https://doi.org/10.1016/j.catena.2020.104647, 2020b.
Kaiser, K., Tolksdorf, J. F., de Boer, A. M., Herbig, C., Hieke, F., Kasprzak, M., Kočár, P., Petr, L., Schubert, M., Schröder, F., Fülling, A., and Hemker, C.: Colluvial sediments originating from past land-use activities in the Erzgebirge Mountains, Central Europe: occurrence, properties and historic environmental implications, Archaeol. Anthropol. Sci., 13, 220, https://doi.org/10.1007/s12520-021-01469-z, 2021.
Kašpar, J., Tumajer, J., Šamonil, P., and Vašíčková, I.: Species-specific climate-growth interactions determine tree species dynamics in mixed Central European mountain forests, Environ. Res. Lett., 16, 034039, https://doi.org/10.1088/1748-9326/abd8fb, 2021.
Käubler, R.: Vergleichende Betrachtungen zu geomorphologischen Ergebnissen im höchsten Teil des Erzgebirges, Hercynia, 6, 109–114, 1969.
Kenzler, H.: Die hoch- und spätmittelalterliche Besiedlung des Erzgebirges: Strategien zur Kolonisation eines landwirtschaftlichen Ungunstraumes, Bamberger Schriften zur Archäologie des Mittelalters und der Neuzeit, 4. Habelt, Bonn, ISBN 978-3-7749-3742-0, 452 pp., 2012.
Kienitz, E.: Wandlung des Holzartenbildes im sächsischen Staatswalde seit dem 16. Jahrhundert, mit Ausblicken auf die Pollenanalyse (Forstinspektionsbezirke Eibenstock und Grimma), Tharandter Forstliches Jahrbuch, 87, 285–326, 413–448, 459–523, 641–690, 747–799, 824–853, 1936.
Kleber, A., Terhorst, B., Bullmann, H., Hülle, D., Leopold, M., Müller, S., Raab, T., Sauer, D., Scholten, T., Dietze, M., Felix-Henningsen, P., Heinrich, J., Spies, E.-D., and Thiemeyer, H.: Subdued mountains of Central Europe, in: Mid-Latitude Slope Deposits (Cover Beds), edited by: Kleber, A. and Terhorst, B., Developments in Sedimentology, 66, Elsevier, Amsterdam, 9–93, https://doi.org/10.1016/B978-0-444-53118-6.00002-7, 2013.
Knapp, H., Robin, V., Kirleis, W., and Nelle, O.: Woodland history in the upper Harz Mountains revealed by kiln site, soil sediment and peat charcoal analyses, Quaternary Int., 289, 88–100, https://doi.org/10.1016/j.quaint.2012.03.040, 2013.
Knapp, H. D., Klaus, S., and Fähser, L.: Der Holzweg. Wald im Widerstreit der Interessen, Oekom, München, ISBN 978-3-96238-266-7, 480 pp., 2021.
Kočár, P., Petr, L., and Kočárová, R.: Vegetationswandel im Bergrevier Preßnitz. in: Mittelalterlicher Bergbau und Hüttenwesen in der Region Preßnitz im mittleren Erzgebirge. Středověké hornictvi a hutnictvi na Přisecnicku ve středním Krušnohoří, edited by: Derner, K., Veröffentlichungen des Landesamtes für Archäologie Sachsen, 68, Landesamt für Archäologie Sachsen, Dresden, 67–78, ISBN 978-3-943770-40-7, 2018.
Kölling, C., Zimmermann, L., and Walentowski, H.: Klimawandel: Was geschieht mit Buche und Fichte?, AFZ-Der Wald, 11/2007, 584–588, 2007.
Kozáková, R., Pokorný, P., Peša, V., Danielisová, A., Čuláková, K., and Svobodová, H. S.: Prehistoric human impact in the mountains of Bohemia. Do pollen and archaeological data support the traditional scenario of a prehistoric “wilderness”?, Rev. Palaeobot. Palynol., 220, 29–43, https://doi.org/10.1016/j.revpalbo.2015.04.008, 2015.
Kozáková, R., Bobek, P., Dreslerová, D., Abraham, V., and Svobodová-Svitavská, H.: The prehistory and early history of the Šumava Mountains (Czech Republic) as seen through anthropogenic pollen indicators and charcoal data, The Holocene, 31, 145–159, https://doi.org/10.1177/0959683620961484, 2022.
Kreienbrink, F., Döhlert-Albani, N., Conrad, M., Herbig, C., Martin, I., Schubert, M., Tinapp, C., Hößler, R., Johl, S., Krämer, U., Mauksch, K., Priske, C., and Stäuble, H.: Von der Großenhainer Pflege übers Elbtal ins Erzgebirge. Die Ausgrabungen an der EUGAL, Ausgrabungen in Sachsen, 7, 134–149, 2020.
Krutzsch, H.: Waldaufbau, Deutscher Bauernverlag, Berlin, 159 pp., 1952.
Kubíková, J.: Forest dieback in Czechoslovakia, Vegetatio, 93, 101–108, https://doi.org/10.1007/BF00033204, 1991.
Kulakowski, D., Seidl, R., Holeksa, J., Kuuluvainen, T., Nagel, T. A., Panayotov, M., Svoboda, M., Thorn, S., Vacchiano, G., Whitlock, C., Wohlgemuth, T., and Bebi, P.: A walk on the wild side: Disturbance dynamics and the conservation and management of European mountain forest ecosystems, For. Ecol. Manage., 388, 120–131, https://doi.org/10.1016/j.foreco.2016.07.037, 2017.
Kupková, L., Potůčková, M., Lhotáková, Z., and Albrechtová, J.: Forest cover and disturbance changes, and their driving forces: A case study in the Ore Mountains, Czechia, heavily affected by anthropogenic acidic pollution in the second half of the 20th century, Environ. Res. Lett., 13, 095008, https://doi.org/10.1088/1748-9326/aadd2c, 2018.
Küssner, R. and Mosandl, R.: Conceptions for converting the forests in the Ore Mts. into ecologically managed ecosystems. in: SO2-pollution and forest decline in the Ore Mountains, edited by: Lomsky, B., Materna, J., and Pfanz, H., Forestry and Game Management Research Institute, Jiloviste-Strnady, Czech Republic, 326–342, 2002.
Landestalsperrenverwaltung des Freistaates Sachsen: Eine Zeitreise in die sächsische Wasserwirtschaft, Pirna, 2017.
Lang, G.: Quartäre Vegetationsgeschichte Europas, Fischer, Jena, ISBN 3334604055, 462 pp., 1994.
Lang, G.: Seen und Moore des Schwarzwaldes als Zeugen spätglazialen und holozänen Vegetationswandels. Stratigraphische, pollenanalytische und großrestanalytische Untersuchungen, Andrias, 16, Staatliches Museum für Naturkunde, Karlsruhe, 166 pp., 2005.
Lange, E. and Heinrich, W.: Floristische und vegetationskundliche Beobachtungen auf dem MTB Frankenberg/Sa. [5044], Hercynia, 7, 53–86, 1970.
Lange, E., Jeschke, L., and Knapp, H. D.: Ralswiek und Rügen. Landschaftsentwicklung und Siedlungsgeschichte der Ostseeinsel. Teil I Die Landschaftsgeschichte der Insel Rügen seit dem Spätglazial, Schriften zur Ur- und Frühgeschichte, 38, Akademie Verlag, Berlin, ISBN 311257463X, 194 pp., 1986.
Lange, E., Christl, A., and Joosten, H.: Ein Pollendiagramm aus der Mothäuser Heide im oberen Erzgebirge unweit des Grenzüberganges Reitzenhain, in: Kirche und geistiges Leben im Prozess des mittelalterlichen Landesausbaus in Ostthüringen/Westsachsen, edited by: Sachenbacher, P., Beiträge zur Frühgeschichte und zum Mittelalter Ostthüringens, 2, Beier & Beran, Langenweißbach, 153–169, ISBN 978-3-937517-04-9, 169 pp., 2005.
Lauterbach, K.: Vom Grenzwald zur Grenzlinie. Zur Entstehung der sächsisch-böhmischen Grenze, Sächsische Heimatblätter, 64, 104–108, https://doi.org/10.52410/shb.Bd.64.2018.H.2.S.104-108, 2018.
Loidi, J., del Arco, M., Pérez de Paz, P.L., Asensi, A., Díez Garretas, B., Costa, M., Díaz González, T., Fernández-González, F., Izco, J., Penas, Á., Rivas-Martínez, S., and Sánchez-Mata, D.: Understanding properly the `potential natural vegetation' concept, J. Biogeogr. 37, 2209–2211, https://doi.org/10.1111/j.1365-2699.2010.02302.x, 2010.
Ludemann, T.: Past fuel wood exploitation and natural forest vegetation in the Black Forest, the Vosges and neighbouring regions in western Central Europe, Palaeogeogr. Palaeoclimatol. Palaeoecol., 291, 154–165, https://doi.org/10.1016/j.palaeo.2009.09.013, 2010.
Luterbacher, J., Werner, J. P., Smerdon, J. E., Fernández-Donado, L., González-Rouco, F. J., Barriopedro, D., Ljungqvist, F. C., Büntgen, U., Zorita, E., Wagner, S., Esper, J., McCarroll, D., Toreti, A., Frank, D., Jungclaus, J. H., Barriendos, M., Bertolin, C., Bothe, O., Brázdil, R., Camuffo, D., Dobrovolný, P., Gagen, M., García-Bustamante, E., Ge, Q., Gómez-Navarro, J. J., Guiot, J., Hao, Z., Hegerl, G. C., Holmgren, K., Klimenko, V. V., Martín-Chivelet, J., Pfister, C., Roberts, N., Schindler, A., Schurer, A., Solomina, O., von Gunten, L., Wahl, E., Wanner, H., Wetter, O., Xoplaki, E., Yuan, N., Zanchettin, D., Zhang, H., and Zerefos, C.: European summer temperatures since Roman times, Environ. Res. Lett., 11, 024001, https://doi.org/10.1088/1748-9326/11/2/024001, 2016.
Marešová, P.: Human impact as reflected in the pollen record, with a focus on the Middle Ages, Ph.D. thesis, Thesis Series, No. 7., University of South Bohemia, Faculty of Science, School of Doctoral Studies in Biological Sciences, České Budějovice, 167 pp., 2022.
Miras, Y., Mariani, M., Ledger, P., Mayoral, A., Chassiot, L., and Lavrieux, M.: Holocene vegetation dynamics and first land-cover estimates in the Auvergne Mountains (Massif Central, France): Key tools to landscape management, Interdisciplinaria Archaeologica – Natural Sciences in Archaeology, 9, 179–190, http://iansa.eu/papers/IANSA-2018-02-miras.html (last access: 20 January 2022), 2018.
Möller, A.: Der Dauerwaldgedanke – Sein Sinn und seine Bedeutung, in: Kommentierter Reprint 1990, edited by: Bode, W., Oberteuringen, Degreif Verlag, 1922.
Morel, A. C. and Nogué, S.: Combining contemporary and paleoecological perspectives for estimating forest resilience, Front. Forests Global Change, 2, 57, https://doi.org/10.3389/ffgc.2019.00057, 2019.
Mrotzek, A., Couwenberg, J., Theuerkauf, M., and Joosten, H.: MARCO POLO – A new and simple tool for pollen-based stand-scale vegetation reconstruction, The Holocene, 27, 321–330, https://doi.org/10.1177/0959683616660171, 2017.
Neubauer, G.: Rekonstruktion der Nutzungsgeschichte einer Waldfläche an der Sauschwemme bei Johanngeorgenstadt (Erzgebirgskreis) anhand der Untersuchung von Holzkohle aus historischen Meilern, MSc. thesis, Institut für Waldwachstum und Forstliche Informatik, Technische Universität Dresden, 99 pp., 2022.
Neumann, M., Mues, V., Moreno, A., Hasenauer, H., and Seidl, R.: Climate variability drives recent tree mortality in Europe, Global Chang. Biol., 23, 4788–4797, https://doi.org/10.1111/gcb.13724, 2017.
Novak, M., Brizova, E., Adamova, M., Erbanova, L., and Bottrell, S. H.: Accumulation of organic carbon over the past 150 years in five freshwater peatlands in western and central Europe, Sci. Total Environ., 390, 425–436, https://doi.org/10.1016/j.scitotenv.2007.10.011, 2008.
Nožička, J.: Proměnylesů a vývoj lesního hospodaření v Krusnohorí do r. 1848 [Die Änderung des Waldbestandes und die Entwicklung der Forstwirtschaft im Erzgebirge bis 1848], Rozprav y Československé Akademie Včd. flada matematických a přírodních véd, 72 (3), Praha, 113 pp., 1962.
Overbeck, F. and Griéz, I.: Mooruntersuchungen zur Rekurrenzflächenfrage und Siedlungsgeschichte in der Rhön, Flora, 141, 51–99, 1954.
Parker, A. G., Goudie, A. S., Anderson, D. E., Robinson, M. A., and Bonsall, C.: A review of the mid-Holocene elm decline in the British Isles, Prog. Phys. Geog., 26, 1–45, https://doi.org/10.1191/0309133302pp323ra, 2002.
Peschel, A. and Wetzel, M.: Naturraum Erzgebirge, in: Erzgebirge, edited by: Schattkowsky, M., Kulturlandschaften Sachsens Band 3, Edition Leipzig, Leipzig, 9–26, 2010.
Piekalski, J. (Ed.): Historyczny krajobraz kulturowy zachodnich Sudetów, Wstęp do badań. Instytut Archeologii Uniwersytetu Wrocławskiego, Wrocław, ISBN 978-83-614116-78-4, 724 pp., 2020.
Piovesan, G., Mercuri, A. M., and Mensing, S. A.: The potential of paleoecology for functional forest restoration planning: lessons from Late Holocene Italian pollen records, Plant Biosyst., 152, 508–514, https://doi.org/10.1080/11263504.2018.1435582, 2018.
Raab, T., Raab, A., Bonhage, A., Schneider, A., Hirsch, F., Birkhofer, K., Drohan, P., Wilmking, M., Kreyling, J., Malik, I., Wistuba, M., van der Maaten, E., van der Maaten-Theunissen, M., and Urich, T.: Do small landforms have large effects? A review on the legacies of pre-industrial charcoal burning, Geomorphology, 413, 108332, https://doi.org/10.1016/j.geomorph.2022.108332, 2022.
Rösch, M.: Land use and food production in Central Europe from the Neolithic to the Medieval period: Change of landscape, soils and agricultural systems according to archaeobotanical data, in: Economic archaeology: from structure to performance in European archaeology, edited by: Kerig, T. and Zimmermann, A., Habelt, Bonn, 109–127, 2013.
Rösch, M.: Abies alba and Homo sapiens in the Schwarzwald – a difficult story, Interdisciplinaria Archaeologica, 6, 47–62, http://iansa.eu/papers/IANSA-2015-01-roesch.pdf (last access: 13 March 2021), 2015.
Rösch, M.: Long-term human impact as registered in an upland pollen profile from the southern Black Forest, south-western Germany, Veg. Hist. Archaeobot., 9, 205–218, https://doi.org/10.1007/BF01294635, 2000.
Rudolph, K.: Die bisherigen Ergebnisse der botanischen Mooruntersuchungen in Böhmen, Beihefte zum Botanischen Centralblatt, 45, Abt. II, 1–180, 1928.
Rudolph, K. and Firbas, F.: Pollenanalytische Untersuchungen böhmischer Moore (Vorläufige Mitteilung), Berichte der deutschen botanischen Gesellschaft, 40, 393–405, 1922.
Rudolph, K. and Firbas, F.: Paläofloristische und stratigraphische Untersuchungen böhmischer Moore. Die Hochmoore des Erzgebirges. Ein Beitrag zur postglazialen Waldgeschichte Böhmens, Beihefte zum Botanischen Centralblatt, 41, Abt. II, 1–162, 1924.
Rutkiewicz, P., Malik, I., Wistuba, M., and Osika, A.: High concentration of charcoal hearth remains as legacy of historical ferrous metallurgy in southern Poland, Quaternary Int., 512, 133–143, https://doi.org/10.1016/j.quaint.2019.04.015, 2019.
Ruttkowski, M.: Altstraßen im Erzgebirge. Archäologische Denkmalinventarisation Böhmischer Steige, Arbeits- und Forschungsberichte zur sächsischen Bodendenkmalpflege, 44, 264–297, 2002.
Sächsische Landesstiftung Natur und Umwelt: Praktischer Moorschutz im Naturpark Erzgebirge/Vogtland und Beispiele aus anderen Gebirgsregionen: Methoden, Probleme, Ausblick, Lausitzer Druck- und Verlagshaus, Bautzen, 93 pp., 2007.
Sächsisches Staatsministerium für Umwelt und Landwirtschaft: Naturschutzgebiete in Sachsen, Dresden, 720 pp., 2008.
Sadovnik, M., Csaplovics, E., and Heynowski, R.: Palaeoecology and geoinformatics for landscape archaeological monitoring in support of heritage documentation in the Ore Mountains, in: Proceedings International Conference Mining Cultural Landscape Krušnohory/Erzgebirge and its nomination for the UNESCO World Heritage List, National Technical Museum in Prague, 11–12 November 2013, 1–13, 2013.
Schafstall, N., Kuosmanen, N., Kuneš, P., Svobodová, H. S., Svitok, M., Chiverrell, R. C., Halsall, K., Fleischer, P., Knížek, M., and Clear, J. L.: Sub-fossil bark beetles as indicators of past disturbance events in temperate Picea abies mountain forests, Quaternary Sci. Rev., 275, 107289, https://doi.org/10.1016/j.quascirev.2021.107289, 2022.
Scheithauer, J. and Grunewald, K.: Saurer Regen und Waldsterben im ehemaligen Schwarzen Dreieck (Osterzgebirge), in: Ökologische Problemräume Deutschlands, edited by: Zepp, H., Wissenschaftliche Buchgesellschaft, Darmstadt, 111–132, 2007.
Schlöffel, M.: Die postglaziale Waldgeschichte der Lehmheide. Rekonstruktion spät- und postglazialer Umweltbedingungen an einem Torfprofil aus dem Erzgebirge, Arbeits- und Forschungsberichte zur sächsischen Bodendenkmalpflege 51/52, 9–27, 2011.
Schmeidl, H.: Beitrag zur Frage des Grenzhorizontes im Sebastiansberger Hochmoor, Beihefte zum Botanischen Centralblatt, 40, Abt. II, 493–524, 1940.
Schmidt, M., Mölder, A., Schönfelder, E., Engel, F., and Fortmann-Valtink, W.: Charcoal kiln sites, associated landscape attributes and historic forest conditions: DTM-based investigations in Hesse (Germany), For. Ecosyst., 3, 8, https://doi.org/10.1186/s40663-016-0067-6, 2016.
Schmidt, P. A.: Veränderung der Flora und Vegetation von Wäldern unter Immissionseinfluß, Forstwiss. Cent.bl., 112, 213–224, https://doi.org/10.1007/BF02742150, 1993.
Schmidt, P. A., Hempel, W., Denner, M., Döring, N., Gnüchtel, A., Walter, B., and Wendel, D.: Potentielle Natürliche Vegetation Sachsens mit Karte 1 : 200 000, Materialien zu Naturschutz und Landschaftspflege, 2002, Sächsisches Landesamt für Umwelt und Geologie, Radebeul, 230 pp., 2002.
Schreiber, H.: Die Moore und die Torfgewinnung im Erzgebirge, Arbeiten der deutschen Sektion des Landeskulturrates für Böhmen, 28, 1–45, 1921.
Schreg, R.: Uncultivated landscapes or wilderness? Early medieval land use in low mountain ranges and flood plains of Southern Germany, Eur. J. Post-Class. Archaeol., 4, 69–98, http://www.postclassical.it/PCA_vol.4_files/PCA 4_Schreg.pdf (last access: 9 June 2021), 2014.
Schubert, M.: Archäologische und naturwissenschaftliche Untersuchungen in den mittelalterlichen Bergbaustädten Dippoldiswalde und Freiberg, Acta rerum naturalium, 21, 125–140, https://actarerumnaturalium.cz/wp-content/uploads/2019/12/archiv_2017-21_10.pdf (last access: 29 May 2022), 2017.
Schubert, M. and Herbig, C.: Buntmetallurgische Prozesse aus mittelalterlichen Bergbausiedlungen im internationalen Vergleich mit einem Ausblick auf die (exquisiten) Ernährungsgewohnheiten der Dippoldiswalder und Freiberger Bergleute, Mitteilungen der Deutschen Gesellschaft für Archäologie des Mittelalters und der Neuzeit, 30, 195–208, 2017.
Schuldt, B., Buras, A., Arend, M., Vitasse, Y., Beierkuhnlein, C., Damm, A., Gharun, M., Grams, T. E. E., Hauck, M., Hajek, P., Hartmann, H., Hiltbrunner, E., Hoch, G., Holloway-Phillips, M., Körner, C., Larysch, E., Lübbe, T., Nelson, D. B., Rammig, A., Rigling, A., Rose, L., Ruehr, N., Schumann, K., Weiser, F., Werner, C., Wohlgemuth, T., Zang, C. S., and Kahmen, A.: A first assessment of the impact of the extreme 2018 summer drought on Central European forests, Basic Appl. Ecol., 45, 86–103, https://doi.org/10.1016/j.baae.2020.04.003, 2020.
Schwabenicky, W.: Der mittelalterliche Silberbergbau im Erzgebirgsvorland und im westlichen Erzgebirge unter besonderer Berücksichtigung der Ausgrabungen in der wüsten Bergstadt Bleiberg bei Frankenberg, Verlag Klaus Gumnior, Chemnitz, ISBN 3937386203, 258 pp., 2009.
Sebastian, U.: Die Geologie des Erzgebirges, Springer Spektrum, Berlin-Heidelberg, ISBN 3827429765, 279 pp., 2013.
Segers-Glocke, C. and Witthöft, H. (Eds.): Aspects of mining and smelting in the upper Harz Mountains (up to the 13th/14th century) – In the early times of a developing European culture and economy, Sachüberlieferung und Geschichte, 33, Scripta Mercaturae Verlag, St. Katharinen, ISBN 978-3-89590-101-0, 168 pp., 2000.
Seifert-Eulen, M.: Die Moore des Erzgebirges und seiner Nordabdachung, Vegetationsgeschichte ausgewählter Moore, Geoprofil, 14, 4–78, 2016.
Seim, A., Marquer, L., Bisson, U., Hofmann, J., Herzig, F., Kontic, R., Lechterbeck, J., Muigg, B., Neyses-Eiden, M., Rzepecki, A., Rösch, M., Walder, F., Weidemüller, J., and Tegel, W.: Historical spruce abundance in Central Europe: A combined dendrochronological and palynological approach, Front. Ecol. Evol., 10, 909453, https://doi.org/10.3389/fevo.2022.909453, 2022.
Sigismund, B.: Lebensbilder vom Sächsischen Erzgebirge, Lorck, Leipzig, 136 pp., 1859.
Slobodda, S.: Entstehung, Nutzungsgeschichte, Pflege- und Entwicklungsgrundsätze für erzgebirgische Hochmoore, in: Ökologie und Schutz der Hochmoore im Erzgebirge, edited by: Sächsische Akademie für Natur und Umwelt, Dresden, 10–30, 1998.
Słowiński, M., Lamentowicz, M., Łuców, D., Barabach, J., Brykała, D., Tyszkowski, S., Pieńczewska, A., Śnieszko, Z., Dietze, E., Jażdżewski, K., Obremska, M., Ott, F., Brauer, A., and Marcisz, K.: Paleoecological and historical data as an important tool in ecosystem management, J. Environ. Manage., 236, 755–768, https://doi.org/10.1016/j.jenvman.2019.02.002, 2019.
SMEKUL – Sächsisches Staatsministerium für Energie, Klimaschutz, Umwelt und Landwirtschaft (Ed.): Waldzustandsbericht 2020, Dresden, 63 pp., 2020.
Spehr, R.: Die Wüstung Warnsdorf im Tharandter Wald, Mitteilungen des Freiberger Altertumsvereines 91, 5–62, 2002.
Spitzer, J. M.: Dendrochronologische Untersuchungen von Jahrringbreiten an Pinus rotundata in der Mothhäuser Haide, Diploma thesis, Institut für Allgemeine Ökologie und Umweltschutz, Technische Universität Dresden, 78 pp., 2010.
Šrámek, V., Slodičák, M., Lomský, B., Balcar, V., Kulhavý, J., Hadaš, P., Pulkráb, K., Šišák, L., Pěnička, L., and Sloup, M.: The Ore Mountains: Will successive recovery of forests from lethal disease be successful?, Mt. Res. Dev., 28, 216–221, https://doi.org/10.1659/mrd.1040, 2008.
Staatsbetrieb Sachsenforst (Ed.): Exkursionsführer zur AFSV-Jahrestagung 2017. Standortswandel und Waldumbau im Oberen Erzgebirge, Pirna, 119 pp., 2017.
Stebich, M. and Litt, T.: Das Georgenfelder Hochmoor – ein Archiv für Vegetations-, Siedlungs- und Bergbaugeschichte, Leipziger Geowissenschaften, 5, 209–216, 1997.
Stojakowits, P. and Friedmann, A.: Anthropogene Einflussnahme in den Hochlagen des Bayerischen Waldes. Aussagen aufgrund der bisherigen Pollenanalysen, Fines Transire, 28, 175–185, 2019.
Suda, T.: Historie vegetace Chebské pánve ze sedimentárního záznamu lokality SOOS, Diploma thesis, Univerzita Karlova v Praze, Prague, 65 pp., 2012.
Sugita, S.: Theory of quantitative reconstruction of vegetation I: pollen from large sites REVEALS regional vegetation composition, The Holocene, 17, 229–241, https://doi.org/10.1177/0959683607075837, 2007a.
Sugita, S.: Theory of quantitative reconstruction of vegetation II: all you need is LOVE, The Holocene, 17, 243–257, https://doi.org/10.1177/0959683607075838, 2007b.
Theuerkauf, M. and Couwenberg, J.: The extended downscaling approach: A new R-tool for pollen-based reconstruction of vegetation patterns, The Holocene, 27, 1252–1258, https://doi.org/10.1177/095968361668325, 2017.
Theuerkauf, M. and Couwenberg, J.: ROPES reveals past land cover and PPEs from single pollen records, Front. Earth Sci., 6, 14, https://doi.org/10.3389/feart.2018.00014, 2018.
Theuerkauf, M., Haberl, A., Wulf, K., Joosten, H., and DUENE e.V. Greifswald: Holozäner Vegetationswandel im Einzugsgebiet der Mothhäuser Haide/Erzgebirge, Unpublished project report as part of the LfUG project “Auswirkungen des Klimawandels auf wasserabhängige Ökosysteme”, University of Greifswald, 55 pp., 2007.
Thomasius, H.: The influence of mining in the Saxon Erzgebirge on woods and forestry up to the beginning of the 19th century, GeoJournal, 32, 103–125, https://doi.org/10.1007/BF00812496, 1994.
Tolksdorf, J. F. (Ed.): Mittelalterlicher Bergbau und Umwelt im Erzgebirge. Eine interdisziplinäre Untersuchung, Veröffentlichungen des Landesamtes für Archäologie Sachsen, 67, Landesamt für Archäologie Sachsen, Dresden, ISBN 3943770370, 213 pp., 2018.
Tolksdorf, J. F. and Scharnweber, T.: Holzfunde als mehrdimensionale Quelle zur Rekonstruktion von Wirtschaft und Landschaft. in: Mittelalterlicher Bergbau und Umwelt im Erzgebirge. Eine interdisziplinäre Untersuchung, edited by: Tolksdorf, J. F., Veröffentlichungen des Landesamtes für Archäologie Sachsen, 67, Landesamt für Archäologie Sachsen, Dresden, 37–56, ISBN 3943770370, 2018.
Tolksdorf, J. F. and Schröder, F.: Rekonstruktion von Holznutzung und Landschaftsgeschichte in einer mittelalterlichen Bergbaulandschaft bei Niederpöbel. Interdisziplinärer Ansatz und methodische Abwägungen, Mitteilungen der Deutschen Gesellschaft für Archäologie des Mittelalters und der Neuzeit, 29, 175–184, 2016.
Tolksdorf, J. F., Elburg, R., Schröder, F., Knapp, H., Herbig, C., Westphal, T., Schneider, B., Fülling, A., and Hemker, C.: Forest exploitation for charcoal production and timber since the 12th century in an intact medieval mining site in the Niederpöbel Valley (Erzgebirge, Eastern Germany), J. Archaeol. Sci. Rep., 4, 487–500, https://doi.org/10.1016/j.jasrep.2015.10.018, 2015.
Tolksdorf, J. F., Kaiser, K., and Bertuch, M.: Stand der Untersuchungen zur Landschaftsgeschichte. in: Mittelalterlicher Bergbau und Umwelt im Erzgebirge. Eine interdisziplinäre Untersuchung, edited by: Tolksdorf, J. F., Veröffentlichungen des Landesamtes für Archäologie Sachsen, 67, Landesamt für Archäologie Sachsen, Dresden, 30–33, ISBN 3943770370, 2018.
Tolksdorf, J. F., Hemker, C., and Schubert, M.: Bronzezeitlicher Zinnseifenbergbau bei Schellerhau im östlichen Erzgebirge, Sachsen, Der Anschnitt, 71, 223–233, 2019.
Tolksdorf, J. F., Kaiser, K., Petr, L., Herbig, C., Kočár, P., Heinrich, S., Wilke, F. D. H., Theuerkauf, M., Fülling, A., Schubert, M., Schröder, F., Křivánek, R., Schulz, L., Bonhage, A., and Hemker, C.: Past human impact in a mountain forest: geoarchaeology of a medieval glass production and charcoal hearth site in the Erzgebirge, Germany, Reg. Environ. Chang., 20, 71, https://doi.org/10.1007/s10113-020-01638-1, 2020a.
Tolksdorf, J. F., Schröder, F., Petr, L., Herbig, C., Kaiser, K., Kočár, P., Fülling, A., Heinrich, S., Hönig, H., and Hemker, C.: Evidence for Bronze Age and Medieval tin placer mining in the Erzgebirge mountains, Saxony (Germany), Geoarchaeology, 35, 198–216, https://doi.org/10.1002/gea.21763, 2020b.
Tolksdorf, J. F., Schubert, M., Schröder, F., Bertuch, M., and Heinrichsen, A.: Meilerplätze im Erzgebirge – Erfassung mittels LiDAR und Einbeziehung dieser Quellengattung in der Montanarchäologie, in: Beiträge zur Landnutzungsgeschichte in der Niederlausitz und im Erzgebirge, edited by: Hirsch, F., Raab, A. and Raab, Geopedology and Landscape Development Research Series (GeoRS), 10, 82–91, Brandenburgische Technische Universität, Cottbus, https://doi.org/10.26127/BTUOpen-5388, 2021.
Valde-Nowak, P.: Short settled Neolithic sites in the Mountains – Economy or religious practice? Case studies from the Polish Carpathians and German Mid-Mountains, in: Economic Archaeology: From Structure to Performance in European Archaeology, edited by: Kerig, T. and Zimmermann, A., Habelt, Bonn, 215–225, 2013.
van der Knaap, W. O., van Leeuwen, J. F. N., Fahse, L., Szidat, S., Studer, T., Baumann, J., Heurich, M., and Tinner, W.: Vegetation and disturbance history of the Bavarian Forest National Park, Germany, Veget. Hist. Archaeobot., 29, 277–295, https://doi.org/10.1007/s00334-019-00742-5, 2020.
Vejpustková, M., Čihák, T., Samusevich, A., Zeidler, A., Novotný, R., and Šrámek, V.: Interactive effect of extreme climatic event and pollution load on growth and wood anatomy of spruce, Trees, 31, 575–586, https://doi.org/10.1007/s00468-016-1491-5, 2017.
Veron, A., Novak, M., Brizova, E., and Stepanova, M.: Environmental imprints of climate changes and anthropogenic activities in the Ore Mountains of Bohemia (Central Europe) since 13 cal. kyr BP, The Holocene, 24, 919–931, https://doi.org/10.1177/0959683614534746, 2014.
Vilímek, V. and Raška, P.: The Krušné Hory Mts. – The Longest Mountain Range of the Czech Republic, in: Landscapes and Landforms of the Czech Republic, edited by: Pánek, T. and Hradecký, J., World Geomorphological Landscapes, Springer, Berlin, 113–122, https://doi.org/10.1007/978-3-319-27537-6_10, 2016.
Vilímek, V., Fantucci, R., and Stemberk, J.: Dendrogeomorphological investigations of slope deformation on Salesius Hill in the Krušné Hory Mts, In: Landslides. Proceedings of the First European Conference on Landslides, Prague, Czech Republic, 24–26 June 2002, edited by: Rybář, J., Stemberk, J., and Wagner, P., Routledge, London, 321–326, 2018.
Vlach, V., Matouskova, M., and Ledvinka, O.: Impacts of regional climate change on hydrological drought characteristics in headwaters of the Ore Mountains, River Res. Appl., 37, 919–930, https://doi.org/10.1002/rra.3818, 2021.
von Carlowitz, H. C. and von Rohr, J. B.: Sylvicultura Oeconomica (reprint of the 2nd edition from 1732), Kessel, Remagen-Oberwinter, ISBN 978-3-941300-19-4, 69 pp., 2012.
Wagenbreth, O. and Wächtler, E. (Eds.): Der Freiberger Bergbau. Technische Denkmale und Geschichte, Springer Spektrum, Berlin & Heidelberg, ISBN 978-3-662-44763-5, 385 pp., 1988.
Wahlmüller, N.: Palynologische Forschung in den Ostalpen und ihren vorgelagerten Gebieten, Berichte des Naturwissenschaftlich-medizinischen Vereins in Innsbruck, 80, 81–95, 1993.
Weber, C. A.: Grenzhorizont und Klimaschwankungen, Abhandlungen des Naturwissenschaftlichen Vereins zu Bremen, 26, 98–106, 1926.
Wendel, D.: Autogene Regenerationserscheinungen in erzgebirgischen Moorwäldern und deren Bedeutung für Schutz und Entwicklung der Moore, Ph.D. thesis, Technische Universität Dresden, 233 pp., 2010.
Wenzel, W.: Slawische Namen im Erzgebirge mit besonderer Berücksichtigung des Raumes um Limbach-Oberfrohna, Sächsische Heimatblätter, 62, 214–217, https://journals.qucosa.de/shb/article/view/98 (last access: 8 October 2021), 2016.
Wetzel, M.: Das Erzgebirge im Wandel der Geschichte. in: Erzgebirge, edited by: Schattkowsky, M., Kulturlandschaften Sachsens 3, Edition Leipzig, Leipzig, 27–72, 2010.
Wiezik, M., Jamrichová, E., Máliš, F., Beláňová, E., Hrivnák, R., Hájek, M., and Hájková, P.: Transformation of West-Carpathian primeval woodlands into high-altitude grasslands from as early as the Bronze Age, Veget. Hist. Archaeobot., 1–6, https://doi.org/10.1007/s00334-022-00896-9, 2022.
Willutzki, H.: Zur Waldgeschichte und Vermoorung sowie über Rekurrenzflächen im Oberharz, Nova Acta Leopoldina NF, 25, 1–52, 1962.
Wilsdorf, H., Herrmann, W., and Löffler, K.: Bergbau, Wald, Flöße: Untersuchungen zur Geschichte der Flößerei im Dienste des Montanwesens und zum montanen Transportproblem, Freiberger Forschungshefte, 28, Akademie-Verlag, Berlin, 360 pp., 1960.
Zelinka, V., Zacharová, J., and Skaloš, J.: Analysis of spatiotemporal changes of agricultural land after the Second World War in Czechia, Sci. Rep., 11, 1–15, https://doi.org/10.1038/s41598-021-91946-1, 2021.
Zimmermann, F., Lux, H., Maenhaut, W., Matschullat, J., Plessow, K., Reuter, F., and Wienhaus, O.: A review of air pollution and atmospheric deposition dynamics in southern Saxony, Germany, Central Europe, Atmos. Environ., 37, 671–691, https://doi.org/10.1016/S1352-2310(02)00829-4, 2003.
Zinke, P.: Nutzungsgeschichte, Zustand und Revitalisierung der Moore im Erzgebirge, Telma, 32, 267–280, https://doi.org/10.23689/fidgeo-2884, 2002.
- How to cite
- Abstract
- Kurzfassung
- Introduction: an extreme mountain forest landscape
- Study area
- History of regional palynological research
- Available data
- Outline of the Holocene vegetation history using pollen data
- Discussion
- Conclusions
- Data availability
- Author contributions
- Competing interests
- Disclaimer
- Acknowledgements
- Financial support
- Review statement
- References
- Supplement
- How to cite
- Abstract
- Kurzfassung
- Introduction: an extreme mountain forest landscape
- Study area
- History of regional palynological research
- Available data
- Outline of the Holocene vegetation history using pollen data
- Discussion
- Conclusions
- Data availability
- Author contributions
- Competing interests
- Disclaimer
- Acknowledgements
- Financial support
- Review statement
- References
- Supplement