the Creative Commons Attribution 4.0 License.
the Creative Commons Attribution 4.0 License.
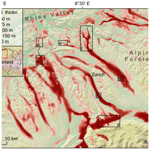
Comparison of overdeepened structures in formerly glaciated areas of the northern Alpine foreland and northern central Europe
Lukas Gegg
Frank Preusser
Gegg, L. and Preusser, F.: Comparison of overdeepened structures in formerly glaciated areas of the northern Alpine foreland and northern central Europe, E&G Quaternary Sci. J., 72, 23–36, https://doi.org/10.5194/egqsj-72-23-2023, 2023.
Overdeepened structures occur in formerly and presently glaciated regions around the earth and are usually referred to as overdeepenings or tunnel valleys. The existence of such troughs has been known for more than a century, and they have been attributed to similar formation processes where subglacial meltwater plays a decisive role. This comparison highlights that (foreland) overdeepenings and tunnel valleys further occur in similar dimensions and share many characteristics such as gently sinuous shapes in plan view, undulating long profiles with terminal adverse slopes, and varying cross-sectional morphologies. The best explored examples of overdeepened structures are situated in and around the European Alps and in the central European lowlands. Especially in the vicinity of the Alps, some individual troughs are well explored, allowing for a reconstruction of their infill history, whereas only a few detailed studies, notably such involving long drill core records, have been presented from northern central Europe. We suggest that more such studies could significantly further our understanding of subglacial erosion processes and the regional glaciation histories and aim to promote more intense exchange and discussion between the respective scientific communities.
In den ehemals und gegenwärtig vergletscherten Regionen der Erde finden sich übertiefte Beckenstrukturen, die üblicherweise als Übertiefungen oder Tunneltäler angesprochen werden. Die Existenz dieser Tröge ist seit mehr als einem Jahrhundert bekannt, und sie wurden ähnlichen Erosionsprozessen zugeschrieben, bei denen subglaziales Schmelzwasser eine entscheidende Rolle spielt. Mit diesem Vergleich möchten wir zeigen, dass (Vorland-)Übertiefungen und Tunneltäler in vergleichbaren Dimensionen auftreten, und eine Reihe weiterer gemeinsamer Merkmale aufweisen, zum Beispiel sanft gewundene Längsachsen, undulierende Profile mit Gegensteigungen am distalen Ende, und variable Formen im Querschnitt. Die bestuntersuchten Beispiele für übertiefte Becken befinden sich im europäischen Alpenraum, und in zentraleuropäischen Tiefländern. Vor allem im Alpenraum wurden einige übertiefte Strukturen detailliert untersucht, was eine Rekonstruktion ihrer Verfüllung erlaubt. Im Gegensatz dazu existieren nur wenige (bohrungsbasierte) Detailstudien im nördlichen Mitteleuropa. Wir betonen, dass solche Untersuchungen zu unserem Verständnis subglazialer Erosionsprozesse, aber auch regionaler Vergletscherungsgeschichten beitragen können, und möchten Austausch und Diskussion unter den entsprechenden wissenschaftlichen Lagern anregen.
The repeated glaciations during the Quaternary period shaped large swathes of the earth's surface, first, by eroding and removing large rock masses from mountain areas and other regions covered by ice; glacially polished bedrock surfaces and deep valley flanks are some of the most striking features in this context. Second, the accumulation of rock debris transported by ice and meltwater led to the formation of characteristic landscape features such as moraine ridges and gravel terraces. However, a third feature that represents a combination of both erosive and aggradational processes is less well known to a broader public: geological structures deeply incised by subglacial erosion into pre-existing landscapes but hidden below the present land surface after being filled up by sediment and/or occupied by large bodies of water. First recognised within and around the European Alps as well as below the central European lowlands, these troughs are referred to as overdeepenings and tunnel valleys, respectively.
While these structures have been known since the late 19th century, for a long time they attracted relatively little scientific attention as their investigation is costly due to the fact that it requires the use of either deep drilling or geophysics – ideally a combination of both. Since the beginning of the 21st century, scientific interest in glacially eroded, and specifically overdeepened, structures increased considerably, to some extent motivated by applied aspects. On the one hand, these structures have a poorly explored potential as groundwater sources and for geothermal energy production. On the other hand, planning nuclear waste repositories requires the identification of areas that will ideally not be affected by deep glacial erosion in the near geological future (i.e. the next million years), as this may challenge the intactness of the disposal site.
Advances in geophysical techniques together with the increased interest in glacially eroded structures have triggered several projects into the subject during the past 2 decades notably in the aforementioned areas in central Europe. These can thus be regarded as the best explored regions with regard to overdeepened structures and, due to their proximity, should be subject to similar evolutionary trends over time. However, there has been very limited exchange among the communities working in and around the Alps and the northern central European lowlands, respectively. This is in contrast to the common assumption that all these structures were formed by similar erosion processes, and, hence, it should be expected they share many similarities.
The aim of this article is to summarise and compare the current knowledge about overdeepenings and tunnel valleys in general but with special regard to the northern Alpine foreland and the wider North Sea area. This comparison is done specifically in light of the question whether there are relevant differences in the morphology and/or filling history of these troughs or whether they are just different examples of basically the same type of feature. We thus touch only lightly upon the concepts of the erosion mechanisms that have been intensively discussed by others (e.g. Alley et al., 2019; Cook and Swift, 2012, and references therein).
The term “overdeepening” is attributed to Albrecht Penck, one of the pioneers and most prominent representatives of Alpine Quaternary geology. He used it to describe intensive and deep-reaching glacial erosion focused in the main Alpine trunk valleys whose valley floors lie significantly below their off-cut tributaries (Penck and Brückner, 1909). The concept of deep-reaching subglacial erosion had been subject to intensive discussion (e.g. Heim, 1885) but was lastly confirmed in 1908 by a tragic accident during tunnel works in central Switzerland (Lötschberg), when blasting their way into an infilled overdeepening ∼170 m below ground cost the lives of 24 workers, as the liquefied valley infill flooded the tunnel (Waltham, 2008). Over the following decades, boreholes and geophysical data revealed the existence of such deep infilled bedrock troughs below the majority of the present-day valleys not only in the Alps and the Alpine foreland (cf. Haeberli et al., 2016; Preusser et al., 2010) but also in other mountain regions on earth (e.g. Carrivick et al., 2016; Gao, 2011; James et al., 2019; Magnússon et al., 2012; Smith, 2004). First systematic compilations of these deeply incised features were provided by Van Husen (1979) and Wildi (1984), who noted that large sections of these troughs lie deeper than the bedrock at the respective valley outlet. This closed basin shape with a terminal adverse slope is today generally regarded as defining an overdeepening (Alley et al., 2019; Cook and Swift, 2012; Patton et al., 2016). Notably – but not exclusively – in Switzerland, overdeepenings have since been intensively studied based on scientific drillings (Hsü and Kelts, 1984; Preusser et al., 2005; Schlüchter, 1989; Schwenk et al., 2022), geophysical campaigns (Bandou et al., 2022; Burschil et al., 2018, 2019; Finckh et al., 1984; Nitsche et al., 2001; Reitner et al., 2010), or a combination of both (Buechi et al., 2018; Dehnert et al., 2012; Gegg et al., 2021; Pomper et al., 2017).
The recognition of tunnel valleys goes similarly far back in time, to the late 19th century, when Danish and north German scholars identified deep subsurface troughs infilled with Quaternary sediments or hosting lakes (cf. van der Vegt et al., 2012). Jentzsch (1884) already hypothesised about an origin by subglacial fluvial erosion, a concept that was refined by Ussing (1904), who attributed these troughs, characterised by internal and terminal adverse slopes, to the action of pressurised subglacial meltwater. The model of Ussing (1904) became increasingly widely accepted, and the “tunnel valleys” (Madsen, 1921) became a subject of increasing scientific interest. Systematic regional-scale mapping and investigation started in the 1960s (e.g. Kuster and Meyer, 1979) and was expanded to other regions such as Great Britain (e.g. Woodland, 1970) and North America (e.g. Wright, 1973). Soon, the widespread availability of high-resolution marine seismic data acquired for oil and gas exploration marked a breakthrough in the recognition of tunnel valleys: they were encountered in previously glaciated shelf areas all around the world and could be temporally efficiently mapped on reflection-seismic sections (Boyd et al., 1988; Destombes et al., 1975; Kunst and Deze, 1985). Tunnel valley characterisation made a second major leap forward with the establishment of 3D seismic acquisition (Praeg, 1997). Based on these datasets, the longitudinal and transverse morphology of individual structures can be imaged and analysed in high detail and free from gaps (Kirkham et al., 2021; Kristensen et al., 2008; Ottesen et al., 2020; Stewart et al., 2013). Further insights derive from electromagnetic (e.g. Bosch et al., 2009; Tezkan et al., 2009) as well as gravimetric studies (e.g. Barker and Harker, 1984; Götze et al., 2009), while only little detailed borehole information is available (e.g. Piotrowski, 1994).
In extensively glaciated mountain ranges, such as the European Alps, large overdeepenings occur in two endmember settings: in the major intermontane trunk valleys and in the mountain forelands (Dürst Stucki and Schlunegger, 2013; Magrani et al., 2020; Preusser et al., 2010). Within the mountain range, the bedrock is generally quite resilient towards erosion (Kühni and Pfiffner, 2001), and glacial erosion occurs preferentially along zones of weakness such as faults (Dürst Stucki and Schlunegger, 2013). This is a self-promoting process: with ongoing downcutting, drainage of water and ice along the deepening valley becomes increasingly efficient, and erosion is further focused on the valley floor, the result being a spatially stable, deeply incised valley, where ice flows comparatively rapidly and under strong lateral confinement (Egholm et al., 2012; Herman et al., 2011; Ugelvig et al., 2016). Glacial erosion sensu stricto, i.e. quarrying and abrasion, probably plays an important role in this setting, aided by subglacial meltwater stripping debris off the glacier base (Alley et al., 2019). It culminates in the formation of overdeepenings, for example at confluences or valley constrictions where ice flow is accelerated (Cook and Swift, 2012; Dürst Stucki and Schlunegger, 2013; Herman et al., 2015; Preusser et al., 2010).
Foreland overdeepenings, in contrast, develop beneath the piedmont tongues of valley glaciers. There, although its large-scale pattern is defined by the locations of mountain valley outlets, the ice flow is topographically much less constrained and potentially diffluent and the ice thickness is significantly smaller (Bini et al., 2009), and as a result, glacial erosion sensu stricto should be rather limited. However, with increasing catchment area, basal water availability also increases towards the glacier termini. This subglacial water, pressurised by the englacial water column, is regarded as a driver of overdeepening erosion in the foreland setting (Alley et al., 1997; Dürst Stucki et al., 2010; Dürst Stucki and Schlunegger, 2013; Gegg et al., 2021), where the bedrock commonly consists of poorly consolidated Molasse-type sediments (Kühni and Pfiffner, 2001). As these deposits are generally readily eroded, the occurrence of faults does not appear to play a significant role facilitating their erosion (Dürst Stucki and Schlunegger, 2013; Gegg et al., 2021). With the effect of structural preconditioning being low and ice flow being largely unconfined, the focusing of subglacial erosion may shift over time, evidenced for example by branching and off-cutting overdeepened troughs (Buechi et al., 2018; Ellwanger et al., 2011).
The primary erosive agent of tunnel valleys is, by definition, inferred to be basal meltwater (Cofaigh, 1996; Kehew et al., 2012; van der Vegt et al., 2012) at the margins of continental-scale ice sheets, where topographical confinement of ice flow is significantly lower than within mountain ranges (Schoof and Hewitt, 2013). Still, discharge at ice sheet margins is concentrated along ice streams, corridors of enhanced ice flow (Margold et al., 2015; Rignot et al., 2011) that develop where basal meltwater abundance is high and facilitates tunnel valley incision (Jennings, 2006; Lelandais et al., 2018). Further, where tunnel valleys occur near highland areas, some lie distinctly in extension of fjord valleys that direct the larger-scale flow pattern of ice and water (e.g. Bradwell et al., 2008; Kearsey et al., 2019). Large tunnel valleys occur predominantly within rather soft sedimentary substrata, while troughs in more resistant lithologies tend to be smaller and narrower (Janszen et al., 2012; Jørgensen and Sandersen, 2006). In resilient crystalline bedrock, tunnel valleys are typically lacking but are replaced by large-scale esker systems that are interpreted to reflect meltwater streams cut into the basal ice instead of the substratum (Boulton et al., 2009; Clark and Walder, 1994).
4.1 Large-scale morphologies
Foreland overdeepenings are up to 15 km wide and 850 m deep bedrock incisions that reach lengths of >100 km (James et al., 2019; Magrani et al., 2020; Smith, 2004). They have been described as slightly sinuous troughs that may be arranged in seemingly anastomosing patterns (Fig. 1; Dürst Stucki and Schlunegger, 2013; Preusser et al., 2010). While some troughs have been re-excavated by multiple phases of ice advance (e.g. Birrfeld overdeepening; Nitsche et al., 2001; Lower Glatt Valley overdeepening; Buechi et al., 2018), the focus of overdeepening has in other areas laterally shifted over the course of several glaciations and produced subparallel (e.g. Reuss Valley; Jordan, 2010) or radially diverting branch basins (e.g. Lake Constance area; Ellwanger et al., 2011). The spacing between individual troughs is 5–20 km (Cummings et al., 2012; see also Jordan, 2010), and their sinuosities are around 1.05–1.10 (Gegg et al., 2021). In the longitudinal section, foreland overdeepenings often consist of several distinct sub-basins separated by abrupt bedrock swells and terminating with mostly gentle (∼ 1–2∘) adverse slopes (Jordan, 2010; Magrani et al., 2020; Preusser et al., 2010). While typically shifted upstream in intra-mountain overdeepenings, the deepest points in most foreland overdeepenings lie roughly at their central position (Magrani et al., 2020).
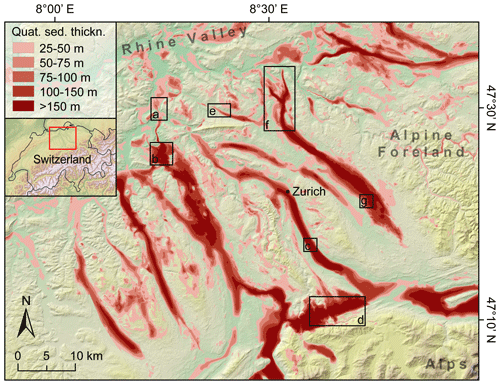
Figure 1Foreland overdeepenings in central northern Switzerland highlighted as areas of increased Quaternary sediment thickness. Data from Pietsch and Jordan (2014), Gegg et al. (2021), and references therein. Black boxes highlight study areas mentioned in the text: (a) Lower Aare Valley (Gegg et al., 2021), (b) Birrfeld (Graf, 2009; Nitsche et al., 2001), (c) Lake Zurich (Lister, 1984a, b), (d) Richterswil (Wyssling, 2002), (e) Wehntal (Anselmetti et al., 2010; Dehnert et al., 2012), (f) Lower Glatt Valley (Buechi et al., 2018), and (g) Uster (Wyssling and Wyssling, 1978).
Tunnel valleys have maximum widths of ∼12 km and maximum depths of ∼500 m and can be over 150 km long (Cameron et al., 1987; Lutz et al., 2009; Praeg, 1997; Stewart et al., 2013). In plan view, they have straight to slightly sinuous courses and can occur in swarms or in pseudo-anastomosing (i.e. different branches of seemingly individual valleys belong to different generations of valley formation; Fig. 2; Jørgensen and Sandersen, 2006; Kristensen et al., 2008), radiating or tributary networks (Cofaigh, 1996; Kehew et al., 2012; van der Vegt et al., 2012). The lateral spacing between individual structures typically ranges between 5 (Livingstone and Clark, 2016) and 20–30 km (Kehew et al., 2012; van der Vegt et al., 2012), while Lutz et al. (2009) specifically note that tunnel valleys are not uniformly distributed and may in some areas be lacking entirely. According to data by Streuff et al. (2022), typical tunnel valley sinuosities are around 1.05. Tunnel valleys characteristically start and terminate abruptly (with terminal adverse slope angles in some cases exceeding 10∘; Kristensen et al., 2008) and have undulating long courses comprising sub-basins as well as steep thresholds (Cofaigh, 1996; Kristensen et al., 2008; Lutz et al., 2009). The deepest points along the thalweg frequently lie far downstream from the valley centre (Jørgensen and Sandersen, 2006).
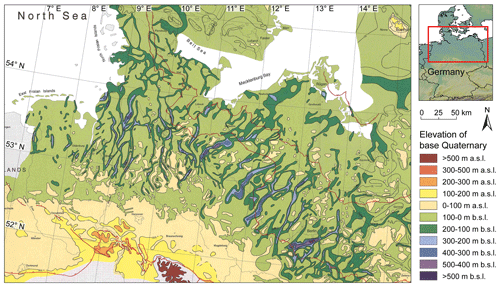
Figure 2Buried tunnel valleys in northern Germany. Red lines mark, from NE to SW, the ice extents of the Weichselian, Saalian, and Elsterian main glacial stages. Base map (Stackebrandt et al., 2001) courtesy of the Geological Survey of Brandenburg (Landesamt für Bergbau, Geologie und Rohstoffe; LBGR), Germany (https://lbgr.brandenburg.de/lbgr/de/, last access: 5 November 2022), © LBGR 2001.
Based on datasets by Magrani et al. (2020) and Kristensen et al. (2008), Swiss foreland overdeepenings tend on average to be shorter, shallower, and narrower than Danish tunnel valleys, although only the difference in depth is statistically significant (unequal-variance t tests; Fig. 3). It should be noted, however, that the dataset by Kristensen et al. (2008) refers only to a restricted area and that other studies in the North Sea found tunnel valleys that generally tend to be shallower (Andersen et al., 2012) and narrower (Jørgensen and Sandersen, 2006; Stewart et al., 2013). The overall size of tunnel valleys as well as their form ratio appears to be influenced mainly by the erosional resistance of the substratum, whereas the valley width has been linked rather to glaciological parameters (e.g. ice thickness; van der Vegt et al., 2012). The maximum size of foreland overdeepenings on the other hand is clearly limited by the spatial extent of the respective piedmont glaciers, which were considerably smaller for example in front of the Eastern or Southern Alps (or in other mountain ranges) than in northern Switzerland (e.g. Preusser et al., 2010). Still, the comparison shows that, although the corresponding ice masses differ vastly in size, the dimensions of tunnel valleys and foreland overdeepenings are not necessarily greatly different.
4.2 Detailed cross-sectional morphologies
Cross-sections of foreland overdeepenings are frequently asymmetric and tend towards a U shape, but especially in more resistant bedrock lithologies, steep-angled (up to 60∘) V-shaped valleys have been observed (Fig. 4; Dürst Stucki and Schlunegger, 2013; Jordan, 2010; Preusser et al., 2010), and both morphologies may occur within a single overdeepening (Gegg et al., 2021). Recent highly resolved geophysical studies have further revealed irregular and stepped flanks. The same morphologies are also encountered in tunnel valleys (Fig. 4). In a dataset comprising >900 Danish tunnel valleys, Andersen et al. (2012) identified ∼65 % of mainly U-shaped or flat-bottomed and ∼35 % of V-shaped structures. Flank slopes vary between <5∘ and >55∘ (Cofaigh, 1996; Huuse and Lykke-Andersen, 2000), and van der Vegt et al. (2012) mention examples of overhanging valley flanks. Individual valleys exhibit downstream transitions in a cross-section, for example, from a V to a U shape (Giglio et al., 2022). In both cases, overdeepenings and tunnel valleys, U-shaped morphologies with flat valley bottoms may be linked to lithological boundaries in the substratum (Gegg et al., 2021; Janszen et al., 2012) but occur also where the bedrock is seemingly rather homogeneous (e.g. Fig. 4a, d)
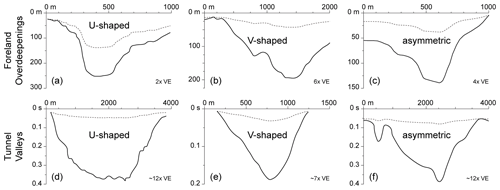
Figure 4Comparison of overdeepening (a–c) and tunnel valley (d–f) cross-sections. x axis: horizontal distance, y axis: depth (a–c)/two-way travel time (d–f). VE: vertical exaggeration (dotted lines plot the respective cross-sections without vertical exaggeration). (a) Basadingen trough, northern Switzerland (after Anselmetti et al., 2022; Brandt, 2020); (b) Tannwald basin, southern Germany (after Burschil et al., 2018); (c) Gebenstorf-Stilli Trough, northern Switzerland (after Gegg et al., 2021); (d) offshore tunnel valley, southern North Sea (after Benvenuti and Moscariello, 2016); (e) offshore tunnel valley, central North Sea (after Kirkham et al., 2021); (f) offshore tunnel valley, southeastern North Sea (after Lohrberg et al., 2020). Note frequently occurring stepped valley flanks.
Concrete information regarding the sedimentary fill of overdeepened structures is rather limited and is often derived from commercial or geotechnical drillings. Under such circumstances, typically no explicit sedimentological descriptions or even further reaching analyses (e.g. pollen analyses, dating) have been carried out. As a result, for the time being, detailed knowledge about the infilling history of overdeepenings is limited to a few case studies. As stated previously, a majority of detailed studies stem from central Europe. This applies especially to the Alpine realm, while fewer well-documented drillings exist for the North Sea region. There, the reconstruction of the sedimentary filling is hence often based on the interpretation of 2D or 3D seismic data that are frequently available from hydrocarbon exploration. We would like to highlight that although we focus on examples from central Europe, a variety of similar case studies on overdeepened structures in other areas exist, e.g. Iceland (Andrews et al., 2000; Gregory, 2012; Quillmann et al., 2010), North America (Atkinson et al., 2013; Smith, 2004; Russell et al., 2003), Patagonia (Bertrand et al., 2017; Boyd et al., 2008; Moernaut et al., 2009), and even regions glaciated during the Palaeozoic (Clerc et al., 2013; Vesely et al., 2021).
5.1 Examples from the northern Alpine foreland
A number of well-studied profiles exist in the greater Bern area/Aare Valley, Switzerland (cf. Schlüchter, 1979). The sequence of Thalgut shows a complex deposition and erosion history and is considered one of the most complete Quaternary sequences of the northern Alpine foreland (Schlüchter, 1989). The bottom part of the sequence is composed of lake deposits with pollen spectra typical of the Holsteinian Interglacial (Welten, 1982, 1988), usually attributed to Marine Isotope Stage (MIS) 11 (424–374 ka; cf. Cohen and Gibbard, 2019). Hence, the formation (or re-excavation) of the overdeepening likely occurred during MIS 12 (478–424 ka). Close to the city of Bern, the Rehhag scientific drilling targeted a tributary overdeepening feeding into the central Aare Valley and recovered a diverse, 240 m thick Quaternary succession (Schwenk et al., 2022). It is subdivided into two basin fill sequences of glacial and (glacio-)lacustrine deposits, the upper being attributed to MIS 8–7 (300–191 ka). Just north of Bern, the core of Meikirch starts with till, followed by a 70 m succession of lacustrine sediments comprising a complex vegetation history with three pronounced warm phases (Welten, 1982; Preusser et al., 2005). Based on luminescence dating, this part of the sequence was assigned to MIS 7 (243–191 ka; Preusser et al., 2005). Above the lake deposits, two successions from glaciofluvial to glacial deposits are recorded. In the overdeepened lowermost Aare Valley, at the confluence with Reuss and Limmat, the sediment filling is dominated by lacustrine sand overlying a thin layer of coarse-grained debris at the trough base (Gegg et al., 2021, and references therein). Towards the distal part of the trough, the glaciolacustrine sand interfingers with deltaic gravel. This rather unusual infill pattern is most likely related to the local confluence situation as well as the overdeepening's narrow cross-section combined with large discharge of meltwater (see also Gegg et al., 2020). The Birrfeld basin in the lower Reuss Valley contains a multiphase infill that has been attributed to up to five different ice advances (Graf, 2009; Nitsche et al., 2001).
At a water depth of 180 m, a drilling in Lake Zurich recovered a thick succession of Late Pleistocene sediments starting with coarse-grained debris interpreted as till and subglacial outwash (Lister, 1984a, b). This debris is overlain by >100 m of diamictic glaciolacustrine muds that repeatedly show traces of glaciotectonic deformation. They transition into laminated, presumably varved, basin fines and are followed by ∼30 m of postglacial lake deposits. The complex filling of the Richterswil trough, west of Lake Zurich, indicates deposition possibly related to three individual glaciations, but this evidence lacks detailed sedimentological and further analyses (Wyssling, 2002; Preusser et al., 2010; Fig. 5).
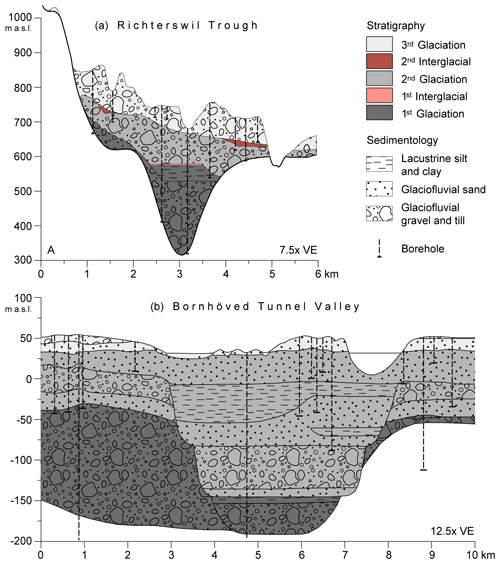
Figure 5Examples of borehole-constrained sedimentary infills of a central Swiss foreland overdeepening (a: Richterswil trough; after Wyssling, 2002, in Preusser et al., 2011) and a northern German tunnel valley (b: Bornhöved tunnel valley; after Piotrowski, 1994). VE: vertical exaggeration.
Several cores taken from the Wehntal trough (Niederweningen) show that the infill starts with glacial deposits followed by lake sediments, first containing dropstones (Anselmetti et al., 2010; Dehnert et al., 2012). Increased shear strength in the lacustrine deposits implies a second glacial advance, grounding in the lake, followed by a final lake and subsequent bog stage. According to luminescence dating, the carving of the basin occurred during early MIS 6 (around 185 ka), whereas the second ice advance is assigned to ca. 140 ka.
In the Lower Glatt Valley, Buechi et al. (2018) distinguished up to nine separate depositional sequences within the infills of the Kloten Trough and its branch basins, three of which represent episodes of overdeepened basin fill. These are characterised by successions of tills and gravel fining upwards into glaciodeltaic to glaciolacustrine deposits, the oldest dating back to at least MIS 8 (300–243 ka). In the middle part of the Glatt Valley, the >200 m deep trough of Uster is filled by till and partially covered by ice decay and meltwater deposits, followed by laminated lake sediments with a thickness of 100–150 m that are interpreted to represent varved late-glacial deposits (Wyssling and Wyssling, 1978; Preusser et al., 2011). The infill of the trough is covered by the deposits of one or possibly two later glacial advances.
In the Lake Constance area, Ellwanger et al. (2011) distinguish three major generations of overdeepenings, with the drilling in the Tannwald basin being the best documented for the time being (LGRB, 2015; Burschil et al., 2018; Anselmetti et al., 2022). This basin belongs to the oldest generation recognised so far. The sequence starts with sheared allochthonous bedrock slabs on top of the bedrock contact (Upper Marine Molasse), followed by gravel with molasse components and diamictic fines. The subsequent fine-grained lacustrine deposits are correlated with a nearby sequence that contains pollen assemblages assigned to the Holsteinian (Ellwanger et al., 2011; Hahne et al., 2012). The upper part of the Tannwald core comprises evidence for further glacial advances. In Lake Constance itself, as revealed by seismic surveys, up to 150 m of sediment has accumulated, the uppermost 24 m of which was recovered by a recent drilling campaign (Schaller et al., 2022). It consists of 12 m of coarse lacustrine sands of the late-glacial period, overlain by Holocene basin fines.
The basin of Wolfratshausen in Bavaria reveals multiple basal tills and lacustrine fines, evidence for a complex erosion and infill history attributed to three individual glaciations (Jerz, 1979). Fiebig et al. (2014) report a core taken in the Salzach basin containing ∼90 m of lacustrine deposits, sand and fines, on top of an Alpine till. Luminescence dating of the lake deposits indicates that the initial formation and filling of the overdeepened trough date to more than 220 ka.
5.2 Examples from northern central Europe
While tunnel valleys have also been described from the British Isles (e.g. Eyles and McCabe, 1989; Coughlan et al., 2020) as well as Poland (e.g. Salamon and Mendecki, 2021), we are here concentrating on the southern North Sea region including the bordering mainland (Denmark, northern Germany, the Netherlands).
For the Bornhöved tunnel valley, Piotrowski (1994) reconstructed a polygenetic evolution based on borehole evidence (Fig. 5). This trough, situated in a peripheral sink between two Permian salt diapirs, was presumably eroded during the Elsterian Glaciation (MIS 12, 478–424 ka) and was filled by fine-grained glaciolacustrine sediments during ice retreat and marine sediments during the Holsteinian Interglacial. These sediments were reworked and redeposited in the shape of a 200 m thick glaciotectonic melange by the first Saalian advance (MIS 10?, 374–337 ka). While the second Saalian advance had little impact, the tunnel valley was reactivated as a subglacial channel during the last, Weichselian, glaciation. In Vendsyssel, northern Denmark, tunnel valleys attributed to the main Weichselian advance (ca. 23–21 ka) are infilled by glaciolacustrine sand and fines (Sandersen et al., 2009). This implies that these tunnel valleys not only have formed rapidly but were also infilled in a few hundred years, or less, before the late-glacial marine inundation (ca. 18 ka).
In the North Sea basin, over 2200 tunnel valleys attributed to up to seven generations were identified using 3D seismic and magnetic data (Stewart and Lonergan, 2011; Ottesen et al., 2020). It appears that each of the seven generations of tunnel valleys, attributed mostly to the Middle Pleistocene, has been excavated and infilled during a separate cycle of ice-sheet advance and retreat (as already suggested by others, e.g. Kristensen et al., 2008), partially reaching into the following interglacial (e.g. Hepp et al., 2012; Janszen et al., 2013; Lang et al., 2015; Steinmetz et al., 2015). Moreau and Huuse (2014) interpret the infill process of tunnel valleys off the shore of the Netherlands to be separate from the incision. Rather than filled by subglacial deposits, Moreau and Huuse (2014) expect the infill sediments were supplied from the southeast by the Rhine–Meuse systems which consistently flowed towards the North Sea basin during glacial periods. This view has been challenged by Benvenuti et al. (2018) who inferred from provenance analysis that the infill of a large Elsterian tunnel valley consists mainly of reworked glacially derived sediment. This is in line with observations by Kirkham et al. (2021) demonstrating that over 40 % of the examined tunnel valleys in the North Sea between Scotland and Norway contain buried glacial landforms such as eskers, crevasse-squeeze ridges, glaciotectonic structures, and kettle holes. Hence, grounded ice must have played an active role not only in the incision but also in the (onsetting) infilling.
5.3 Synthesis
Fillings of overdeepenings in the northern Alpine foreland are clearly dominated by glacial and proglacial deposits. The typical sedimentary succession starts with glacigenic diamicts that are frequently referred to as tills. However, only a few studies (e.g. Buechi et al., 2017) investigated these deposits in sufficient detail to clearly identify them as subglacial ice-contact deposits (cf. Evans, 2007, and references therein), and thus their palaeo-glaciological significance is often not clear. These diamicts are overlain by extensive glaciolacustrine deposits, sands or fines, that at their base frequently contain dropstones. In some basins, several such infill cycles are recorded. Interglacial sediments are in most cases lacking or occur only near the ground surface, known exceptions being the successions of Thalgut, Meikirch, Uster, and Richterswil. This suggests that overdeepened troughs are in most cases infilled and silted up under still periglacial conditions, i.e. in a brief time interval (cf. Van Husen, 1979; Pomper et al., 2017). In contrast, overdeepenings incised during the last glaciation host a number of large and deep contemporaneous, i.e. interglacial, lakes that have not yet been infilled completely (Fig. 6). Whether this is due to exceptionally large basin sizes, comparatively small sediment influxes, or other geological or climatic factors is difficult to assess.
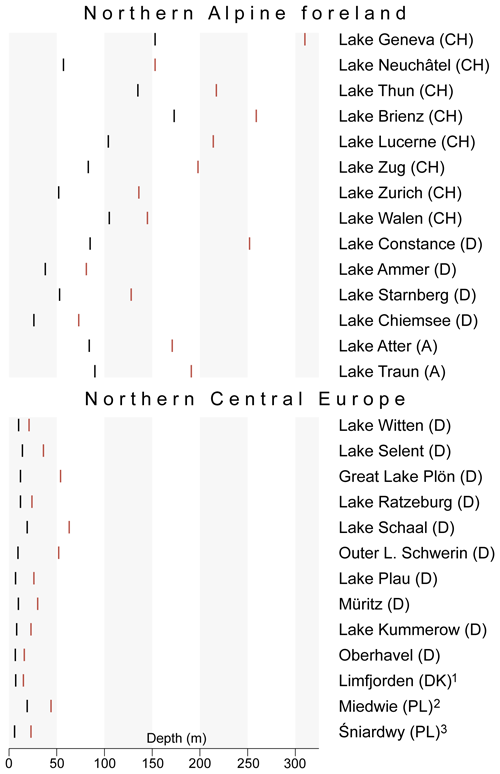
Figure 6Comparison of mean (black) and maximum (red) depths of large contemporaneous lakes in formerly glaciated areas in the northern Alpine foreland and northern central Europe (Switzerland, CH: BAFU, 2016; Germany, D: Nixdorf et al., 2004; Austria, A: Beiwl and Mühlmann, 2008; Denmark, DK: 1Riemann and Hoffmann, 1991; Poland, PL: 2Czerniejewski et al., 2004; 3Robbins and Jasinski, 1995).
The sediment filling of tunnel valleys and the related depositional processes in northern central Europe are less constrained and remain more poorly understood, although it appears that interglacial sediments are more frequently observed than in the Alpine realm. This could imply a longer “lifetime” of tunnel valleys before being entirely infilled. However, potential tunnel valleys of the last glaciation are completely infilled (e.g. Sandersen et al., 2009) or at least infilled to an extent that does not allow the formation of deep lakes anymore. While some large lakes also exist in the formerly glaciated areas of northern Germany and neighbouring countries, they are significantly shallower than their Alpine counterparts (Fig. 6); do not exhibit the typical elongated plan view trough morphology; and might not be products of overdeepening erosion at all but rather, for example, ice decay structures.
Foreland overdeepenings and tunnel valleys are connected to two very different types of ice masses but occur in generally similar palaeo-glaciological and topographic settings. They are formed at the termini of large ice bodies, where ice flow is little constrained, but the abundance of meltwater as well as the ice surface slope, and thus the subglacial pressure gradient towards the margin, are comparatively high. It is this pressurised meltwater that the incision of the spectacular subglacial landforms is mainly attributed to. Both foreland overdeepenings and tunnel valleys share the same morphological characteristics, including anastomosing courses, undulating longitudinal profiles with swells and adverse slopes, and a variety of different cross-sectional shapes. And, perhaps surprisingly, the absolute dimensions of both groups of troughs also appear not to be significantly different from each other.
However, a review of individual troughs and their fillings in central Europe, where these structures have the longest history of recognition and exploration, reveals some notable disparities. In the northern Alpine foreland, multiple well-studied cores exist that allow the characterisation of a typical overdeepening-fill succession in the shape of glacigenic diamicts overlain by glaciolacustrine deposits. In contrast, the infills of tunnel valleys and the related processes have received only little attention and remain rather poorly understood. Further, while the Middle Pleistocene sedimentary record would imply that foreland overdeepenings are more quickly infilled and silted up than tunnel valleys (i.e. typically before interglacial conditions ensue), the characteristics of present-day lakes suggest that the exact opposite has been the case following the last glaciation.
Detailed borehole records are an important tool to better characterise the formation and infilling process of overdeepenings and tunnel valleys (e.g. by process-oriented investigations of the basal “tills”). This is the central objective of the current International Scientific Continental Drilling Program project Drilling Overdeepened Alpine Valleys (DOVE; Anselmetti et al., 2022). Similar drilling projects targeting tunnel valleys are in dire need in order to better understand the overdeepening erosion and glaciation history of northern central Europe. We would like to stress that ongoing and future work of the respective scientific communities may be significantly facilitated and improved by more intensive exchange and discussion, as stimulated for example by the “Subglaziale Rinnen” workshop. The relevance of this exchange is highlighted by the socio-economic aspects of overdeepenings and tunnel valleys, especially in the context of radioactive waste disposal.
We utilised datasets by Magrani et al. (2020; https://www.sciencedirect.com/science/article/pii/S0277379120304455; last access: 13 January 2023) and Streuff et al. (2021; https://doi.org/10.1594/PANGAEA.937782).
This paper was conceptualised and the figures were prepared by LG, and the manuscript was written by LG and FP in a joint effort.
The contact author has declared that neither of the authors has any competing interests.
Publisher’s note: Copernicus Publications remains neutral with regard to jurisdictional claims in published maps and institutional affiliations.
This article is part of the special issue “Subglacial erosional landforms and their relevance for the long-term safety of a radioactive waste repository”. It is the result of a virtual workshop held in December 2021.
We thank the Swiss National Cooperative for the Disposal of Radioactive Waste (Nagra) for providing the base Quaternary digital elevation model (DEM) of Switzerland and the Geological Survey of Brandenburg, Germany, for permission to reproduce geological map data. We also thank editors Jörg Lang and Christopher Lüthgens as well as Simon Cook and an anonymous reviewer for their constructive comments.
This open-access publication was funded by the University of Freiburg.
This paper was edited by Jörg Lang and reviewed by Simon Cook and one anonymous referee.
Alley, R. B., Cuffey, K. M., Evenson, E. B., Strasser, J. C., Lawson, D. E., and Larson, G. J.: How glaciers entrain and transport basal sediment: physical constraints, Quaternary Sci. Rev., 16, 1017–1038, https://doi.org/10.1016/S0277-3791(97)00034-6, 1997.
Alley, R. B., Cuffey, K. M., and Zoet, L. K.: Glacial erosion: status and outlook, Ann. Glaciol., 60, 1–13, https://doi.org/10.1017/aog.2019.38, 2019.
Andersen, T. R., Huuse, M., Jørgensen, F., and Christensen, S.: Seismic investigations of buried tunnel valleys on-and offshore Denmark, Geol. Soc. Spec. Publ., 368, 129–144, https://doi.org/10.1144/SP368.14, 2012.
Andrews, J. T., Hardardóttir, J., Helgadóttir, G., Jennings, A. E., Geirsdóttir, Á., Sveinbjörnsdóttir, Á. E., Schoolfield, S., Kristjánsdóttir, G. B., Smith, L. M., Thorse, K., and Syvitski, J. P. M.: The N and W Iceland Shelf: insights into Last Glacial Maximum ice extent and deglaciation based on acoustic stratigraphy and basal radiocarbon AMS dates, Quaternary Sci. Rev., 19, 619–631, https://doi.org/10.1016/S0277-3791(99)00036-0, 2000.
Anselmetti, F. S., Drescher-Schneider, R., Furrer, H., Graf, H. R., Lowick, S. E., Preusser, F., and Riedi, M. A.: A ∼ 180,000 years sedimentation history of a perialpine overdeepened glacial trough (Wehntal, N-Switzerland), Swiss J. Geosci., 103, 345–361, https://doi.org/10.1007/s00015-010-0041-1, 2010.
Anselmetti, F. S., Bavec, M., Crouzet, C., Fiebig, M., Gabriel, G., Preusser, F., Ravazzi, C., and DOVE scientific team: Drilling Overdeepened Alpine Valleys (ICDP-DOVE): quantifying the age, extent, and environmental impact of Alpine glaciations, Sci. Dril., 31, 51–70, https://doi.org/10.5194/sd-31-51-2022, 2022.
Atkinson, N., Andriashek, L. D., and Slattery, S. R.: Morphological analysis and evolution of buried tunnel valleys in northeast Alberta, Canada, Quaternary Sci. Rev., 65, 53–72, https://doi.org/10.1016/j.quascirev.2012.11.031, 2013.
BAFU: Faktenblätter Zustand bezüglich Wasserqualität, https://www.bafu.admin.ch/bafu/de/home/themen/wasser/fachinformationen/zustand-der-gewaesser/zustand-der-seen/wasserqualitaet-der-seen.html (last access: 10 November 2022), 2016.
Bandou, D., Schlunegger, F., Kissling, E., Marti, U., Schwenk, M., Schläfli, P., Douillet, G., and Mair, D.: Three-dimensional gravity modelling of a Quaternary overdeepening fill in the Bern area of Switzerland discloses two stages of glacial carving, Sci. Rep., 12, 1–14, https://doi.org/10.1038/s41598-022-04830-x, 2022.
Barker, R. D. and Harker, D.: The location of the Stour buried tunnel-valley using geophysical techniques, Q. J. Eng. Geol., 17, 103–115, https://doi.org/10.1144/GSL.QJEG.1984.017.02.03, 1984.
Beiwl, C. and Mühlmann, H.: Atlas der natürlichen Seen Österreichs mit einer Fläche ≥50 ha Morphometrie – Typisierung – Trophie Stand 2005, Schriftenreihe des Bundesamtes für Wasserwirtschaft, Band 29, 147 pp., ISBN: 3-901605-29-0, 2008.
Benvenuti, A. and Moscariello, A.: High-resolution seismic geomorphology and stratigraphy of a tunnel valley confined ice-margin fan (Elsterian glaciation, Southern North Sea), Interpretation, 4, T461–T483, https://doi.org/10.1190/INT-2016-0026.1, 2016.
Benvenuti, A., Šegvić, B., and Moscariello, A.: Tunnel valley deposits from the southern North Sea – material provenance and depositional processes, Boreas, 47, 625–642, https://doi.org/10.1111/bor.12292, 2018.
Bertrand, S., Lange, C. B., Pantoja, S., Hughen, K., Van Tornhout, E., and Wellner, J. S.: Postglacial fluctuations of Cordillera Darwin glaciers (southernmost Patagonia) reconstructed from Almirantazgo fjord sediments. Quaternary Sci. Rev., 177, 265–275, https://doi.org/10.1016/j.quascirev.2017.10.029, 2017.
Bini, A., Buoncristiani, J. F., Couterrand, S., Ellwanger, D., Felber, M., Florineth, D., Graf, H. R., Keller, O., Kelly, M., and Schlüchter, C.: Die Schweiz während des letzteiszeitlichen Maximums (LGM) 1:500.000, Bundesamt für Landestopographie swisstopo, Wabern, Switzerland https://opendata.swiss/de/dataset/die-schweiz- wahrend-des-letzteiszeitlichen-maximums-lgm-1-500000 (last access: 10 January 2023), 2009.
Bosch, J. A., Bakker, M. A., Gunnink, J. L., and Paap, B. F.: Airborne electromagnetic measurements as basis for a 3D geological model of an Elsterian incision, Z. Dtsch. Ges. Geowiss., 160, 249–258, https://doi.org/10.1127/1860-1804/2009/0160-0258, 2009.
Boulton, G. S., Hagdorn, M., Maillot, P. B., and Zatsepin, S.: Drainage beneath ice sheets: groundwater–channel coupling, and the origin of esker systems from former ice sheets, Quaternary Sci. Rev., 28, 621–638, https://doi.org/10.1016/j.quascirev.2008.05.009, 2009.
Boyd, B. L., Anderson, J. B., Wellner, J. S., and Fernandez, R. A.: The sedimentary record of glacial retreat, Marinelli Fjord, Patagonia: Regional correlations and climate ties, Mar. Geol., 255, 165–178, https://doi.org/10.1016/j.margeo.2008.09.001, 2008.
Boyd, R., Scott, D. B., and Douma, M.: Glacial tunnel valleys and Quaternary history of the outer Scotian shelf, Nature, 333, 61–64, https://doi.org/10.1038/333061a0, 1988.
Bradwell, T., Stoker, M. S., Golledge, N. R., Wilson, C. K., Merritt, J. W., Long, D., Everest, J. D., Hestvik, O. B., Stevenson, A. G., and Hubbard, A. L.: The northern sector of the last British Ice Sheet: maximum extent and demise, Earth-Sci. Rev., 88, 207–226, https://doi.org/10.1016/J.EARSCIREV.2008.01.008, 2008.
Brandt, A. C.: Erkundung des alpinen, glazial-übertieften Basadingen-Beckens mithilfe von P-Wellen-Seismik, Bachelor thesis, Leibniz University Hannover, 2020.
Buechi, M. W., Frank, S. M., Graf, H. R., Menzies, J., and Anselmetti, F. S.: Subglacial emplacement of tills and meltwater deposits at the base of overdeepened bedrock troughs, Sedimentology, 64, 658–685, https://doi.org/10.1111/sed.12319, 2017.
Buechi, M. W., Graf, H. R., Haldimann, P., Lowick, S. E., and Anselmetti, F. S.: Multiple Quaternary erosion and infill cycles in overdeepened basins of the northern Alpine foreland, Swiss J. Geosci., 111, 1–34, https://doi.org/10.1007/s00015-017-0289-9, 2018.
Burschil, T., Buness, H., Tanner, D. C., Wielandt-Schuster, U., Ellwanger, D., and Gabriel, G.: High-resolution reflection seismics reveal the structure and the evolution of the Quaternary glacial Tannwald Basin, Near Surf. Geophys., 16, 593–610, https://doi.org/10.1002/nsg.12011, 2018.
Burschil, T., Buness, H., Gabriel, G., Tanner, D. C., and Reitner, J. M.: Unravelling the shape and stratigraphy of a glacially-overdeepened valley with reflection seismic: the Lienz Basin (Austria), Swiss J. Geosci., 112, 341–355, https://doi.org/10.1007/s00015-019-00339-0, 2019.
Cameron, T. D. J., Stoker, M. S., and Long, D.: The history of Quaternary sedimentation in the UK sector of the North Sea Basin, J. Geol. Soc., 144, 43–58, https://doi.org/10.1144/gsjgs.144.1.0043, 1987.
Carrivick, J. L., Davies, B. J., James, W. H. M., Quincey, D. J., and Glasser, N. F.: Distributed ice thickness and glacier volume in southern South America, Global Planet. Change, 146, 122–132, https://doi.org/10.1016/j.gloplacha.2016.09.010, 2016.
Clark, P. U. and Walder, J. S.: Subglacial drainage, eskers, and deforming beds beneath the Laurentide and Eurasian ice sheets, Geol. Soc. Am. Bull., 106, 304–314, https://doi.org/10.1130/0016-7606(1994)106<0304:SDEADB>2.3.CO;2, 1994.
Clerc, S., Buoncristiani, J. F., Guiraud, M., Vennin, E., Desaubliaux, G., and Portier, E.: Subglacial to proglacial depositional environments in an Ordovician glacial tunnel valley, Alnif, Morocco, Palaeogeogr. Palaeocl., 370, 127–144, https://doi.org/10.1016/j.palaeo.2012.12.002, 2013.
Cofaigh, C. Ó.: Tunnel valley genesis, Prog. Phys. Geogr., 20, 1–19, https://doi.org/10.1177/030913339602000101, 1996.
Cohen, K. M. and Gibbard, P. L.: Global chronostrati-graphical correlation table for the last 2.7 million years, version 2019 QI-500, Quatern. Int., 500, 20–31, https://doi.org/10.1016/j.quaint.2019.03.009, 2019.
Cook, S. J. and Swift, D. A.: Subglacial basins: Their origin and importance in glacial systems and landscapes, Earth-Sci. Rev., 115, 332–372, https://doi.org/10.1016/j.earscirev.2012.09.009, 2012.
Coughlan, M., Tóth, Z., Van Landeghem, K. J. J., Mccarron, S., Wheeler, A. J.: Formational history of the Wicklow Trough: a marine-transgressed tunnel valley revealing ice flow velocity and retreat rates for the largest ice stream draining the late-Devensian British–Irish Ice Sheet, J. Quateranry Sci., 35, 907–919, https://doi.org/10.1002/jqs.3234, 2020.
Cummings, D. I., Russell, H. A. J., and Sharpe, D. R.: Buried-valley aquifers in the Canadian Prairies: geology, hydrogeology, and origin, Can. J. Earth Sci., 49, 987–1004, https://doi.org/10.1139/e2012-041, 2012.
Czerniejewski, P., Filipiak, J., Poleszczuk, G., and Wawrzyniak, W.: Selected biological characteristics of the catch-available part of population of vendace, Coregonus albula (L.) from Lake Miedwie, Poland, Acta Ichthyologica et Piscatoria, 34, 219–233, https://doi.org/10.3750/AIP2004.34.2.09, 2004.
Dehnert, A., Lowick, S. E., Preusser, F., Anselmetti, F. S., Drescher-Schneider, R., Graf, H. R., Heller, F., Horstmeyer, H., Kemna, H. A., and Nowaczyk, N. R.: Evolution of an overdeepened trough in the northern Alpine Foreland at Niederweningen, Switzerland, Quaternary Sci. Rev., 34, 127–145, https://doi.org/10.1016/j.quascirev.2011.12.015, 2012.
Destombes, J.-P., Shephard-Thorn, E. R., Redding, J. H., and Morzadec-Kerfourn, M. T.: A buried valley system in the Strait of Dover, Philos. T. Roy. Soc. A, 279, 243–253, https://doi.org/10.1098/rsta.1975.0056, 1975.
Dürst Stucki, M. and Schlunegger, F.: Identification of erosional mechanisms during past glaciations based on a bedrock surface model of the central European Alps, Earth Planet. Sc. Lett., 384, 57–70, https://doi.org/10.1016/j.epsl.2013.10.009, 2013.
Dürst Stucki, M., Reber, R., and Schlunegger, F.: Subglacial tunnel valleys in the Alpine foreland: an example from Bern, Switzerland, Swiss J. Geosci., 103, 363–374, https://doi.org/10.1007/s00015-010-0042-0, 2010.
Egholm, D. L., Pedersen, V. K., Knudsen, M. F., and Larsen, N. K.: Coupling the flow of ice, water, and sediment in a glacial landscape evolution model, Geomorphology, 141, 47–66, https://doi.org/10.1016/j.geomorph.2011.12.019, 2012.
Ellwanger, D., Wielandt-Schuster, U., Franz, M., and Simon, T.: The Quaternary of the southwest German Alpine Foreland (Bodensee-Oberschwaben, Baden-Württemberg, Southwest Germany), E&G Quaternary Sci. J., 60, 22, https://doi.org/10.3285/eg.60.2-3.07, 2011.
Evans, D. J. A.: Glacial landforms, sediments – Tills, Encyclopedia of Quaternary Science, 959–975, https://doi.org/10.1016/B0-44-452747-8/00092-2, 2007.
Eyles, N. and McCabe, A. M.: Glaciomarine facies within subglacial tunnel valleys: the sedimentary record of glacioisostatic downwarping in the Irish Sea Basin, Sedimentology, 36, 431–448, https://doi.org/10.1111/j.1365-3091.1989.tb00618.x, 1989.
Fiebig, M., Herbst, P., Drescher-Schneider, R., Lüthgens, C., Lomax, J., and Doppler, G.: Some remarks about a new Last Glacial record from the western Salzach foreland glacier basin (Southern Germany), Quatern. Int., 328, 107–119, https://doi.org/10.1016/j.quaint.2013.12.048, 2014.
Finckh, P., Kelts, K., and Lambert, A.: Seismic stratigraphy and bedrock forms in perialpine lakes, Geol. Soc. Am. Bull., 95, 1118–1128, https://doi.org/10.1130/0016-7606(1984)95<1118:SSABFI>2.0.CO;2, 1984.
Gao, C.: Buried bedrock valleys and glacial and subglacial meltwater erosion in southern Ontario, Canada, Can. J. Earth Sci., 48, 801–818, https://doi.org/10.1139/e10-104, 2011.
Gegg, L., Buechi, M. W., Ebert, A., Deplazes, G., Madritsch, H., and Anselmetti, F. S.: Brecciation of glacially overridden palaeokarst (Lower Aare Valley, northern Switzerland): result of subglacial water-pressure peaks?, Boreas, 49, 813–827, https://doi.org/10.1111/bor.12457, 2020.
Gegg, L., Deplazes, G., Keller, L., Madritsch, H., Spillmann, T., Anselmetti, F. S., and Buechi, M. W.: 3D morphology of a glacially overdeepened trough controlled by underlying bedrock geology, Geomorphology, 394, 107950, https://doi.org/10.1016/j.geomorph.2021.107950, 2021.
Giglio, C., Benetti, S., Sacchetti, F., Lockhart, E., Hughes Clarke, J., Plets, R., van Landeghem, K., Ó Cofaigh, C., Scourse, J., and Dunlop, P.: A Late Pleistocene channelized subglacial meltwater system on the Atlantic continental shelf south of Ireland, Boreas, 51, 118–135, https://doi.org/10.1111/bor.12536, 2022.
Götze, H. J. G., Giszas, V., Hese, F., Kirsch, R. K., and Schmidt, S.: The ice age paleo-channel Ellerbeker Rinne an integrated 3D gravity study, Z. Dtsch. Ges. Geowiss., 160, 279–293, https://doi.org/10.1127/1860-1804/2009/0160-0279, 2009.
Graf, H. R.: Stratigraphie von Mittel- und Spätpleistozän in der Nordschweiz, Beiträge zur Geologischen Karte der Schweiz, NF-168, 198 pp., 2009.
Gregory, A. R.: The formation and development of proglacial overdeepenings at a contemporary Piedmont lobe glacier: Skeiðarárjökull, South East Iceland, PhD thesis, Newcastle University, http://theses.ncl.ac.uk/jspui/handle/10443/1704 (last access: 4 December 2022), 2012.
Haeberli, W., Linsbauer, A., Cochachin, A., Salazar, C., and Fischer, U.: On the morphological characteristics of overdeepenings in high-mountain glacier beds, Earth Surf. Proc. Land., 41, 1980–1990, https://doi.org/10.1002/esp.3966, 2016.
Hahne, J., Ellwanger, D., Franz, M., Stritzke, R., and Wielandt-Schuster, U.: Pollenanalytische Untersuchungsergebnisse aus dem Baden-Württembergischen Rheinsystem Oberrheingraben, Hochrhein, Oberschwaben – eine Zusammenfassung des aktuellen Kenntnisstandes, LGRB Informationen, 26, 119–154, 2012.
Heim, A.: Handbuch der Gletscherkunde, J. Engelhorn, 1885.
Hepp, D. A., Hebbeln, D., Kreiter, S., Keil, H., Bathmann, C., Ehlers, J., and Tobias Mörz, T.: An east–west-trending Quaternary tunnel valley in the south-eastern North Sea and its seismic–sedimentological interpretation, J. Quaternary Sci., 27, 844–853, https://doi.org/10.1002/jqs.2599, 2012.
Herman, F., Beaud, F., Champagnac, J.-D., Lemieux, J.-M., and Sternai, P.: Glacial hydrology and erosion patterns: a mechanism for carving glacial valleys, Earth Planet. Sc. Lett., 310, 498–508, https://doi.org/10.1016/j.epsl.2011.08.022, 2011.
Herman, F., Beyssac, O., Brughelli, M., Lane, S. N., Leprince, S., Adatte, T., Lin, J. Y. Y., Avouac, J.-P., and Cox, S. C.: Erosion by an Alpine glacier, Science, 350, 193–195, https://doi.org/10.1126/science.aab2386, 2015.
Hsü, K. J. and Kelts, K. R. (Eds.): Quaternary geology of Lake Zurich, Contributions to Sedimentary Geology, 13, 210 pp., ISBN: 978-3-510-57013-3, 1984.
Huuse, M. and Lykke-Andersen, H.: Overdeepened Quaternary valleys in the eastern Danish North Sea: morphology and origin, Quaternary Sci. Rev., 19, 1233–1253, https://doi.org/10.1016/S0277-3791(99)00103-1, 2000.
James, W. H. M., Carrivick, J. L., Quincey, D. J., and Glasser, N. F.: A geomorphology based reconstruction of ice volume distribution at the Last Glacial Maximum across the Southern Alps of New Zealand, Quaternary Sci. Rev., 219, 20–35, https://doi.org/10.1016/j.quascirev.2019.06.035, 2019.
Janszen, A., Spaak, M., and Moscariello, A.: Effects of the substratum on the formation of glacial tunnel valleys: an example from the Middle Pleistocene of the southern North Sea Basin, Boreas, 41, 629–643, https://doi.org/10.1111/j.1502-3885.2012.00260.x, 2012.
Janszen, A., Moreau, J., Moscariello, A., Ehlers, J., and Kröger, J.: Time-transgressive tunnel-valley infill revealed by a threedimensional sedimentary model, Hamburg, north-west Germany, Sedimentology, 60, 693–719, https://doi.org/10.1111/j.1365-3091.2012.01357.x, 2013.
Jennings, C. E.: Terrestrial ice streams – a view from the lobe, Geomorphology, 75, 100–124, https://doi.org/10.1016/j.geomorph.2005.05.016, 2006.
Jentzsch, A.: Über die Bildung der Preussischen Seen, Z. Dtsch. Ges. Geowiss., 36, 699–702, 1884.
Jerz, H.: Das Wolfratshausener Becken: seine glaziale Anlage und Übertiefung, E&G Quaternary Sci. J., 29, 63–70, https://doi.org/10.23689/fidgeo-1646, 1979.
Jordan, P.: Analysis of overdeepened valleys using the digital elevation model of the bedrock surface of Northern Switzerland, Swiss J. Geosci., 103, 375–384, https://doi.org/10.1007/s00015-010-0043-z, 2010.
Jørgensen, F. and Sandersen, P. B. E.: Buried and open tunnel valleys in Denmark–erosion beneath multiple ice sheets, Quaternary Sci. Rev., 25, 1339–1363, https://doi.org/10.1016/j.quascirev.2005.11.006, 2006.
Kearsey, T. I., Lee, J. R., Finlayson, A., Garcia-Bajo, M., and Irving, A. A. M.: Examining the geometry, age and genesis of buried Quaternary valley systems in the Midland Valley of Scotland, UK, Boreas, 48, 658–677, https://doi.org/10.1111/bor.12364, 2019.
Kehew, A. E., Piotrowski, J. A., and Jørgensen, F.: Tunnel valleys: Concepts and controversies – A review, Earth-Sci. Rev., 113, 33–58, https://doi.org/10.1016/j.earscirev.2012.02.002, 2012.
Kirkham, J. D., Hogan, K. A., Larter, R. D., Self, E., Games, K., Huuse, M., Stewart, M. A., Ottesen, D., Arnold, N. S., and Dowdeswell, J. A.: Tunnel valley infill and genesis revealed by high-resolution 3-D seismic data, Geology, 49, 1516–1520, https://doi.org/10.1130/G49048.1, 2021.
Kristensen, T. B., Piotrowski, J. A., Huuse, M., Clausen, O. R., and Hamberg, L.: Time-transgressive tunnel valley formation indicated by infill sediment structure, North Sea – the role of glaciohydraulic supercooling, Earth Surf. Proc. Land., 33, 546–559, https://doi.org/10.1002/esp.1668, 2008.
Kühni, A. and Pfiffner, O.-A.: The relief of the Swiss Alps and adjacent areas and its relation to lithology and structure: topographic analysis from a 250-m DEM, Geomorphology, 41, 285–307, https://doi.org/10.1016/S0169-555X(01)00060-5, 2001.
Kunst, F. and Deze, J. F.: The case history of a high-resolution seismic survey in the central North Sea, in: Offshore Tech. Conf., Houston, Texas, 6–9 May 1985, https://doi.org/10.4043/4968-MS, 1985.
Kuster, H. and Meyer, K.-D.: Glaziäre Rinnen im mittleren und nördlichen Niedersachsen, E&G Quaternary Sci. J., 29, 135–156, https://doi.org/10.3285/eg.29.1.12, 1979.
Lang, J., Böhner, U., Polom, U., Serangeli, J., and Winsemann, J.: The Middle Pleistocene tunnel valley at Schöningen as a Paleolithic archive, J. Hum. Evol., 89, 18–26, https://doi.org/10.1016/j.jhevol.2015.02.004, 2015.
Lelandais, T., Ravier, É., Pochat, S., Bourgeois, O., Clark, C., Mourgues, R., and Strzerzynski, P.: Modelled subglacial floods and tunnel valleys control the life cycle of transitory ice streams, The Cryosphere, 12, 2759–2772, https://doi.org/10.5194/tc-12-2759-2018, 2018.
LGRB: Lithostratigraphische Entwicklung des Baden-Württembergischen Rheingletschergebiets: Übertiefste Becken- und Moränen-Landschaft, LGRB-Fachbericht 2015/4, 86 pp., https://produkte.lgrb-bw.de/schriftensuche/sonstige-produkte/?aid=9 (last access: 10 January 2023), 2015.
Lister, G. S.: Lithostratigraphy of Zübo sediments, Contributions to sedimentology, 13, 31–58, 1984a.
Lister, G. S.: Deglaciation of the Lake Zurich area: a model based on the sedimentological record, Contributions to sedimentology, 13, 177–185, 1984b.
Livingstone, S. J. and Clark, C. D.: Morphological properties of tunnel valleys of the southern sector of the Laurentide Ice Sheet and implications for their formation, Earth Surf. Dynam., 4, 567–589, https://doi.org/10.5194/esurf-4-567-2016, 2016.
Lohrberg, A., Schwarzer, K., Unverricht, D., Omlin, A., and Krastel, S.: Architecture of tunnel valleys in the southeastern North Sea: new insights from high-resolution seismic imaging, J. Quaternary Sci., 35, 892–906, https://doi.org/10.1002/jqs.3244, 2020.
Lutz, R., Kalka, S., Gaedicke, C., Reinhardt, L., and Winsemann, J.: Pleistocene tunnel valleys in the German North Sea: spatial distribution and morphology, Z. Dtsch. Ges. Geowiss., 160, 225–235, https://doi.org/10.1127/1860-1804/2009/0160-0225, 2009.
Madsen, V.: Terrainformerne paa Skovbjerg Bakkeø, Danmarks Geologiske Undersøgelse, IV. Række, 1, 1–24, https://doi.org/10.34194/raekke4.v1.6971, 1921.
Magnússon, E., Pálsson, F., Björnsson, H., and Guðmundsson, S.: Removing the ice cap of Öræfajökull central volcano, SE-Iceland: mapping and interpretation of bedrock topography, ice volumes, subglacial troughs and implications for hazards assessments, Jökull, 62, 131–150, 2012.
Magrani, F., Valla, P. G., Gribenski, N., and Serra, E.: Glacial overdeepenings in the Swiss Alps and foreland: Spatial distribution and morphometrics, Quaternary Sci. Rev., 243, 106483, https://doi.org/10.1016/j.quascirev.2020.106483, 2020 (data available at: https://www.sciencedirect.com/science/article/pii/S0277379120304455, last access: 13 January 2023).
Margold, M., Stokes, C. R., and Clark, C. D.: Ice streams in the Laurentide Ice Sheet: Identification, characteristics and comparison to modern ice sheets, Earth-Sci. Rev., 143, 117–146, https://doi.org/10.1016/j.earscirev.2015.01.011, 2015.
Moernaut, J., De Batist, M., Heirman, K., Van Daele, M., Pino, M., Brümmer, R., and Urrutia, R.: Fluidization of buried mass-wasting deposits in lake sediments and its relevance for paleoseismology: results from a reflection seismic study of lakes Villarrica and Calafquén (South-Central Chile), Sediment. Geol., 213, 121–135, https://doi.org/10.1016/j.sedgeo.2008.12.002, 2009.
Moreau, J. and Huuse, M.: Infill of tunnel valleys associated with landward-flowing ice sheets: The missing Middle Pleistocene record of the NW European rivers?, Geochem. Geophy. Geosy., 15, 1–9, https://doi.org/10.1002/2013GC005007, 2014.
Nitsche, F. O., Monin, G., Marillier, F., Graf, H., and Ansorge, J.: Reflection seismic study of Cenozoic sediments in an overdeepened valley of northern Switzerland: the Birrfeld area, Eclogae Geol. Helv., 94, 363–371, https://doi.org/10.5169/seals-168901, 2001.
Nixdorf, B., Hemm, M., Hoffmann, A., and Richter, P.: Dokumentation von Zustand und Entwicklung der wichtigsten Seen Deutschlands, Umweltbundesamt Forschungsbericht 299 24 274, 274 pp., 2004.
Ottesen, D., Stewart, M., Brönner, M., and Batchelor, C. L.: Tunnel valleys of the central and northern North Sea (56∘ N to 62∘ N): Distribution and characteristics, Mar. Geol., 425, 106199, https://doi.org/10.1016/j.margeo.2020.106199, 2020.
Patton, H., Swift, D. A., Clark, C. D., Livingstone, S. J., and Cook, S. J.: Distribution and characteristics of overdeepenings beneath the Greenland and Antarctic ice sheets: Implications for overdeepening origin and evolution, Quaternary Sci. Rev., 148, 128–145, https://doi.org/10.1016/j.quascirev.2016.07.012, 2016.
Penck, A. and Brückner, E.: Die Alpen im Eiszeitalter, Tauchnitz, Leipzig, 1909.
Pietsch, J. and Jordan, P.: Digitales Höhenmodell Basis Quartär der Nordschweiz – Version 2014 und ausgewählte Auswertungen, Nagra Arbeitsbericht NAB 14-02, 69 pp., https://nagra.ch/downloads/arbeitsbericht-nab-14-02/ (last access: 10 January 2023), 2014.
Piotrowski, J. A.: Tunnel-valley formation in northwest Germany—geology, mechanisms of formation and subglacial bed conditions for the Bornhöved tunnel valley, Sediment. Geol., 89, 107–141, https://doi.org/10.1016/0037-0738(94)90086-8, 1994.
Pomper, J., Salcher, B. C., Eichkitz, C., Prasicek, G., Lang, A., Lindner, M., and Götz, J.: The glacially overdeepened trough of the Salzach Valley, Austria: Bedrock geometry and sedimentary fill of a major Alpine subglacial basin, Geomorphology, 295, 147–158, https://doi.org/10.1016/j.geomorph.2017.07.009, 2017.
Praeg, D.: Buried fluvial channels: 3D-seismic geomorphology, in: Glaciated Continental Margins, Springer, 162–163, https://doi.org/10.1007/978-94-011-5820-6_65, 1997.
Preusser, F., Drescher-Schneider, R., Fiebig, M., and Schlüchter, C.: Re-interpretation of the Meikirch pollen record, Swiss Alpine Foreland, and implications for Middle Pleistocene chrono-stratigraphy, J. Quaternary Sci., 20, 607–620, https://doi.org/10.1002/jqs.930, 2005.
Preusser, F., Reitner, J. M., and Schlüchter, C.: Distribution, geometry, age and origin of overdeepened valleys and basins in the Alps and their foreland, Swiss J. Geosci., 103, 407–426, https://doi.org/10.1007/s00015-010-0044-y, 2010.
Preusser, F., Graf, H. R., Keller, O., Krayss, E., and Schlüchter, C.: Quaternary glaciation history of northern Switzerland, E&G Quaternary Sci. J., 60, 21, https://doi.org/10.3285/eg.60.2-3.06, 2011.
Quillmann, U., Jennings, A., and Andrews, J.: Reconstructing Holocene palaeoclimate and palaeoceanography in Ìsafjarðardjúp, northwest Iceland, from two fjord records overprinted by relative sea-level and local hydrographic changes, J. Quaternary Sci., 25, 1144–1159, https://doi.org/10.1002/jqs.1395, 2010.
Reitner, J. M., Gruber, W., Römer, A., and Morawetz, R.: Alpine overdeepenings and paleo-ice flow changes: an integrated geophysical-sedimentological case study from Tyrol (Austria), Swiss J. Geosci., 103, 385–405, https://doi.org/10.1007/s00015-010-0046-9, 2010.
Riemann, B. and Hoffmann, E.: Ecological consequences of dredging and bottom trawling in the Limfjord, Denmark, Mar. Ecol. Prog. Ser., 69, 171–178, https://doi.org/10.3354/meps069171, 1991.
Rignot, E., Mouginot, J., and Scheuchl, B.: Ice flow of the Antarctic ice sheet, Science, 333, 1427–1430, https://doi.org/10.1126/science.1208336, 2011.
Robbins, J. A. and Jasinski, A. W.: Chernobyl fallout radionuclides in lake Sniardwy, Poland, J. Environ. Radioactiv., 26, 157–184, https://doi.org/10.1016/0265-931X(94)00005-H, 1995.
Russell, H. A. J., Arnott, R. W. C., and Sharpe, D. R.: Evidence for rapid sedimentation in a tunnel channel, Oak Ridges Moraine, southern Ontario, Canada, Sediment. Geol., 160, 33–55, https://doi.org/10.1016/S0037-0738(02)00335-4, 2003.
Salamon, T. and Mendecki, M.: A rare signature of subglacial outburst floods developed along structural ice weaknesses in the southern sector of the Scandinavian Ice Sheet during the Drenthian Glaciation, S Poland, Geomorphology, 378, 107593, https://doi.org/10.1016/j.geomorph.2021.107593, 2021.
Sandersen, P. B. E., Jørgensen, F., Larsen, N. K., Westergaard, J. H., and Auken, E.: Rapid tunnel valley formation beneath the receding Late Weichselian ice sheet in Vendsyssel, Denmark, Boreas, 38, 834–851, https://doi.org/10.1111/j.1502-3885.2009.00105.x, 2009.
Schaller, S., Böttcher, M. E., Buechi, M. W., Epp, L. S., Fabbri, S. C., Gribenski, N., Harms, U., Krastel, S., Liebezeit, A., Lindhorst, K., Marxen, H., Raschke, U., Schleheck, D., Schmiedinger, I., Schwalb, A., Vogel, H., Wessels, M., and Anselmetti, F. S.: Postglacial evolution of Lake Constance: sedimentological and geochemical evidence from a deep-basin sediment core, Swiss J. Geosci., 115, 7, https://doi.org/10.1186/s00015-022-00412-1, 2022.
Schlüchter, C.: Übertiefte Talabschnitte im Berner Mittelland zwischen Alpen und Jura (Schweiz), E&G Quaternary Sci. J., 29, 101—113, https://doi.org/10.23689/fidgeo-921, 1979.
Schlüchter, C.: The most complete Quaternary record of the Swiss Alpine Foreland, Palaeogeogr. Palaeocl., 72, 141–146, https://doi.org/10.1016/0031-0182(89)90138-7, 1989.
Schoof, C. and Hewitt, I.: Ice-sheet dynamics, Annu. Rev. Fluid Mech., 45, 217–239, https://doi.org/10.1146/annurev-fluid-011212-140632, 2013.
Schwenk, M. A., Schläfli, P., Bandou, D., Gribenski, N., Douillet, G. A., and Schlunegger, F.: From glacial erosion to basin overfill: a 240 m-thick overdeepening–fill sequence in Bern, Switzerland, Sci. Dril., 30, 17–42, https://doi.org/10.5194/sd-30-17-2022, 2022.
Smith, L. N.: Late Pleistocene stratigraphy and implications for deglaciation and subglacial processes of the Flathead Lobe of the Cordilleran Ice Sheet, Flathead Valley, Montana, USA, Sediment. Geol., 165, 295–332, https://doi.org/10.1016/j.sedgeo.2003.11.013, 2004.
Stackebrandt, W., Ludwig, A. O., and Ostaficzuk, S.: Base of Quaternary deposits of the Baltic Sea depression and adjacent areas (map 2), Brandenburgische Geowiss. Beitr., 8, 13–19, 2001.
Steinmetz, D., Winsemann, J., Brandes, C., Siemon, B., Ullmann, A., Wiederhold, H., and Meyer, U.: Towards an improved geological interpretation of airborne electromagnetic data: a case study from the Cuxhaven tunnel valley and its Neogene host sediments (northwest Germany), Neth. J. Geosci., 94, 201–227, https://doi.org/10.1017/njg.2014.39, 2015.
Stewart, M. A. and Lonergan, L.: Seven glacial cycles in the middle-late Pleistocene of northwest Europe: Geomorphic evidence from buried tunnel valleys, Geology, 39, 283–286, https://doi.org/10.1130/G31631.1, 2011.
Stewart, M. A., Lonergan, L., and Hampson, G.: 3D seismic analysis of buried tunnel valleys in the central North Sea: morphology, cross-cutting generations and glacial history, Quaternary Sci. Rev., 72, 1–17, https://doi.org/10.1016/j.quascirev.2013.03.016, 2013.
Streuff, K. T., Ó Cofaigh, C., and Wintersteller, P.: A GIS Database of Submarine Glacial Landforms and Sediments on Arctic Continental Shelves, PANGAEA [data set], https://doi.org/10.1594/PANGAEA.937782, 2021.
Streuff, K. T., Ó Cofaigh, C., and Wintersteller, P.: GlaciDat – a GIS database of submarine glacial landforms and sediments in the Arctic, Boreas, 51, 517–531, https://doi.org/10.1111/bor.12577, 2022.
Tezkan, B. M., Helwig, S. L., and Bergers, R.: Time domain electromagnetic (TEM) measurements on a buried subglacial valley in Northern Germany by using a large transmitter size and a high current, Z. Dtsch. Ges. Geowiss., 160, 271–278, https://doi.org/10.1127/1860-1804/2009/0160-0271, 2009.
Ugelvig, S. V., Egholm, D. L., and Iverson, N. R.: Glacial landscape evolution by subglacial quarrying: A multiscale computational approach, J. Geophys. Res.-Earth, 121, 2042–2068, https://doi.org/10.1002/2016JF003960, 2016.
Ussing, N. V.: Danmarks Geologi i almenfatteligt Omrids. Anden udgave, Danmarks Geologiske Undersøgelse III. Række, 2, 358 pp., https://doi.org/10.34194/raekke3.v2.6907, 1904.
van der Vegt, P., Janszen, A., and Moscariello, A.: Tunnel valleys: current knowledge and future perspectives, Geol. Soc. Spec. Publ., 368, 75–97, https://doi.org/10.1144/SP368.13, 2012.
Van Husen, D.: Verbreitung, Ursachen und Füllung glazial übertiefter Talabschnitte an Beispielen aus den Ostalpen, E&G Quaternary Sci. J., 29, 9–22, https://doi.org/10.3285/eg.29.1.02, 1979.
Vesely, F. F., Assine, M. L., França, A. B., Paim, P. S., and Rostirolla, S. P.: Tunnel-valley fills in the Paraná Basin and their implications for the extent of late Paleozoic glaciation in SW Gondwana, J. S. Am. Earth Sci., 106, 102969, https://doi.org/10.1016/j.jsames.2020.102969, 2021.
Waltham, T.: Lötschberg tunnel disaster, 100 years ago, Q. J. Eng. Geol. Hydroge., 41, 131–136, https://doi.org/10.1144/1470-9236/06-033, 2008.
Welten, M.: Pollenanalytische Untersuchungen im Jüngeren Quartär des nördlichen Alpenvorlandes der Schweiz, Beiträge zur Geologischen Karte der Schweiz, NF-156, 174 pp., 1982.
Welten, M.: Neue pollenanalytische Ergebnisse über das Jüngere Quartär des nördlichen Alpenvorlandes der Schweiz (Mittel- und Jungpleistozän), Beiträge zur Geologischen Karte der Schweiz, NF-162, 40 pp., 1988.
Wildi, W.: Isohypsenkarte der quartären Felstäler in der Nord-und Ostschweiz, mit kurzen Erläuterungen, Eclogae Geol. Helv., 77, 541–551, https://doi.org/10.5169/seals-165521, 1984.
Woodland, A. W.: The buried tunnel-valleys of East Anglia, P. Yorks. Geol. Soc., 37, 521–578, https://doi.org/10.1144/pygs.37.4.521, 1970.
Wright, H. E.: Tunnel valleys, glacial surges, and subglacial hydrology of the Superior Lobe, Minnesota, Geol. Soc. Mem., 136, 251–276, https://doi.org/10.1130/MEM136-p251, 1973.
Wyssling, G.: Die Ur-Sihl floss einst ins Reusstal, Zur Geologie des Sihltales zwischen Schindellegi und Sihlbrugg, Verein Pro Sihltal, 52, 1–14, 2002.
Wyssling, L. and Wyssling, G.: Interglaziale Seeablagerungen in einer Bohrung bei Uster (Kt. Zürich), Eclogae Geol. Helv., 71, 357–375, https://doi.org/10.5169/seals-164737, 1978.
- How to cite
- Abstract
- Kurzfassung
- Introduction
- History of recognition and state-of-the-art research
- Geological and glaciological settings
- Morphological comparisons
- Post-erosional history
- Conclusions
- Data availability
- Author contributions
- Competing interests
- Disclaimer
- Special issue statement
- Acknowledgements
- Financial support
- Review statement
- References
- How to cite
- Abstract
- Kurzfassung
- Introduction
- History of recognition and state-of-the-art research
- Geological and glaciological settings
- Morphological comparisons
- Post-erosional history
- Conclusions
- Data availability
- Author contributions
- Competing interests
- Disclaimer
- Special issue statement
- Acknowledgements
- Financial support
- Review statement
- References