the Creative Commons Attribution 4.0 License.
the Creative Commons Attribution 4.0 License.
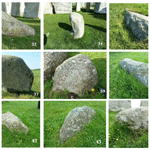
A bluestone boulder at Stonehenge: implications for the glacial transport theory
John, B. S.: A bluestone boulder at Stonehenge: implications for the glacial transport theory, E&G Quaternary Sci. J., 73, 117–134, https://doi.org/10.5194/egqsj-73-117-2024, 2024.
There has been considerable dispute over the mode of transport of the Stonehenge bluestones from their multiple sources in West Wales. For a century most archaeologists have accepted that the stones were transported by humans, but a number of earth scientists have taken the view that they were entrained and transported to Salisbury Plain by glacier ice. There is remarkably little evidence in support of either theory, and for this reason any new description of a possible glacial clast found at or near the stone monument is of potentially great importance. A small bullet-shaped boulder of welded tuff was found in a Stonehenge excavation in 1924, and apart from a brief examination by geologists from the Institute of Geological Sciences (IGS) around 1970, it has been stored out of sight and out of mind. Its geological source is uncertain. Following a detailed examination of its shape and surface characteristics it is now proposed that it has been subjected to glacial transport and that it has had a long and complex history. It is also proposed that the abundant weathered and abraded bluestone boulders and slabs at Stonehenge were also glacially transported, along with many of the cobbles and stone fragments found in the sediments of the local landscape. The elaborate archaeological narrative of bluestone quarrying and human transport to Stonehenge must now be re-examined.
Über die Frage wie die sogenannten “Bluestones” von Ihren zahlreichen Quellen im Westen von Wales zu ihren heutigen Fundorten in und um Stonehenge transportiert wurden, gibt es ehebliche Differenzen. Seit einem Jahrhundert gehen die meisten Archäologen davon aus, dass die Steine von Menschen transportiert wurden, allerdings sind viele Geowissenschaftler der Meinung, dass die Steine mittels glazialen Transportes die “Salisbury-Plain” erreicht haben. Für beide Theorien gibt es bemerkenswert wenige Beweise. Deshalb ist jede neue Beschreibung eines möglichen glazialen Geschiebes, das am oder in der Nähe des Steinmonuments gefunden wurde, von potenziell großer Bedeutung. Ein kleiner, geschossartig geformter Block aus verschweißtem Tuffstein wurde 1942 bei einer Ausgrabung in Stonehenge gefunden und abgesehen von einer kurzen Untersuchung durch Geologen des UK Institute of Geological Sciences (IGS) um 1970, wurde er aus den Augen und aus dem Sinn eingelagert. Seine geologische Herkunft ist ungewiss. Nach einer eingehenden Untersuchung seiner Form und Oberflächenbeschaffenheit wird nun angenommen, dass er glazial transportiert wurde und eine lange und komplexe Geschichte hinter sich hat. Es wird weiterhin vorgeschlagen, dass die zahlreichen verwitterten und abradierten “Bluestone”-Blöcke und -Platten, ebenso wie viele der Feldsteine und Steinfragmente, die in den Sedimenten der Landschaft um Stonehenge auftreten, ebenfalls glazial transportiert wurden. Das ausgefeilte archäologische Narrativ des “Bluestone”-Abbaus und -Transports durch den Menschen nach Stonehenge muss nun neu untersucht werden. (Abstract was translated by Christopher Luethgens.)
-
Please read the editorial note first before accessing the article.
-
Article
(24162 KB)
-
Please read the editorial note first before accessing the article.
- Article
(24162 KB) - Full-text XML
- BibTeX
- EndNote
The Neolithic/Bronze Age megalithic monument of Stonehenge, on Salisbury Plain in southern England, is the most famous prehistoric structure in the British Isles. It is instantly recognisable, and there is an insatiable appetite for research “revelations” relating to its age, its purpose and the methods used in its construction. The small “bluestone” monoliths on the site (Fig. 1) have attracted attention ever since it was realised that they are geologically distinct from the larger sarsen stone monoliths which are found in the trilithon setting (with large pillars supporting lintels) and in the outer circle (Ramsey, 1858; Judd, 1903). They are however highly varied, and the term “bluestone” has no geologically consistent meaning. Studies of the petrography and geochemistry of the 43 remaining bluestones and of thousands of fragments in the “debitage” in the Stonehenge landscape have revealed multiple sources, mostly in West Wales (Thorpe et al., 1991; Ixer and Bevins, 2017; John, 2018a).
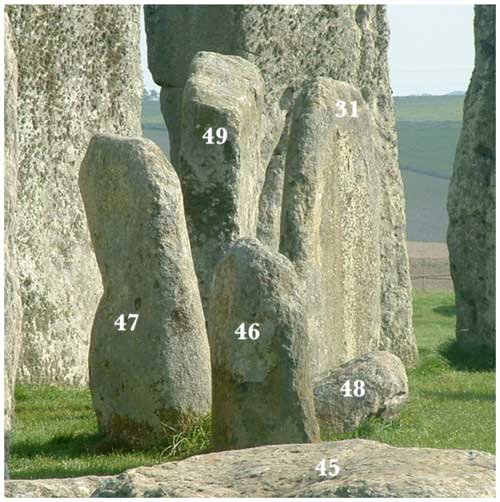
Figure 1Six of the Stonehenge bluestones belonging to the bluestone circle, in the NE quadrant of the stone monument. They are overlooked by the larger sarsens of the outer circle. For scale, stone 47 is 1.45 m tall. For the most part the bluestones are not elegant pillars but heavily abraded and weathered erratic boulders and slabs (photo: the author).
This lack of lithological conformity in the bluestone assemblage led Judd (1903) to conclude that they are probably derived from ancient and degraded glacial deposits on Salisbury Plain, and this interpretation was supported by Kellaway (1971), Briggs (1977) and then Thorpe et al. (1991) in the context of a detailed study by a team of geologists affiliated to the UK Open University. The present author (John, 2018a) pointed out that the bulk of the Stonehenge bluestone monoliths are not elegant and carefully selected pillars but highly abraded and weathered erratic boulders and slabs of many different rock types, probably collected from within the Stonehenge landscape (Field et al., 2015). He also supported the view that the stone settings at Stonehenge (involving both sarsen stones and bluestones) were frequently revised and never finished. He supported Stone (1924) in proposing that the stone monument was abandoned when the supply of accessible stones ran out.
This version of events is at odds with that proposed initially by Thomas (1923). He discovered that many of the sampled Stonehenge bluestones had close geological matches in some of the intrusive and extrusive rocks of the Fishguard Volcanic Group (FVG), which outcrops in Mynydd Preseli, Pembrokeshire, West Wales (Fig. 2). He postulated that they had come from a very limited geographical area. On the question of bluestone transport, he stated that because glacier ice had never (in his view) extended much further south than the South Pembrokeshire coast, this “permanently disposes of the idea of glacial transport for the foreign stones of Stonehenge”. He therefore proposed that the bluestones were first gathered together in West Wales and then transported over a distance in excess of 230 km by humans, and this version of events has subsequently been repeated by many others (Atkinson, 1979; Parker Pearson, 2012; Pitts, 2022). The human transport theory has recently been expanded to incorporate bluestone monolith quarrying at two supposed Neolithic stone quarries, at Craig Rhosyfelin and Carn Goedog, both on the northern flank of the Mynydd Preseli upland ridge in North Pembrokeshire. This speculation, which is the subject of intense debate, might explain how some foliated rhyolite and spotted dolerite monoliths were obtained, but it tells us nothing at all about how many other rock types were incorporated into the Stonehenge bluestone assemblage (John, 2018a).
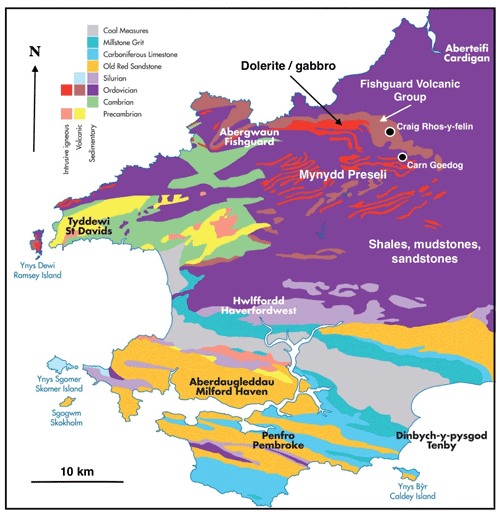
Figure 2Map of basic Pembrokeshire geology, showing the outcrops of the Fishguard Volcanic Group extrusive rocks and the strips of Ordovician intrusive rocks in the Mynydd Preseli area. Also marked are the two disputed “Neolithic quarrying” sites (source: modified from a Pembrokeshire Coast National Park base map).
In the last century it has been established that the ice of the Irish Sea Ice Stream (ISIS) has indeed affected the coasts of Somerset, Devon and Cornwall and has extended to the Celtic Sea shelf edge on at least one occasion (Clark et al., 2022; Scourse et al., 2019). Numerical ice sheet modelling has also demonstrated that the glacial transport of the bluestones all the way to Stonehenge was “not impossible” (Hubbard et al., 2009), since lateral spreading must have filled the Bristol Channel, the Severn Estuary and the Somerset Lowlands with glacier ice (John, 2018b).
Opponents of the glacial transport theory have argued that there is no bluestone erratic train between North Pembrokeshire (the entrainment area) and Salisbury Plain (the emplacement area) and that there are no known bluestone erratic boulders or glacial deposits on the Wiltshire chalk downs (Scourse, 1997; Parker Pearson, 2012). Further, following research on the river terrace gravels of the river Avon and its tributaries in the Salisbury area, Green (1973) reported that after examining 50 000 pebbles from 28 sites, he had not found a single pebble that could not have been derived from the local bedrock sequence. He therefore argued that there cannot have been a glacial incursion from the west or any other direction.
In the light of these considerable disagreements about the likelihood of glacial bluestone transport, it is clearly of some importance that all “foreign” clasts that might have been deposited in the neighbourhood of the stone monument should be carefully described and analysed.
It is frequently claimed that there are no known foreign stones on Salisbury Plain, apart from those at Stonehenge. This is not true. Fragments of many sizes and shapes have been found since the 1700s in chalk rubble and in other superficial deposits across Salisbury Plain (Thorpe et al., 1991; Ixer and Bevins, 2010). The clasts are generally referred to as “knock-offs” or debitage from the shaping of the Stonehenge bluestone monoliths or from the manufacture of stone axes, but some are discrete cobbles and larger clasts with abraded edges. Cleal et al. (1995, p. 383), in a classic and comprehensive study, mentioned bluestone “lumps and blocks” which “do not exhibit flaking characteristics”. They reported that 90 % of the bluestone clasts and fragments found by Col William Hawley in his Stonehenge excavations between 1919 and 1926 were thrown away on the grounds that they were not recognisable as artefacts and were therefore worthless. It is not known what happened to them, but it seems that some were thrown into Stonehenge “graves” along with many cremated bone fragments. When Aubrey Hole 7 (one of a series of holes outside the stone settings and near the eastern edge of the monument) was re-opened in 2008, the bones were deemed to be much more interesting than the stones (Willis et al., 2016). Clast rejection on such a scale has introduced an unconscious bias into all subsequent studies.
In 1924 an erratic rhyolitic boulder made of welded tuff or ignimbrite and measuring ca. 22 × 15 × 10 cm was found at Stonehenge in the course of one of the early excavations by Col William Hawley (Hawley, 1926). It is much smaller than any of the standing or fallen stones, and it has been referred to subsequently as “the Newall Boulder” (Fig. 3). Its find context is secure; it was one of four bluestone clasts found in the same dig, in the south-east quadrant of the stone monument. The “find zone” is now designated as C13, not far from Sarsen Stone 8, bluestone 34, and stumps 33f and 33e (Fig. 4). Two of the other discovered clasts were made of rhyolite and one of diorite. The archaeologists did not differentiate between clasts found in primary and secondary positions, and because the Newall Boulder appeared to have been worked or dressed, it was assumed to have been discovered in prehistoric times in one place, dumped in another, and then buried in the debitage and accumulating surface sediments.
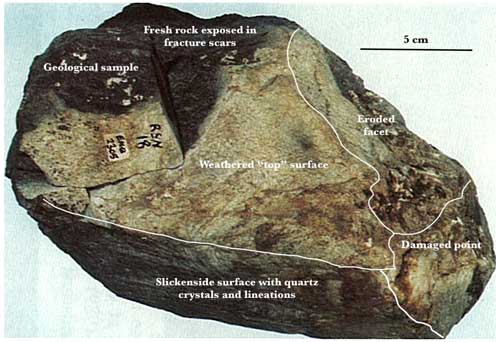
Figure 3A much-discussed photograph of the Newall Boulder, annotated by the author. The shape and surface features are widely interpreted as indicators of sub-glacial transport, in spite of heavy damage by humans (courtesy of the Institute of Geological Sciences/British Geological Survey).
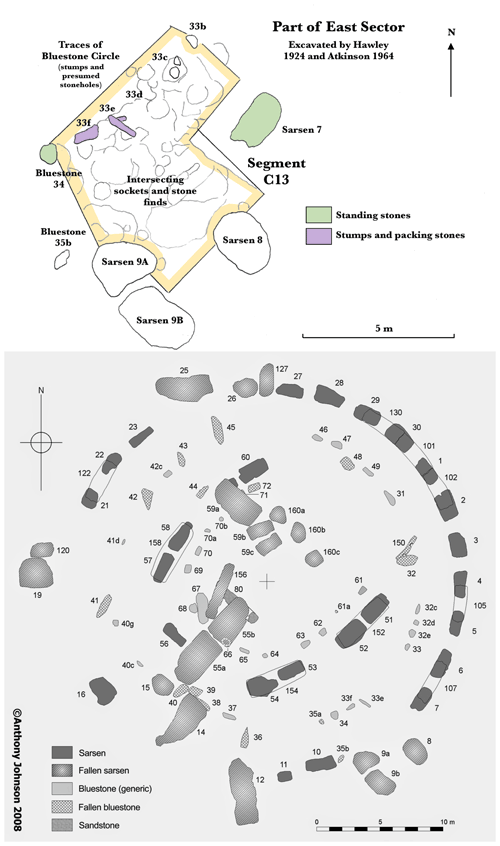
Figure 4Above extract from Fig. 120 (east sector plan) in Cleal et al. (1995), with annotations added. Below, plan of bluestone and sarsen locations at Stonehenge, courtesy of Anthony Johnson (2008). The Newall Boulder was found somewhere within the area outlined in yellow.
The ignimbrite boulder was “rescued” by Robert Newall (who was a part of Hawley's team between 1919 and 1926), taken home and stored in his attic, along with other clasts and bluestone samples. There it stayed for 46 years. In 1970, when Geoffrey Kellaway of the Institute of Geological Sciences (IGS) was preparing his article called “Glaciation and the stones of Stonehenge” (Kellaway, 1971), he discovered a reference to the boulder in one of Hawley's interim excavation reports and tracked it down to Newall's attic. Newall passed it over to him, and it was then subjected to intensive scientific scrutiny (and damage) by a team of petrologists including R.K. Harrison, R. Sanderson and B.R. Young. Some of their findings were later reported by Kellaway (1991), and some are in unpublished reports (Bevins et al., 2023). The boulder then went back into Newall's attic, but in 1976, two years before he died, it was passed for safe keeping to the curator of the Salisbury Museum, where it remained for a further 46 years.
It was deemed to have been lost, as mentioned by Thorpe et al. (1991), Scourse (1997) and John (2018a), but following the digitisation of Hawley's interim reports in the spring of 2022, blogger Tim Daw drew attention to the report of the 1924 dig, and following a speculative request to Adrian Green, the Director of Salisbury Museum, by the present author, the boulder was rediscovered. Following brief examination in June 2022, there is no doubt about its identity; it is the same boulder as that photographed by the IGS in 1972, and a cut rock sample numbered RNS18-ENQ2305 has also been retained (along with three others from the same boulder) in the extensive Salisbury collection of Stonehenge clasts and samples (Ixer et al., 2022; Bevins et al., 2023). The provenance is confirmed in correspondence between Kellaway, Newall and IGS geologists, which is now held in the Kellaway Archive of Bath University.
As reported by Kellaway (1991), geologist R.K. Harrison described the lithology of the boulder as follows:
This large, dark blue-grey, hard, flinty (? partly worked artifact) shows a white weathered crust up to 5 mm thick. The thin section shows a complex structure of very finely banded welded tuff (compressed foliated shards cemented by fine silica) with composite quartz grains and strings of dusty leucoxene, separated by patches of much finer grained, finely fluxioned glassy lava with patches of granular quartz. This specimen appears to represent a complex of originally viscous glassy lava and welded vitric tuff, all presumably of rhyolitic composition.
It is a coherent small boulder (Fig. 5) which is elongated, narrower at one end and with a wedge shaped cross section with dimensions ca. 22 × 15 × 10 cm. It was found at a depth of about 64 cm. It is approximately the same shape and size as an adult human face, and it is crudely bullet-shaped. Its original weight is estimated at ca. 5 kg. There are five major natural facets and several smaller ones, with further surface damage associated with fracture scars and geological sampling. One surface is undulating, one other is flat and another is curved. Some of the smaller facets appear to have been modified by abrasion, and others have a slight discolouration. The boulder has highly variable surface characteristics and patina. Grain size in the dark blue matrix is very small. There are many intersecting small fractures and veins marked by whitish crystal concentrations, as well as some lumps and patches of quartz crystals. There is one prominent yellowish nodule about 10 mm thick. One of the facets has a quartz crystal slickenside veneer, with remnants which seem to be streamlined and fractured (Bevins et al., 2023). There is also some iron staining on fresher fracture scars.
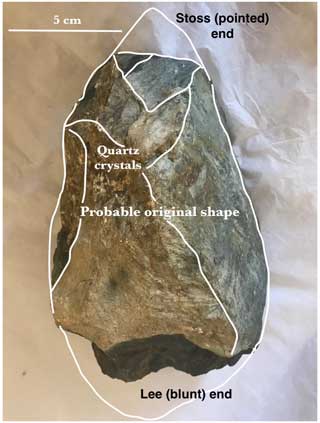
Figure 5The suggested original shape of the boulder, together with the natural facets, as they might have appeared prior to the infliction of damage by human beings (courtesy of Salisbury Museum).
There are many slight scratches on the boulder surface. There is one distinct patch of crossing scratches, ca. 5 cm × 5 cm on the flat facet. On another surface there are deeper non-parallel striations that have been damaged by weathering processes; these may be based on foliations or other lithological features within the rock (Bevins et al., 2023). Other marks are very shallow and do not appear to be geologically controlled.
A striking feature of the boulder is the contrast between the faceted and discoloured surfaces and the dark blue fracture scars particularly at its blunt end. Here the rock surface is very fresh, and edges are sharp. Some scars seem to have been present before the most recent phase of surface damage linked to geological sample collection. The two main fracture scars on the flanks of the boulder also seem to be older and are slightly stained. Some of the fresh fracture scars have been determined by vein positions.
The “top surface” of the clast is slightly convex, undulating and irregular. This surface, including the place from which the sample RSN18/ENQ2305 was taken, has a whitish colouring and a distinct weathering crust up to 5 mm thick (Fig. 6). The “flank facet” of the boulder is flatter and has a patina and quartz crystal veneer but no marked weathering crust. This is a crucial piece of evidence relating to the history of the boulder.
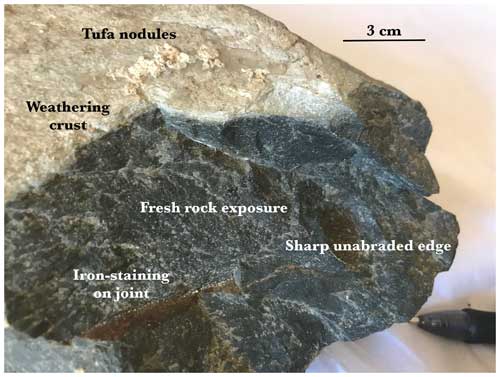
Figure 6Fresh “flinty” welded tuff exposed near the blunt end of the boulder. The damage has most likely arisen because of multiple blows with a hammer stone or other percussion tool (courtesy of Salisbury Museum)
In several parts of the boulder where there is no weathering crust, there is a tufa or sinter layer (calcium carbonate) up to ca. 4 mm thick (Kellaway, 1991). Some white crusty deposits of tufa occur on all faces and fracture scars, including the relatively fresh percussion fractures. But on these fractures there are simply a few small nodules.
The surface of the boulder has been extensively damaged by geologists. As demonstrated by Bevins et al. (2023), four substantial rock samples have been knocked or cut off the blunt end of the boulder by Geological Survey and Open University research teams, and the three samples that are still held in the collections can be fitted together with confidence. Another chunk from the blunt end was taken and pulverised for geochemical analysis. Yet another sample (numbered 36/1978) has been taken from the flank. Further damage can be seen on two fresh facets near the pointed end of the boulder. The clean and unweathered surfaces of these facets are either quite recent or else indicative of burial in a “protective” environment.
The dark blue flinty rock (Fig. 6) looks very different from the dolerites (spotted and unspotted), foliated rhyolites and ashes that make up the bulk of the Stonehenge bluestones, but it has similarities with some of the rhyolitic lavas and welded tuffs found in the Fishguard Volcanic Group in North Pembrokeshire. As suggested by Thomas (1923) and more recently by Ixer et al. (2022) and Bevins et al. (2023), the provenancing of the bulk of Stonehenge bluestone monoliths and debitage is now reasonably secure (Ixer et al., 2020), with the great majority of the examined clasts derived from Mynydd Preseli and the surrounding countryside. No exact provenances have yet been demonstrated satisfactorily, in spite of claims to the contrary. At the latest count, there are around 46 different lithologies represented in the “bluestone assemblage” at Stonehenge, almost all of them derived from the west (John, 2018a). Several attempts have been made to group these into a small number of rock type categories, but each attempt at simplification is followed by an admission of outliers and exceptions. Some Stonehenge monoliths and clasts (including the sandstone Altar Stone) have still not been subjected to modern physical sampling and are thus not securely provenanced.
Gowland (1903) reported “chippings of a hard compact rock” unlike any of the known bluestone monoliths. Was this a reference to detritus related to the Newall Boulder? Harrison and his colleagues suggested that the boulder had most likely come from near Capel Curig in North Wales, but that may be because they were more familiar with North Wales intrusions and volcanics than with similar rocks in Pembrokeshire. Peter Kokelaar of Liverpool University suggests (personal communication, 2022) that the boulder might have come from Ramsey Island or one of the minor rhyolite sheets near the North Pembrokeshire coast.
The available samples from the boulder have been examined by Bevins et al. (2023), with a view to provenancing. They state the following: “We have recently reunited and examined the joint block and all its offcuts and associated thin sections, and the rhyolitic tuff shows all the key characteristics needed to assign it to Rhyolite Group C from Craig Rhos-y-Felin.” This is a crag (Fig. 2) in a deep valley near the village of Brynberian. They provide no evidence in support of this claim, and indeed Harrison's description (see above) suggests substantial physical differences between this dark blue boulder and the light blue and fine-grained rock exposures at the named outcrop.
Ixer et al. (2022) refer to the Newall Boulder as a “broken joint block”. They assert the following:
analysis by pXRF on all pieces of the joint block plus two other visually similar rhyolitic tuffs from the same Newall collection (RSN9/ENQ 2295 and RSN10/ENQ 2296) clearly show that these fragments are compositionally Rhyolite Group C, confirming the petrographic identifications.
The geochemical plots of ppm of selected minerals (Ba, Zr, Nb and Rb) on A and B axes, for a large number of “Craig Rhos-y-felin” analyses compared with analyses for three Newall samples numbered RSN18, RSN9 and RSN10, are cited in support of this assertion. However, it is not clear whether the Rhos-y-felin X-ray analyses were all conducted on rock faces at Rhos-y-felin or whether some (or even most) of the analyses were conducted on samples found at Stonehenge but deemed to have come from Rhos-y-felin. The “field” covered by the Rhos-y-felin samples is very large, and plots from many other rhyolites and dolerites from diverse sources would fall within it if they were to be added. The bivariate plot of Ca ppm against K ppm shows virtually no coincidence or overlap between RSN18 samples and Craig Rhos-y-felin samples (Bevins et al., 2023). The fact that the petrography and geochemistry of the RSN18 samples are “consistent” with rocks classified as “Rhyolite Group C” does not mean that the boulder must have come from Craig Rhos-y-felin. Thus their Fig. 7 tells us nothing about the precise source of the Newall Boulder.
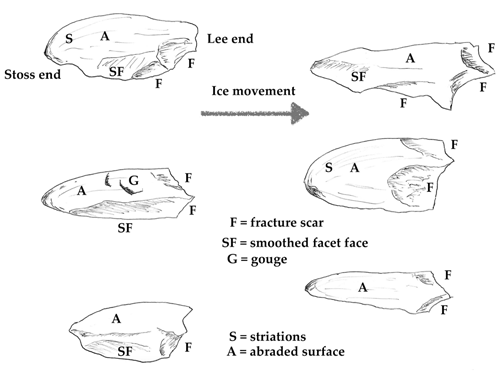
Figure 7Some of the features of bullet-shaped clasts thought to have been fashioned in a subglacial environment. There are multiple variations on these themes, with intersecting fractures, rough scars which turn into polished facets, gouges in more or less random positions, and crossing striations. There will also be modifications related to the lithology and internal structure of the clast, foliations, ancient fractures, presence of quartz veins etc. (based on clasts and photos in the author's field collection).
A highly magnified image published by Ixer et al. (2022) as their Fig. 5 shows the mineral stilpnomelane, labelled as follows: “This image highlights the pale brown wispy stilpnomelane, which is a defining characteristic of rhyolites from Craig Rhos-y-felin.” However, no provenance is attached to the image, so its significance is difficult to assess. Stilpnomelane also occurs elsewhere in the Fishguard Volcanic Group outcrops and in other areas of low-grade metamorphism, and it cannot therefore be used as a precise provenance indicator (Howells, 2007). The best that can be said about provenance is that the boulder has possibly come from one of the FVG outcrops in the Brynberian–Pont Saeson area of North Pembrokeshire.
Bevins et al. (2023) suggest that the boulder is the broken-off top of a foliated rhyolite standing stone, and they compare its shape with the tips of bedrock pillars at Craig Rhos-y-felin (Parker Pearson et al., 2015). However, if it is indeed the broken-off tip of an elongated monolith, the latter must have been so tall and slim that it could not possibly have been extracted intact from a quarry face or transported intact from West Wales to Stonehenge. The “parent monolith” would have had b-axis and c-axis dimensions of ca. 10 and ca. 15 cm – making it far more fragile than any of the 43 known bluestones at Stonehenge. In any case, the breakage of the top of a monolith (whether by natural processes or human agency) would have left one clean fracture scar, probably iron-stained. Instead, there are at least three intersecting fresh fracture scars on the blunt end of the boulder, two near the pointed tip, and another three on the flanks (Fig. 5). As appreciated by both Newall and Kellaway, damage on this scale is a sure sign of human activity, probably in the prehistoric period.
Further, there are no known monoliths of this rock type in the stone settings. It is speculated by Bevins et al. (2023) that the stump of stone 32d (less than 10 m from the find location) might be all that is left of a destroyed foliated rhyolite monolith, but no sound evidence for that claim has ever been published. The stump was excavated by Richard John Copland Atkinson in 1954, and in photographs it looks like a fractured and flaky rhyolite, but it is recorded confidently by Cleal et al. (1995, p. 246) as a “spotted dolerite”. So any link between the Newall Boulder and stump 32d is at present entirely speculative.
The rhyolite exposed in the rock face at Craig Rhos-y-felin is so fractured and brittle that during the Neolithic and the Bronze Age it was never valued in West Wales as a raw material for megalithic structures (John et al., 2015a). It is therefore highly unlikely that there would have been any motivation for the removal of “rubbish” monoliths for use in either local or distant stone settings (Thorpe et al., 1991).
In seeking to understand how the Newall Boulder might have been transported from its original place of origin, a number of key features need to be explained:
- 1.
a crude bullet shape, with a pointed nose and a blunt back end
- 2.
at least five major facets and several smaller ones
- 3.
abraded surfaces and edges
- 4.
fracture scars on the flanks and especially at the lee or blunt end
- 5.
apparent streamlining prominent on one facet
- 6.
faint crossing scratches on one facet and weathered parallel scratches on another
- 7.
minor crescentic gouges and microfeatures (chip marks or chatter marks) attributed to pressure exerted at particular points.
On the basis of its shape the Newall Boulder is classified on the Powers six-category scale as sub-angular (Powers, 1953). In general, clasts in periglacial slope deposits and rockfall fragments tend to be sharp-edged or angular, simply because they have not travelled far. This boulder is clearly not simply a broken piece of rockfall debris. In analyses of glacially transported clasts the majority fall in the sub-angular and sub-rounded categories. Glaciofluvial sands and gravels contain more rounded and sub-rounded stones and fewer sub-angular ones (Lukas et al., 2013; Evans, 2018; Benn and Lukas, 2021). Fluvially transported clasts contain more rounded clasts, and clasts on wave-washed beaches tend to be well rounded. The boulder is therefore unlikely to have come from river gravels or from a wave-washed shoreline.
The simplest explanation of the bullet shape is that the boulder has been shaped while taking a position of least resistance in a flowing medium, with erosive forces concentrated on the pointed (upstream) end and reduced at the blunt (downstream) end. When shown a simple photograph of the boulder (Fig. 3), without any contextual information, 11 out of 12 senior geomorphologists agreed that the boulder has probably been transported sub-glacially. The other colleague suggested a glaciofluvial origin. However, close examination reveals that there are many fresh microsurface features which would have been removed if there had been prolonged water transport or modification.
Clasts occupy a wide range of positions in mobile subglacial till (Evans et al., 2016, 2018). They are subject to complex transport histories that involve variable amounts of dragging, rolling and lodging, during which they are subject to surface modification through inter-clast collisions and contacts. Any single clast may be reworked numerous times during successive glaciations. Because clasts will tend to take the line of least resistance to the flow of the surrounding deforming till matrix, facetted and bullet or wedge shapes are developed. Whenever a clast is disrupted from its lodged position, it can be subject to fresh fracturing, gradually changing its overall shape to one of a block (Boulton, 1978; Benn and Evans, 1996; Evans, 2018). Although not all glacially transported clasts display such bullet or flat-iron shapes, such an appearance is diagnostic of significant subglacial transport (Evans, 2018; Evans et al., 2006) (Fig. 7).
The characteristics of the Newall Boulder suggest a subglacial transport history. For example, the top of the boulder is abraded and yet rough, with a number of surface projections and grooves related to lithology. Another large facet is convex or curved, dominated by sheets and strips of microcrystalline quartz with slickenside alignments roughly parallel with the boulder's long axis. One small facet near the boulder tip is concave. Two small meeting facets near the boulder tip are remarkably clean, with a “polished” appearance, and another near the tip has a protruding quartz mass that has somehow survived abrasion. On the edge of a facet near the blunt end of the boulder, there are two pronounced concave fracture scars. Overall, the surface characteristics of this boulder suggest that it is a discrete erratic that has been transported for much if not all of the time in a subglacial position (Benn and Ballantyne, 1994; Lukas et al., 2013; Benn and Lukas, 2021).
The apparent streamlining on a patchy crystalline quartz deposit on one large curved facet is not thought to be evidence of glacial transport. This is also pointed out by Ixer et al. (2022) and Bevins et al. (2023). Instead, it is interpreted as the result of slickensiding on a fault plane (Fig. 8). Peter Kokelaar suggests (personal communication, 2022) that there are several diagnostic features, including quartz-mineral ribbon/fibre growths, growth increments, and ribbons with stepped ends showing where missing counterparts have broken away. The protruding yellowish quartz mass near the boulder tip appears to be associated with a second, smaller fault plane. The presence of two intersecting faulted surfaces in the original rock outcrop could have facilitated either breakage under a periglacial climatic regime or glacial entrainment of the boulder at a time of thick ice cover.
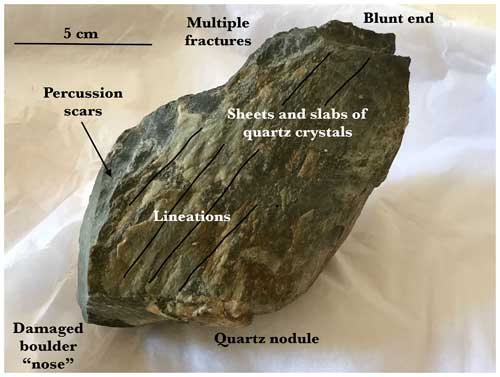
Figure 8Faulted surface displaying slickenside features. These include sheets and slabs of microcrystalline quartz and prominent lineations. The marked quartz nodule appears to have formed on a smaller intersecting faulted surface (courtesy of Salisbury Museum).
Bevins et al. (2023) argue that because slickenside features are present on the Newall Boulder and also on foliated rhyolite surfaces at Craig Rhos-y-felin, this suggests a source for the boulder. However, slickenside features including slickencrysts are common across West Wales, in all faulted lithologies and of all ages. None of the surface features of this boulder demonstrates a link with Craig Rhos-y-felin.
The presence of genuine glacial striations on the surface of the boulder is a matter of dispute. It is appreciated that the smaller striations on the boulder surface are so faint that some will doubt their significance as glacial diagnostic features. However, both Engleheart and Dale said in 1921 that they had seen striated glacial erratics at Stonehenge (Hawley, 1921). Bevins et al. (2023) suggest that Kellaway thought the streamlined/slickensided features were glacial striations, but in the view of the present author he must have been recording some very subtle but still discernible features that are unrelated either to the internal structure of the rock or to slickensiding (Fig. 9).
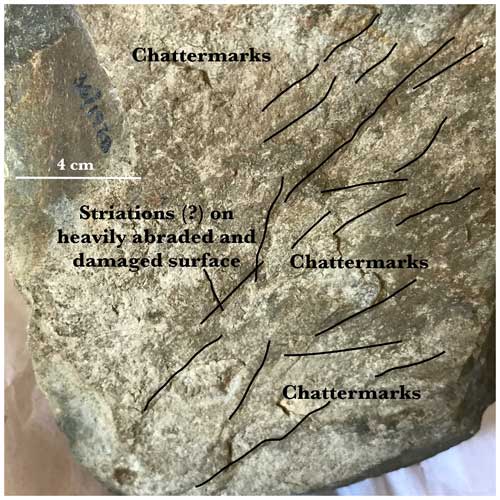
Figure 9Possible traces of glacial erosion on the rough and weathered face of the boulder (courtesy of Salisbury Museum).
When discussing the possible impacts of glacial processes on the Newall Boulder, Bevins et al. (2023) claim that striae typically cover abraded surfaces. However, the great majority of glacially transported clasts do not carry any striae at all. Striae are not typically dense or continuous over a large proportion of a facet surface. In short, most striated clasts are not particularly spectacular (cf. Sharp, 1982; Kruger, 1984; Benn and Evans, 2010; Evans et al., 2016, 2018).
The weathering crust on the top of the boulder, ca. 5 mm thick, is of considerable importance (Fig. 10). Kellaway (1991) suggested that it might have been created prior to glacial transport. This is unlikely; although it is known that some glacial erratics carry inherited cosmogenic exposure ages for example, it would be rare for a heavily abraded and faceted erratic boulder to display “pre-glacial” weathering traces, since those would be the first to be removed during transport. This dilemma can be resolved by cosmogenic dating. Bevins et al. (2023) have conducted valuable work on the weathered surface of the Newall Boulder and on other rock surfaces, using pXRF technology, but only with a view to establishing geological relationships and provenancing. They have not commented on the “exposure ages” of the surfaces investigated. The fact that the weathering crust only exists on part of the boulder argues for the operation of weathering processes on an exposed surface after its emplacement, while the rest of the boulder was buried and thus protected.
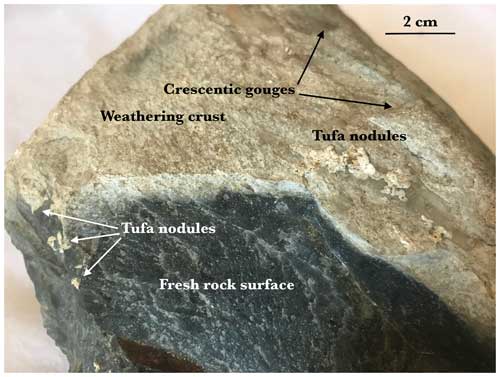
Figure 10Features associated with different phases in the boulder's history. The crescentic gouges are suggestive of subglacial transport. The weathering crust and larger tufa nodules suggest long-continued exposure in a calcium-rich environment. The fresh rock surfaces suggest fracturing as a result of repeated percussion in prehistoric (?) time. The smaller tufa nodules suggest renewed precipitation of calcium carbonate following Neolithic/Bronze Age reburial at Stonehenge (courtesy of Salisbury Museum).
An old “ground surface” position is revealed by a change in surface colouration; the whitish boulder top was clearly exposed to weathering/cosmic bombardment over a long period, while the rest (coloured dark blue or black) was buried. There may also have been effects associated with a vegetation cover and humic acid penetration. In several places beneath “ground level” there are crusty tufa or sinter deposits up to ca. 4 mm thick (Fig. 9). This suggests a calcium-rich environment, probably on Salisbury Plain. Some white crusty deposits of tufa occur on all faces and on some percussion fracture scars of probable human origin, and this suggests a second phase of tufa precipitation while the boulder was completely buried.
The interpretation of the boulder is made more intriguing because of the substantial prehistoric human damage that it has suffered (Fig. 11). This damage has been recognised ever since it was found in 1924. Detailed investigation suggests the following narrative. It is a rejected “artefact” in the sense that somebody has tried at some stage (Neolithic?) to use it either as a maul (hammering tool) or as the raw material for a large stone axe (Fig. 5). The axe-making attempt was not successful, probably because the rock does not fracture conchoidally, and when several chunks had been knocked off the pointed end, the blunt end and the edges, the attempt was abandoned and the stone was thrown away and buried under accumulating debris associated with the Stonehenge stone settings. It has also been damaged within the last 60 years in several different places by the geologists who have examined it; as mentioned above, there are traces of cutting and grinding in perhaps four different places, and there are several new percussion scars in a row near the pointed end of the clast (Fig. 12). There is one flat, smooth face which looks as if it has been ground down in the collection of a rock powder sample. The fact that tufa nodules are found on some percussion fractures suggests further slight precipitation of calcium carbonate following the rejection by the Neolithic (?) axe maker and subsequent burial in chalk rubble. The boulder remained in this position until it was uncovered by Hawley's team in 1924.
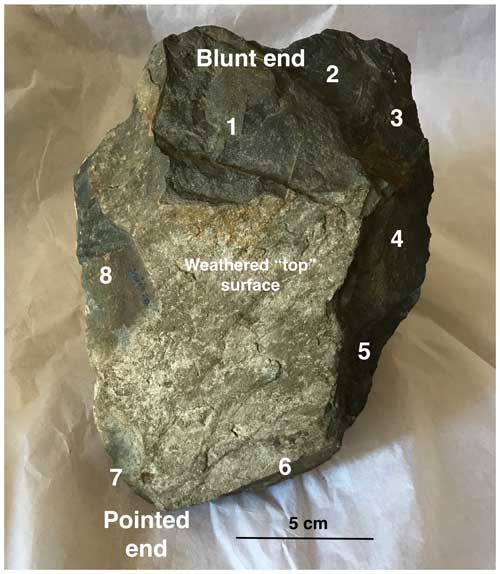
Figure 11Likely human damage to the top and the flanks of the boulder. Areas 1 and 8 (and possibly 6) have also been affected by geological sampling since Kellaway initiated research investigations (courtesy of Salisbury Museum).
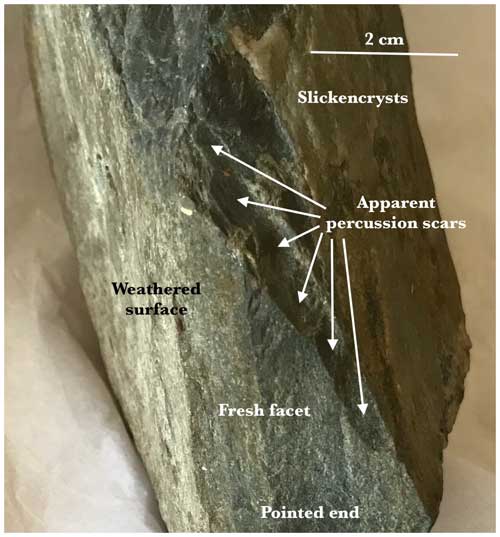
Figure 12A row of small percussion (?) scars near the damaged pointed tip of the boulder (courtesy of Salisbury Museum).
Is there a possibility that this stone could have been transported to Stonehenge from far away by the people who built the monument or by visiting traders? This was the response of Kellaway (1991) in answer to that question:
When found, the weathered boulder had been thrown away with chippings and other waste material. An attempt had been made to dress one end of the boulder but this, in Mr Newall's opinion, had failed because of the sheared condition of the rock. It would appear that this small boulder, already deeply weathered, would never have been of any practical value. To suggest it had been carried from North Wales to Wiltshire only to be tested and thrown away as worthless would imply an astounding lack of common sense and understanding of the properties of rocks on the part of the men who built Stonehenge. If, however, the bluestones were recovered locally from material scattered on the surface of the Chalk or were present in solution cavities, then the presence of inferior material is comprehensible. Having gathered up all the available bluestones, both from natural sources and from abandoned Neolithic structures, the Bronze Age builders of Stonehenge used the large ones for constructional purposes and tested the smaller boulders for the manufacture of implements. Those which were unsuitable were thrown away.
Kellaway's comments appear to be well founded, and this author concurs with most of them. The boulder's irregular faceted shape and varied surface features must have rendered it almost impossible from the outset to fashion into an axe, and this suggests strongly that it was found locally. The attempted dressing of the stone was crude and apparently somewhat half-hearted, and this indicates that it never was a particularly desirable or special object. It happened to be there, so it was used.
The tufa deposits, together with the above characteristics, indicate that this boulder was not freshly quarried at Rhos-y-felin and carried by humans to Stonehenge. As initially observed by Kellaway, it is unclear why anybody would gather up a small stone of this size and carry it all the way to Stonehenge only to knock a few bits off and then discard it.
In summary, this boulder has undergone a complex transport history of several distinct phases:
- 1.
Following entrainment the boulder was reduced in size and heavily modified during glacial transport, for much of the time on the bed of a glacier. It was eventually dumped at some location on, or relatively close to, Salisbury Plain.
- 2.
The top of the boulder projected above the ground surface and was subjected to subaerial weathering over a protracted period. In this calcium-rich environment a crust of tufa was deposited on some of the buried surfaces of the boulder.
- 3.
It was dug up and collected by an axe-maker on the basis that it was roughly the right shape and size for a large hand axe. He tried to shape it by percussion, particularly on the blunt end, possibly taking advantage of existing fracture scars acquired during glacial transport. Some attempts were also made to shape the pointed or stoss end. The enterprise failed because the stone did not fracture conchoidally or predictably.
- 4.
The stone was thrown away into a pile of accumulating chalk rubble in connection with the Neolithic erection of standing stones at Stonehenge. It was gradually buried to a depth of 64 cm, and over time further nodules and crusts of tufa were formed on all surfaces, including the “fresh” human-made surfaces.
- 5.
Following its discovery (in 1924) by Hawley and his co-workers, Hawley treated it as “rubbish” and wanted to discard it, but Newall took it home together with other foreign stones and put them in his attic.
“Although it still has vocal supporters, eminent geologists and glaciologists have dismissed the glacial theory” (Bowen, 2005; Green, 1997; Scourse, 1997) and concur with Thomas's original suggestion that the stones “were transported by human agency, in all probability by an overland route” (Thomas, 1923).
This statement from Darvill and Wainwright (2016) is mirrored in scores of other publications and is too easily accepted as confirmation that a consensus exists. However, it is notable that many specialists in the field of glacial geomorphology have pointed to conditions suitable for the long-distance transport of monoliths and other glacial debris from west to east, on at least one occasion (Gilbertson and Hawkins, 1978; Thorpe et al., 1991; Green, 1992; Bowen, 2005; Hubbard et al., 2009; Patton et al., 2017; Gibbard et al., 2017). If there is a specialist consensus in the earth sciences field, it is in favour of the glacial transport hypothesis.
As noted above, in spite of the wide belief that erratic clasts are entirely absent from the chalk downs and Salisbury Plain, there is abundant evidence of finds in the literature (Thorpe et al., 1991; John, 2018a). It is true that no coherent glacial deposits or morainic landforms have been described, but most of the land surface on Salisbury Plain has never been investigated, and research within the military training area is not encouraged. It is also true that no erratic train has ever been traced between Pembrokeshire and Salisbury Plain, but those who repeatedly highlight this fact forget that a large percentage of the route that might have been followed by the ice is now submerged beneath the waters of the Bristol Channel and the thick organic sediments of the Somerset Levels (Gibbard et al., 2017).
The debate continues about the significance of the spotted dolerite boulder found in a Neolithic context in Boles Barrow, near Heytesbury, the lumps of rotten granite found near West Kennet and the dolerite debris found near the village of Lake. There is also speculation about an abraded rhyolite cobble found near Durrington (Fig. 13) and about bluestone clasts found in the long barrow numbered Amesbury 39. Bluestone clasts have been found near the summit of Silbury Hill, and finds from the Cursus and Fargo Wood have been identified as “acid volcanics and tuffs” and also spotted dolerite. Some finds were identified as calcareous ashes. In 2008 a further “bluestone” from the fill of a Cursus pit was identified as identical to one of the sandstone stumps in the Stonehenge bluestone circle. At least 20 “bluestones” have been listed by the Wessex Archaeological Trust in the Stonehenge environs but outside the monument itself. According to Thorpe et al. (1991),
Bluestone fragments are frequently reported on and near Salisbury Plain in archaeological literature, and include a wide range of rock types from monuments of widely differing types and dates, and pieces not directly associated with archaeological structures
In much of the current literature these finds are dismissed as fragments of destroyed Stonehenge bluestones that have found their way into other contexts. But this fails to explain why some finds seem to have been in position prior to the assumed “bluestone arrival date” of ca. 5000 years BP (Cleal et al., 1995).
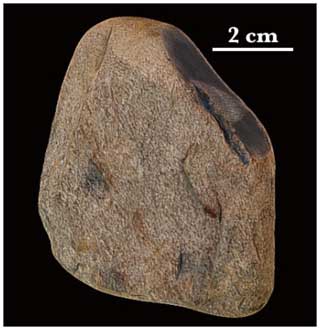
Figure 13The Durrington rhyolite cobble, minus a sample taken by the geologists. It has a weathered and abraded surface and bears signs of subglacial pressure fractures. It is highly unlikely to be a “knock-off” from a destroyed Stonehenge bluestone monolith or a cultural “object” (reproduced with permission, © Wessex Archaeology).
As Thorpe et al. (1991) pointed out,
The monoliths at Stonehenge include some structurally poor rock types, now completely eroded above ground. We conclude that the builders of the bluestone structures at Stonehenge utilized a heterogeneous deposit of glacial boulders readily available on Salisbury Plain.
It should be noted that most of the 43 bluestone “monoliths” at Stonehenge are not elongated elegant pillars (as portrayed in most reconstructions) but heavily abraded unremarkable boulders and elongated slabs. There are clearly defined facets, some of which are rough and others smooth. There are few sharp edges. The stones would not be out of place in the morainic accumulations around any glacier snout in the world (Benn and Evans, 2010, and references therein). They look like glacial erratics, and they are heavily weathered as a result of prolonged exposure (Fig. 14). On some weathered surfaces segments of the crust have peeled away and have been lost. It is probable that Stonehenge was built where the stones were found, as suggested by Judd (1903) and Field et al. (2015), and this is supported here by the preliminary analysis of the Newall Boulder.
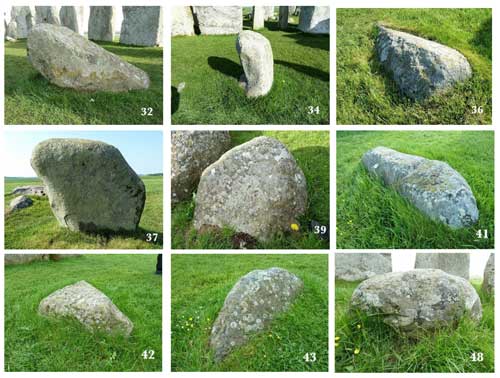
Figure 14Some of the Stonehenge boulders found in the Bluestone Circle. All have rounded edges and facets, some rough and some smooth. These features in combination are suggestive of ice transport. These are mostly made of dolerite, which seldom supports striations. Note also the weathering crusts, which in places have peeled off. For the locations of these monoliths, please refer to Fig. 4 (courtesy of Simon Banton and the Stones of Stonehenge website: http://www.stonesofstonehenge.org.uk/, last access: 27 May 2024).
On the matter of clast morphology in the debitage, Ixer and Bevins (2010, 2011a, b, 2013) have consistently failed to differentiate between sharp-edged fragments, cobbles and larger clasts and have concentrated on material that might be related to existing or destroyed bluestone monoliths. So-called hammer stones, mauls and packing stones have not received adequate attention, although some of them are made of igneous rock and look like glacial clasts (Cleal et al., 1995).
Green's evidence relating to Salisbury Plain river gravels is at odds with that presented above. He claims that there is a “complete lack of glacially derived material in the Pleistocene river gravels of the Wylye, Nadder and Avon” (Green, 1973, p. 216). He did find “far travelled pebbles” in the river gravels but expresses the view that they came from post-Cretaceous rocks which once capped the chalk and which have subsequently been removed by erosion. He appears to have used vein quartz pebbles as a proxy for “far travelled material”. In examining ca. 50 000 pebbles he classifies the great majority of them (in excess of 95 %) as “flint and greensand”. While it is accepted that the river gravels of the Thames Valley contain much more abundant glacially transported erratics, Green does not adequately demonstrate a “complete lack” of glacial detritus on the chalk downs of Salisbury Plain. Upper greensand pebbles might themselves have been glacially transported from Upper Greensand outcrops to the west.
The multiple arguments assembled by Scourse (1997) against glacial impacts in the Stonehenge landscape are even more problematical, although most have been overtaken by events. In the last sentence of his chapter, James Scourse claims to have “eliminated the impossible” from the debate about bluestone transport, thereby demonstrating that the human transport of the stones – however improbable – actually happened. It is unusual for a scientist to use the word “impossible”. James Scourse claims that if Salisbury Plain had been glaciated, there should be glacial sediment sequences and “depositional landforms”. This claim cannot be sustained, since “unglaciated” or “drift-free” terrain in all glaciated areas has been shown, over and again, after detailed investigations, to have been affected by ice (Clapperton and Sugden, 1975; Håkansson et al., 2009; Evans, 2016; John, 2023). He claims that if the chalk scarp had been overridden by ice from the west, there would be “glacio-tectonic structures”. Such glacitectonic disturbance is indeed common in glaciated soft bedrock terrains (e.g. Vaughan et al., 2011) but is dictated by optimum localised conditions and may only be detected if suitably large exposures are available. Such conditions were satisfied in some of the chalk areas of eastern England. He claims that glaciological theory makes it impossible for glacier ice to have carried erratics from Preseli to Stonehenge. That is disproved simply by reference to the large far-travelled erratics found in Somerset, Devon and Cornwall, some of which are in excess of 80 m above sea level (Madgett and Inglis, 1987). Spectacular igneous erratics are still being found on Bristol Channel coasts; indeed a large dolerite boulder was found on the shoreline in Limeslade Bay (Gower Peninsula) as recently as 2022. James Scourse says there is an inconsistency between “observational and theoretical data... and the regional geological data”. That is a matter of opinion, and as noted above glacier modelling has repeatedly shown that Salisbury Plain could well have been located within the “glacial footprint” during some Quaternary glacial episodes (Patton et al., 2017, 2022) (Fig. 15).
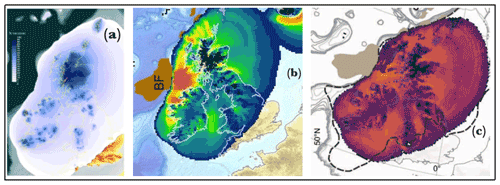
Figure 15Three “extreme” models in which attempts are made to define the extent and other characteristics of the LGM British–Irish Ice Sheet. (a) Hubbard et al. (2009), an outlier model run showing the percentage of time during which the land surface was ice covered. (b) Patton et al. (2016), showing potential LGM erosion. (c) Patton et al. (2022), showing rates of erosion during the Late Devensian glacial episode. These models are all constrained by unreliable information about ice extent in the Celtic Sea arena. If the ice edge is pushed south-westwards to the shelf edge in line with “ground truthing”, then it must also be pushed south-eastwards in southern England. The new limit would incorporate local ice caps over the uplands of SW England.
It is widely accepted that at some stage during the Quaternary glacier ice travelling from the west affected the coastal zone near Bristol, the Mendips and Bathampton Down near Bath (Hunt, 1998; Gilbertson and Hawkins, 1978). This means that the ice surface must have been at least 250 m above present sea level. It follows that in accordance with the laws of ice physics and the tendency of flowing ice to spread laterally into depressions, the extensive Somerset Lowlands must have been glaciated. Indeed, glacial deposits are recorded at Greylake (south-east of Bridgwater) beneath approximately 7 m of interglacial deposits, and at nearby Westonzoyland there was a giant erratic (now destroyed) known as the Devil's Upping Stock.
With regard to the timing of the glacial episode responsible for the transport of the Stonehenge bluestones, the relative scarcity of field evidence suggests that a prolonged period of landform degradation and sediment redistribution has occurred since the last glacial ice on or near Salisbury Plain melted away. The glaciation is unlikely therefore to have been that of the Last Glacial Maximum (LGM), which culminated around 25 000 years ago. The depositional features, till and related deposits from that episode are still relatively fresh, and the extent of that glaciation in the Celtic Sea arena and in SW England is now relatively well understood (Scourse et al., 2022). The LGM Irish Sea Ice Stream is thought to have pushed into the Bristol Channel and to have affected the coasts of Devon and Cornwall, but it is unlikely to have reached Somerset or Wiltshire. Very little is known about the MIS10–16 glacial episode in the western part of the British Isles, so the most likely candidate for bluestone transport is the Irish Sea Ice Stream of the Anglian glacial episode (MIS12), around 450 000 years ago (Fig. 16).
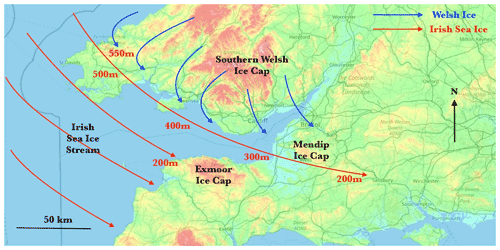
Figure 16Suggested ice flow patterns for the southern parts of the Irish Sea Ice Stream and the Welsh Ice Cap during the “Greatest British Glaciation” (?Anglian). Approximate ice surface altitudes are added. The ice edge maximum position for this episode is still to be delineated.
The Anglian Glaciation (ca. 0.43 Ma; MIS 12) marks the biggest Quaternary expansion of the BIS and had a marked effect on the landscape of Britain. It radically altered drainage and relief, and initiated the formation of the Straits of Dover which led Britain to be isolated from mainland Europe during various high sea-level stands during the Middle and Late Pleistocene. The chronology of Middle Pleistocene expansions of the BIS is still contentious, with conflicts existing between different types of evidence, and in some cases, different interpretations of the same evidence (Lee et al., 2011).
In the view of the present author there are cemented till deposits probably dating from the Anglian Glaciation in a number of West Wales localities, and elsewhere concentrations of sub-rounded and weathered erratic boulders appear to be older than the raised beach deposits attributed to the Last Interglacial (Ipswichian). The glacial deposits of Somerset are also likely to be of Anglian age (Green, 1992), as are some of the tills and terrace gravels of the Upper Thames basin. The correlation of deposits in the South Midlands (including the Upper Thames drainage basin) presents a formidable challenge (Gibson et al., 2022; Gibbard et al., 2022), and it now appears that the Wolstonian Glaciation might, in some sectors, have been more extensive than the Anglian. Much work remains to be done on the timing, extent and characteristics of the British–Irish Ice Sheet and its associated ice streams prior to the last (Ipswichian) interglacial (Lee et al., 2004).
With regard to ancient glacial episodes, the Newall Boulder has an intriguing association with the “Ardleigh Boulder” found in river gravels near Colchester in Essex in 2009 (Rose et al., 2010). The two boulders have clear morphological similarities. They are approximately the same size; both are roughly bullet-shaped, both are made of rhyolite, and both appear to have come from Wales. Both are faceted and have abraded edges, and both have one facet that appears to support striations. Rose et al. (2010) have suggested that the Ardleigh Boulder, more than 200 km from its probable source, was transported fluvially in or on floe ice. And it was also suggested that the Ardleigh Boulder came originally from ancient glacial deposits, possibly in the Welsh Borders. The difference between the two boulders is that the Ardleigh Boulder was entirely naturally emplaced, whereas the human damage to the Newall Boulder, following its initial discovery, is all too obvious.
In emphasising the possibility that the Stonehenge bluestone monoliths and other fragments in the debitage were transported by Anglian ice, and in accepting that firm evidence on the ground is hard to come by, as pointed out by Scourse (1997), it should be emphasised that evidence in support of the alternative theory – of human transport – is even more difficult to assemble.
- 1.
There is no sound evidence from anywhere in the British Neolithic/Bronze Age record of large stones being hauled over long distances for incorporation in a megalithic monument (Thorpe et al., 1991).
- 2.
Field observations show, consistently, that the builders of Neolithic monuments across the UK simply used whatever large stones were at hand (Burrow, 2006).
- 3.
If special or sacred stones were being transported to Stonehenge, it is vanishingly unlikely that they would all have been collected in the west, to the exclusion of all other points of the compass (John, 2018a).
- 4.
There is no evidence either from West Wales or from anywhere else of bluestones (for example foliated rhyolite or spotted dolerite) being used preferentially in megalithic monuments or revered in any way (Darvill and Wainwright, 2016).
- 5.
If long-distance stone haulage was an “organised activity” for the builders of Stonehenge, it is noteworthy that there is no evidence of the development of an appropriate haulage technology leading up to the Late Neolithic and a decline afterwards. In other words, there is no sign of any diffusion of innovation (Rogers, 2003).
- 6.
The evidence for quarrying activity in key Preseli locations is questionable (John et al., 2015b). No archaeological or cultural links have been established between Stonehenge and the proposed “quarries” at Craig Rhos-y-felin and Carn Goedog.
- 7.
The sheer variety of bluestone types argues against human selection and transport. There cannot possibly have been multiple “bluestone monolith quarries” scattered across West Wales (Thorpe et al., 1991).
- 8.
No physical evidence has ever been found of ropes, rollers, trackways, sledges, abandoned stones, quarry worker camps or anything else that might bolster the hypothesis (Kellaway, 1971).
- 9.
Experimental archaeology on stone haulage techniques (normally in “ideal” conditions) has done nothing to show that our ancestors could cope with the sheer physical difficulty of stone haulage across the heavily wooded Neolithic terrain of West Wales (characterised by bogs, cataracts, steep slopes and very few clearings) or around the rocky coast. Burl (2007) made this point forcefully, and it remains forceful today.
- 10.
No convincing evidence has ever been found of a “proto-Stonehenge” in West Wales, built of assorted local stones that were dismantled and taken off to Stonehenge. Mike Parker Pearson's claim that a “giant stone circle” at Waun Mawn in Mynydd Preseli was the source and the inspiration for Stonehenge has been criticised by Darvill (2022) and others and has now been abandoned (Bevins et al., 2022).
This is the first well-documented small glacial clast to have been described in a Stonehenge context. It is noteworthy that its finders, Hawley and Newall, did not recognise its significance. However, in 1924 they were clearly heavily influenced by the forceful views of Herbert H. Thomas, who had declared that “the geological evidence is such that the idea of a glacial origin for the foreign stones will not bear investigation” (Thomas, 1923). He had also declared that his own evidence “permanently disposes of the idea of glacial transport”. This view was not seriously challenged in 1923, and it has prevailed in archaeological circles for almost a century, in spite of the fact that the “human transport hypothesis” has remained unproven.
With regard to the exact origins of the boulder, it may have come from one of the rhyolitic tuff exposures in the Mynydd Preseli area, but the geological work done thus far does not support provenancing to the Rhos-y-felin–Pont Saeson area (Bevins et al., 2023).
This preliminary investigation, which must be followed and corrected by more detailed research by experts in a number of fields, strongly suggests that glacially deposited materials and far-travelled erratics unrelated to the bluestone monoliths do exist at Stonehenge. This is what has been suspected for many years and proposed by Kellaway (1971, 1991), Thorpe et al. (1991) and John (2018a). The interpretations made by Thomas (1923) and repeated by many other Stonehenge researchers and commentators have not been verified by recent research. This also brings into question the views of geologists Robert Ixer and Richard Bevins: (1) that the bluestone fragments and small boulders at Stonehenge must have been quarried and then transported by humans from Pembrokeshire, (2) that the debris must be related to the known (and unknown) bluestone monoliths in the stone settings, and (3) that there was an identifiable Late Neolithic “arrival date” for the bluestones at the site of the monument.
The complex narrative concerning the Stonehenge bluestones, involving large-scale bluestone quarries at Craig Rhos-y-felin and Carn Goedog and a “lost stone circle” at Waun Mawn (Parker Pearson et al., 2015; Bevins et al., 2013; Ixer and Bevins, 2011a, 2013; Parker Pearson et al., 2021), appears to this author as a classic example of interpretative inflation (Barclay and Brophy, 2020). It must therefore be re-examined. There are no demonstrable cultural links between the three Pembrokeshire sites or with Stonehenge (Darvill, 2022; Bevins et al., 2022).
The simplest explanation of the presence of the bluestones at Stonehenge is that they are glacial erratics from the west, emplaced by ice at some site still to be discovered, on or near Salisbury Plain, where they were later collected up and used by the builders of the stone monument.
No data sets were used in this article.
The author has declared that there are no competing interests.
Publisher’s note: Copernicus Publications remains neutral with regard to jurisdictional claims made in the text, published maps, institutional affiliations, or any other geographical representation in this paper. While Copernicus Publications makes every effort to include appropriate place names, the final responsibility lies with the authors.
I am grateful to Adrian Green, Director of Salisbury Museum, for permission to examine and photograph the Newall Boulder and to publish this article. I also thank Lizzie Richmond for information from the Kellaway Collection in the Library of Bath University, which was invaluable for confirming the history of the boulder since its initial discovery in 1924. David Evans and an anonymous referee are thanked for their constructive comments which have led to significant improvements since the first drafting of this paper.
This paper was edited by Christopher Lüthgens and reviewed by David Evans and one anonymous referee.
Atkinson, R. J. C.: Stonehenge, Hamilton, London, 224 pp., ISBN 014 02 0450 4, 1979.
Barclay, G. J. and Brophy, K.: 'A Veritable Chauvinism of Prehistory': Nationalist Prehistories and the 'British' Late Neolithic Mythos, Archaeological Journal, 178, 330–360, https://doi.org/10.1080/00665983.2020.1769399, 2020.
Benn, D. I. and Ballantyne, C. K.: Reconstructing the transport history of glacigenic sediments: a new approach based on the co-variance of clast shape indices, Sediment. Geol., 91, 215–227, https://doi.org/10.1016/0037-0738(94)90130-9, 1994.
Benn, D. I. and Evans, D. J. A.: The interpretation and classification of subglacially-deformed materials, Quaternary Sci. Rev., 15, 23–52, 1996.
Benn, D. I. and Evans, D. J. A.: Glaciers and Glaciation, Hodder, London, 802 pp., 2010.
Benn, D. I. and Lukas, S.: Clast morphology, in: A Practical Guide to the Study of Glacial Sediments, edited by: Evans, D. J. A. and Benn, D. I., QRA, London, 107–124, ISBN 9780203783481, 2021.
Bevins, R. E., Ixer, R. A., and Pearce, N. G.: Carn Goedog is the likely major source of Stonehenge doleritic bluestones: evidence based on compatible element geochemistry and principal components analysis, J. Archaeol. Sci., 42, 179–193, https://doi.org/10.1016/j.jas.2013.11.009, 2013.
Bevins, R. E., Pearce, N. J. G., Parker Pearson, M., and Ixer, R. A.: Identification of the source of dolerites used at the Waun Mawn stone circle in the Mynydd Preseli, west Wales and implications for the proposed link with Stonehenge, Journal of Archaeological Science: Reports, 45, 103556, https://doi.org/10.1016/j.jasrep.2022.103556, 2022.
Bevins, R. E., Ixer, R. A., Pearce, N. G., Scourse, J., and Daw, T.: Lithological description and provenancing of a collection of bluestones from excavations at Stonehenge by William Hawley in 1924 with implications for the human versus ice transport debate of the monument's bluestone megaliths, Geoarchaeology, 38, 771–785, https://doi.org/10.1002/gea.21971, 2023.
Boulton, G. S.: Boulder shapes and grain-size distributions of debris as indicators of transport paths through a glacier and till genesis, Sedimentology, 25, 773–779, 1978.
Bowen, D. Q.: South Wales, in: The Glaciations of Wales and Adjacent Areas, edited by: Lewis, C. and Richards, A. E., Logaston Press, 145–164, ISBN 1 904396 36 4, 2005.
Briggs, C. S.: Stone axe “trade” or glacial erratics?, Current Archaeology, 57, p. 303, 1977.
Burl, A.: A Brief History of Stonehenge, Robinson, 368 pp., ISBN 9781845295912, 2007.
Burrow, S.: The Tomb Builders, National Museum Wales, 150 pp., ISBN 0 7200 0568 X, 2006.
Clapperton, C. M. and Sugden, D. E.: The glaciation of Buchan – a reappraisal, in: Quaternary studies in North East Scotland, edited by: Gemmell, A. M. D., Department of Geography, University of Aberdeen, 19–22, 1975.
Cleal, R., Walker, K. E., and Montague, R.: Stonehenge in its landscape: 20th century excavations, English Heritage, 618 pp., ISBN 1 85074 605 2, 1995.
Clark, C. D., Ely, J. C., Hindmarsh, R. C. A., Bradley, S., Ignéczi, A., Fabel, D., Ó Cofaigh, C., Chiverrell, R. C., Scourse, J., Benetti, S., Bradwell, T., Evans, D. J. A., Roberts, D. H., Burke, M., Callard, S. L., Medialdea, A., Saher, M., Small, D., Smedley, R. K., Gasson, E., Gregoire, L., Gandy, N., Hughes, A. L. C., Ballantyne, C., Bateman, M. D., Bigg, G. R., Doole, J., Dove, D., Duller, G. A. T., Jenkins, G. T. H., Livingstone, S. L., McCarron, S., Moreton, S., Pollard, D., Praeg, D., Sejrup, H. P., Van Landeghem, K. J. J., and Wilson, P.: Growth and retreat of the last British–Irish Ice Sheet, 31 000 to 15 000 years ago: the BRITICE-CHRONO reconstruction, Boreas, 51, 699–758, https://doi.org/10.1111/bor.12594, 2022.
Darvill, T.: Mythical rings? Waun Mawn and Stonehenge Stage 1, Antiquity, 96, 1515–1529, https://doi.org/10.15184/aqy.2022.82, 2022.
Darvill, T. and Wainwright, G.: Neolithic and Bronze Age Pembrokeshire, Chap. 2, in: Pembrokeshire County History, Vol. 1, Pembrokeshire County History Trust, 55–222, ISBN 0 903771 16 0, 2016.
Evans, D. J. A.: Landscapes at the Periphery of Glacierization – Retrospect and Prospect, Scot. Geogr. J., 132, 140–163, https://doi.org/10.1080/14702541.2016.1156732, 2016.
Evans, D. J. A.: Till – A Glacial Process Sedimentology, Wiley-Blackwell, Chichester, ISBN 9781118652596, 2018.
Evans, D. J. A., Phillips, E., Hiemstra, J. F., and Auton, C. A.: Subglacial till: Formation, sedimentary characteristics and classification, Earth-Sci. Rev., 78, 115–176, https://doi.org/10.1016/j.earscirev.2006.04.001, 2006.
Evans, D. J. A., Roberts, D. H., and Evans, S. C.: Multiple subglacial till deposition: a modern exemplar for Quaternary palaeoglaciology, Quaternary Sci. Rev., 145, 183–203, https://doi.org/10.1016/j.quascirev.2016.05.029, 2016.
Evans, D. J. A., Roberts, D. H., Hiemstra, J. F., Nye, K. M., Wright, H., and Steer, A.: Submarginal debris transport and till formation in active temperate glacier systems: the southeast Iceland type locality, Quaternary Sci. Rev., 195, 72–108, https://doi.org/10.1016/j.quascirev.2018.07.002, 2018.
Field, D., Anderson-Whymark, H., Linford, N., Barber, M., Bowden, M., Linford, P., Topping, P., Abbott, M., Bryan, P., Cunliffe, D., Hardie, C., Martin, L., Payne, A., Pearson, T., Small, F., Smith, N., Soutar, S., and Winton, H.: Analytical Surveys of Stonehenge and its Environs, 2009–2013: Part 2 – the Stones, P. Prehist. Soc., 81, 125–148, https://doi.org/10.1017/ppr.2015.2, 2015.
Gibson, S. M., Bateman, M. D., Murton, J. B., Barrows, T., Fifield, L. K., and Gibbard, P. L.: Timing and dynamics of Late Wolstonian Substage 'Moreton Stadial' (MIS 6) glaciation in the English West Midlands, UK, Roy. Soc. Open Sci., 9, 220312, https://doi.org/10.1098/rsos.220312, 2022.
Gibbard, P. L., Hughes, P. D., and Rolfe, C. J.: New insights into the Quaternary evolution of the Bristol Channel, UK, J. Quaternary Sci., 32, 15 pp., https://doi.org/10.1002/jqs.2951, 2017.
Gibbard, P. L., Hughes, P. D., Clark, C. D., Glasser, N. F., and Tomkins, M. D.: Chapter 34 – Britain and Ireland: glacial landforms prior to the Last Glacial Maximum, European Glacial Landscapes, Maximum Extent of Glaciations, 245–253, https://doi.org/10.1016/B978-0-12-823498-3.00050-9, 2022.
Gilbertson, D. S. and Hawkins, A. B.: The Pleistocene Succession at Kenn, Somerset, Bulletin of the Geological Survey of Great Britain, 66, 41 pp., 1978.
Gowland, W.: Recent excavations at Stonehenge, Wiltshire Magazine, June 1903, 1–46, 1903.
Green, C. P.: Pleistocene river gravels and the Stonehenge problem, Nature, 243, 214–216, 1973.
Green, C. P.: The provenance of rocks used in the construction of Stonehenge, in: Science and Stonehenge, edited by: Cunliffe, B. and Renfrew, C., P. Brit. Acad., 92, 257–270, 1997.
Green, G. W.: British regional geology: Bristol and Gloucester region, 3rd edn., HMSO for the British Geological Survey, London, ISBN 978 0118844826, 1992.
Håkansson, L., Alexandersson, H., Hjort, C., Mölle, P., Briner, J. P., Aldahan, A., and Possnert, G.: Late Pleistocene glacial history of Jameson Land, central East Greenland, derived from cosmogenic 10Be and 26Al exposure dating, Boreas, 38, 244–260, https://doi.org/10.1111/j.1502-3885.2008.00064.x, 2009.
Hawley, W.: The Excavations at Stonehenge: Interim Report on the exploration, Antiq. J., 1, 9–41, 1921.
Hawley, W.: Report on the excavations at Stonehenge during the season 1924, Antiq. J., 6, 1–25, 1926.
Howells, M. F.: Wales: British Regional Geology, BGS, 230 pp., ISBN 0 85272 584 9, 2007.
Hubbard, A., Bradwell, T., Golledge, N., Hall, A., Patton, H., Sugden, D., Cooper, R., and Stoker, M.: Dynamic cycles, ice streams and their impact on the extent, chronology and deglaciation of the British–Irish ice sheet, Quaternary Sci. Rev., 28, 759–777, https://doi.org/10.1016/j.quascirev.2008.12.026, 2009.
Hunt, C. O.: Quaternary of South-West England, in: Geological Conservation Review Series, edited by: Campbell, S., Hunt, C. O., Scourse, J. D., Keen, D. H., and Stephens, N., Joint Nature Conservation Committee, Springer Science, 287–363, 1998.
Ixer, R. A. and Bevins, R. E.: The petrography, affinity and provenance of lithics from the Cursus Field, Stonehenge, Wiltshire Archaeological and Natural History Magazine, 103, 1–15, 2010.
Ixer, R. A. and Bevins, R. E.: Craig Rhos-y-Felin, Pont Saeson is the dominant source of the Stonehange rhyolitic 'debitage', Archaeology in Wales, 50, 21–31, 2011a.
Ixer, R. A. and Bevins, R. E.: The detailed petrography of six orthostats from the bluestone circle, Stonehenge, Wiltshire Archaeological and Natural History Magazine, 104, 1–14, 2011b.
Ixer, R. A. and Bevins, R. E.: Chips off the old block: the Stonehenge debitage dilemma, Archaeology in Wales, 52, 11–22, 2013.
Ixer, R. A. and Bevins, R. E.: The bluestones of Stonehenge, Geology Today, 33, 180–184, https://doi.org/10.1111/gto.12198, 2017.
Ixer, R. A., Bevins, R., and Pirrie, D.: Provenancing the stones: mapping the Stonehenge bluestones using mineralogy, Current Archaeology, 366, 34–41, https://archaeology.co.uk/articles/features/provenancing-the-stones.htm (last access: 28 May 2024), 2020.
Ixer, R. A., Bevins, R., Pearce, N., and Dawson, D.: Victorian gifts: new insights into the Stonehenge bluestones, Current Archaeology, 29 August 2022, https://the-past.com/feature/victorian-gifts-new-insights-into-the-stonehenge-bluestones/ (last access: 28 May 2024), 2022.
John, B. S.: The Stonehenge Bluestones, Greencroft Books, 256 pp., ISBN 978 0905559 94 0, 2018a.
John, B. S.: Evidence for extensive ice cover on the Isles of Scilly, Quaternary Newsletter, 146, 3–27, https://www.researchgate.net/publication/328413421_Evidence_ for_extensive_ice_cover_on_the_Isles_of_Scilly (last access: 28 May 2024), 2018b.
John, B. S.: Was there a Late Devensian ice-free corridor in Pembrokeshire?, Quaternary Newsletter, 158, 5–16, https://www.qra.org.uk/mp-files/qn158_1_late-devensian-ice- free-corridor-in-pembrokshire.pdf/ (last access: 28 May 2024), 2023.
John, B. S., Elis-Gruffydd, D., and Downes, J.: Observations on the supposed “Neolithic Bluestone Quarry” at Craig Rhosyfelin, Pembrokeshire, Archaeology in Wales, 54, 139–148, https://www.researchgate.net/publication/286775899_OBSERVATIONS_ON_THE_SUPPOSED_NEOLITHIC_BLUESTONE_QUARRY_AT_CRAIG_RHOSYFELIN_PEMBROKESHIRE (last access: 28 May 2024), 2015a.
John, B. S., Elis-Gruffydd, D., and Downes, J.: Quaternary Events at Craig Rhosyfelin, Pembrokeshire, Quaternary Newsletter, 137, 16–32, https://www.researchgate.net/publication/283643851_QUATERNARY_EVENTS_AT_CRAIG_RHOSYFELIN_PEMBROKESHIRE (last access: 28 May 2024), 2015b.
Johnson, A.: Solving Stonehenge, Thames & Hudson, 288 pp., ISBN 978 0 500 05155 9, 2008.
Judd, W.: Note on the nature and origin of the rock fragments found in the excavations made at Stonehenge by Mr Gowland in 1901, Wiltshire Magazine, 47–61, 1903.
Kellaway, G. A.: Glaciation and the stones of Stonehenge, Nature, 233, 30–35, https://doi.org/10.1038/233030a0, 1971.
Kellaway, G. A.: The older Plio-Pleistocene glaciations of the region around Bath, in: Hot Springs of Bath, edited by: Kellaway, G. A., Bath CC, 243–241, 1991.
Krüger, J.: Clasts with stoss-lee forms in lodgement tills: a discussion, J. Glaciol., 30, 241–243, 1984.
Lee, J. R., Rose, J., Hamblin, R. J. O., and Moorlock, B. S. P.: Dating the earliest lowland glaciation of eastern England: A pre-MIS 12 early Middle Pleistocene Happisburgh glaciation, Quaternary Sci. Rev., 23, 1551–1566, https://doi.org/10.1016/j.quascirev.2004.02.002, 2004.
Lee, J. R., Rose, J., Hamblin, R. J., Moorlock, B. S., Riding, J. B., Phillips, E., Barendregt, R. W., and Candy, I.: The Glacial History of the British Isles during the Early and Middle Pleistocene: Implications for the long-term development of the British Ice Sheet, edited by: Ehlers, J., Gibbard, P. L., and Hughes, P. D., Developments in Quaternary Sciences, 15, 59–74, https://doi.org/10.1016/B978-0-444-53447-7.00006-4, 2011.
Lukas, S., Benn, D. I., Boston, C. M., Brook, M. S., Coray, S., Evans, D. J. A., Graf, A., Kellerer-Pirklbauer-Eulenstein, A., Kirkbride, M. P., Krabbendam, M., Lovell, H., Machiedo, M., Mills, S. C., Nye, K., Reinardy, B. T. I., Ross, F. H., and Signer, M.: Clast shape analysis and clast transport paths in glacial environments: A critical review of methods and the role of lithology, Earth-Sci. Rev., 121, 96–116, https://doi.org/10.1016/j.earscirev.2013.02.005, 2013.
Madgett, P. A. and Inglis, E. A.: A Re-Appraisal of the Erratic Suite of the Saunton and Croyde Areas, North Devon, Rep. Transactions of the Devon Association for the Advancement of Science, 119, 135–144, 1987.
Parker Pearson, M.: Stonehenge: exploring the greatest Stone Age mystery, Simon and Schuster, 406 pp., ISBN 978 0 85720 730 2, 2012.
Parker Pearson, M., Bevins, R. E., Ixer, R. A., Pollard, J., Richards, C., Welham, K., Chan, B. K., Edinborough, K., Hamilton, D., McPhail, R., Schlee, D., Schweenninger, J.-L., Simmons, E., and Smith, M.: Craig Rhos-y-felin: a Welsh bluestone megalith quarry for Stonehenge, Antiquity, 89, 1331–1352, https://doi.org/10.15184/aqy.2015.177, 2015.
Parker Pearson, M., Pollard, J., Richards, C., Welham, K., Kinnaird, T., Shaw, D., Simmons, E., Stanford, A., Bevins, R. E., Ixer, R. A., Ruggles, C., Rylatt, J., and Edinborough, K.: The original Stonehenge? A dismantled stone circle in the Preseli Hills of west Wales, Antiquity, 95, 85–103, https://doi.org/10.15184/aqy.2020.239, 2021.
Patton, H., Hubbard, A., Andreassen, K., Winsborrow, M., and Stroeven, A. P.: The build-up, configuration, and dynamical sensitivity of the Eurasian ice-sheet complex to Late Weichselian climatic and oceanic forcing, Quaternary Sci. Rev., 153, 97–121, 2016.
Patton, H., Hubbard, A., Andreassen, K., Auriac, A., Whitehouse, P. L., Stroeven, A. P., Shackleton, C., Winsborrow, M., Heymane, J., and Hall, A. M.: Deglaciation of the Eurasian ice sheet complex, Quaternary Sci. Rev., 169, 148–172, https://doi.org/10.1016/j.quascirev.2017.05.019, 2017.
Patton, H., Hubbard, A., Heyman, J., Alexandropoulou, N., Lasabuda, A. P. E., Stroeven, A. P., Hall, A. M., Winsborrow, M., Sugden, D. E., Kleman, J., and Andreassen, K.: The extreme yet transient nature of glacial erosion, Nat. Commun., 13, 7377, https://doi.org/10.1038/s41467-022-35072-0, 2022.
Pitts, M.: How to build Stonehenge, Thames and Hudson, 249 pp., ISBN 978 0 500 02419 5, 2022.
Powers, M. C.: New roundness scale for sedimentary particles, J. Sediment. Res., 23, 117–119, https://doi.org/10.1306/D4269567-2B26-11D7-8648000102C1865D, 1953.
Ramsey, A. C.: Geology of Parts of Wiltshire and Gloucestershire (Sheet 34), HMSO, London, 1858.
Rogers, E.: Diffusion of Innovations, 5th edn, Simon and Schuster, 576 pp., ISBN 978 074322209 9, 2003.
Rose, J., Carney, J. N., Silva, B. N., and Booth, S. J.: A striated, far travelled clast of rhyolitic tuff from Thames river deposits at Ardleigh, Essex, England: evide.nce for early Middle Pleistocene glaciation in the Thames catchment, Neth. J. Geosci., 89, 137–146, 2010.
Scourse, J. D.: Transport of the Stonehenge Bluestones: Testing the Glacial Hypothesis, in: Science and Stonehenge, edited by: Cunliffe, B. and Renfrew, C., British Academy, 271–314, ISBN 0 19 726174 4, 1997.
Scourse, J. D., Saher, M., Van Landeghem, K. J. J., Lockhart, E., Purcell, C., Callard, L., Roseby, Z., Allinson, B., Pieńkowski, A. J., O'Cofaigh, C., Praeg, D., Ward, S., Chiverrell, R., Moreton, S., Fabel, D., Clark, C. D.: Advance and retreat of the marine-terminating Irish Sea Ice Stream into the Celtic Sea during the Last Glacial: Timing and maximum extent, Mar. Geol., 412, 53–68, https://doi.org/10.1016/j.margeo.2019.03.003, 2019.
Sharp, M. J.: Modification of clasts in lodgement tills by glacial erosion, J. Glaciol., 28, 475–481, 1982.
Stone, E. H.: The Stones of Stonehenge, Robert Scott, London, ISBN: 9781169296169, 1924.
Thomas, H. H.: The source of the stones of Stonehenge, The Antiquaries Journal, 3, 239–260, 1923.
Thorpe, R. S., Williams-Thorpe, O., Jenkins, D. G., and Watson, J. S. (with contributions by Ixer, R. A. and Thomas, R. G.): The Geological Sources and Transport of the Bluestones of Stonehenge, Wiltshire, UK, Proceedings of the Prehistoric Society, 57, 103–157, 1991.
Vaughan, D., Phillips, E., Lee, J. R., and Hart, J. K.: Glacitectonic rafting of chalk bedrock: Overstrand, in: Glacitectonics – Field Guide, edited by: Phillips, E., Lee, J. R., and Evans, H., Quaternary Research Association, ISBN 9780907780830, 2011.
Willis, C., Marshall, P., McKinley, J., Pitts, M., Pollard, J., Richards, C., Richards, J., Thomas, J., Waldron, C., Welham, K., and Parker Pearson, M.: The dead of Stonehenge, Antiquity, 90, 337–356, https://doi.org/10.15184/aqy.2016.26, 2016.
- How to cite
- Abstract
- Kurzfassung
- Introduction
- Discovery of the Newall Boulder (RSN18)
- Description of the boulder
- Interpretation: boulder provenance
- Interpretation: shape and surface characteristics
- The evidence for glaciation on Salisbury Plain
- Shortcomings of the human transport hypothesis
- Conclusions
- Data availability
- Competing interests
- Disclaimer
- Acknowledgements
- Review statement
- References
Please read the editorial note first before accessing the article.
- Article
(24162 KB) - Full-text XML
lostfor over 90 years has been found in Salisbury Museum. Its shape and surface characteristics suggest that it was carried from West Wales by the Irish Sea Ice Stream. The author proposes that most if not all of the 43 bluestone monoliths are glacial erratics.
- How to cite
- Abstract
- Kurzfassung
- Introduction
- Discovery of the Newall Boulder (RSN18)
- Description of the boulder
- Interpretation: boulder provenance
- Interpretation: shape and surface characteristics
- The evidence for glaciation on Salisbury Plain
- Shortcomings of the human transport hypothesis
- Conclusions
- Data availability
- Competing interests
- Disclaimer
- Acknowledgements
- Review statement
- References