the Creative Commons Attribution 4.0 License.
the Creative Commons Attribution 4.0 License.
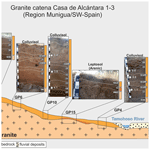
A pedo-geomorphological view on land use and its potential in the surroundings of the ancient Hispano-Roman city Munigua (Seville, SW Spain)
André Kirchner
Nico Herrmann
Paul Matras
Iris Müller
Julia Meister
Thomas G. Schattner
Kirchner, A., Herrmann, N., Matras, P., Müller, I., Meister, J., and Schattner, T. G.: A pedo-geomorphological view on land use and its potential in the surroundings of the ancient Hispano-Roman city Munigua (Seville, SW Spain), E&G Quaternary Sci. J., 71, 123–143, https://doi.org/10.5194/egqsj-71-123-2022, 2022.
This study investigates the surroundings of Munigua (municipium Flavium Muniguense), a small Roman town in the ancient province of Hispania Baetica (SW Spain). The city's economy was based primarily on copper and iron mining, which brought financial prosperity to its citizens. Local production of agricultural goods is thought to have been of little importance, as the regional soil conditions do not seem to be suitable for extensive agriculture.
To evaluate the recent soil agro-potential and to find evidence for prehistoric and historic land use in the surroundings of Munigua, we applied a pedo-geomorphological approach based on the physico-chemical analysis of 14 representative soil and sediment exposures. Selected samples were analyzed for bulk chemistry, texture and phytoliths. The chronostratigraphy of the sequences was based on radiocarbon dating of charcoal samples. The site evaluation of the present-day soil agro-potential was carried out according to standard procedures and included evaluation of potential rootability, available water-storage capacity and nutrient budget within the uppermost 1 m.
The results show that moderate to very good soil agro-potential prevails in the granitic and floodplain areas surrounding Munigua. Clearly, recent soil agro-potential in these areas allows the production of basic agricultural goods, and similar limited agricultural use should also have been possible in ancient times. In contrast, weak to very weak present-day soil agro-potential prevails in the metamorphic landscape due to the occurrence of shallow and sandy to stony soils.
In addition, the study provides pedo-geomorphological evidence for prehistoric and historic land use in pre-Roman, Roman and post-Roman times. Catenary soil mapping in the vicinity of a Roman house complex reveals multi-layered colluvial deposits. They document phases of hillslope erosion mainly triggered by human land use between 4063 ± 82 and 3796 ± 76 cal BP, around 2601 ± 115 cal BP, and between 1424 ± 96 and 421 ± 88 cal BP. Moreover, geochemical and phytolith analyses of a Roman hortic Anthrosol indicate the local cultivation of agricultural products that contributed to the food supply of Munigua.
Overall, the evidence of Roman agricultural use in the Munigua area indicates that the city's economy was by no means focused solely on mining. The production of basic agricultural products was also part of Munigua's economic portfolio. Our geoarcheological study thus supports the archeological concept of economically diversified Roman cities in the province of Baetica and in Hispania.
Diese Studie untersucht die Umgebung von Munigua (municipium Flavium Muniguense), einer kleinen römischen Stadt in der antiken Provinz Hispania Baetica (Südwestspanien). Die Wirtschaft der Stadt basierte in erster Linie auf dem Kupfer- und Eisenbergbau, der den Bürgern finanziellen Wohlstand bescherte. Es wird angenommen, dass die lokale Produktion von landwirtschaftlichen Gütern von geringer Bedeutung war, da insbesondere die regionalen Bodenbedingungen für eine extensive Landwirtschaft nicht geeignet zu sein scheinen.
Um das rezente landwirtschaftliche Potenzial der Böden zu bewerten und mögliche Hinweise für prähistorische und historische Landnutzung in der Umgebung von Munigua zu finden, wurden geoarchäologische Untersuchungen durchgeführt, im Zuge derer 14 repräsentative Boden- und Sedimentaufschlüsse bearbeitet wurden. Ausgewählte Proben wurden auf ihre physikalisch-chemischen Eigenschaften sowie Phytholiten untersucht. Die chronostratigraphische Einordnung erfolgte auf der Grundlage von Radiokohlenstoffdatierungen. Die Standortbewertung des heutigen landwirtschaftlichen Potenzials der Böden wurde nach Standardverfahren durchgeführt und umfasste die Bewertung der potenziellen Durchwurzelbarkeit, der verfügbaren Wasserspeicherkapazität und des Nährstoffhaushalts.
Die Ergebnisse zum landwirtschaftlichen Potenzial der Böden zeigen, dass in Granit- und Auengebieten rund um Munigua ein mäßiges bis sehr gutes Ertragspotenzial vorherrscht, dass die begrenzte Produktion von landwirtschaftlichen Erzeugnissen erlauben würde. Eine vergleichbar begrenzte landwirtschaftliche Nutzung dürfte auch in der Antike möglich gewesen sein. Die Böden in der umgebenden Metamorphitlandschaft besitzen im Gegensatz dazu ein nur schwaches bis sehr schwaches Agrarpotenzial und Hinweise auf eine antike Agrarnutzung sind nicht vorhanden. Darüber hinaus liefert die Studie weitere Hinweise auf prähistorische und historische Landnutzung in vorrömischer, römischer und nachrömischer Zeit. So zeigt eine Catena, die in der Nähe eines römischen Hauskomplexes angelegt wurde, eine reliefabhängige Bodenabfolge mit mehrgliedrigen Hangkolluvien, die anthropogene Bodenerosion zwischen 4063 ± 82 und 3796 ± 76 cal BP, um 2601 ± 115 cal BP, und zwischen 1424 ± 96 und 421 ± 88 cal BP dokumentieren. Darüber hinaus weisen geochemische und Phytolithen-Analysen eines untersuchten römischen Gartenbodens (Hortic Anthrosol) auf den lokalen Anbau landwirtschaftlicher Produkte hin, die zur Nahrungsmittelversorgung von Munigua beitrugen.
Die im Rahmen dieser Untersuchung erzielten Belege für eine landwirtschaftliche Nutzung im Gebiet von Munigua zeigen, dass die Wirtschaft der Stadt keineswegs nur auf den Bergbau ausgerichtet war. Auch die Produktion von landwirtschaftlichen Grunderzeugnissen gehörte zum wirtschaftlichen Portfolio Muniguas. Die Hypothese, dass die lokale Produktion von landwirtschaftlichen Gütern aufgrund eines unzureichenden Ertragspotentials der Böden unbedeutend war, kann somit nicht bestätigt werden. Vielmehr unterstützt diese Studie das archäologische Konzept einer wirtschaftlich diversifizierten römischen Stadt.
- Article
(7223 KB) - Full-text XML
-
Supplement
(396 KB) - BibTeX
- EndNote
During the imperial period, the economy of small Roman cities in the Roman Empire and particularly in the Roman province of Baetica is assumed to have been very diverse and, depending on the city, typically specialized around a certain economic good (Bowman and Wilson, 2009, 2011). In addition to archeological evidence, abundant historical sources provide a vivid and rich picture of ancient agriculture, fishing and mineral resources, basic foodstuffs, and luxury products (e.g., Blázquez Martinez, 1967; Remesal Rodríguez, 2020).
This study was carried out in the surroundings of the Hispano-Roman municipium Flavium Muniguense, in modern times known as Castillo de Mulva or Munigua (Schattner, 2003), a small urban center in the ancient province of Hispania Baetica. From a historiographic perspective, Munigua is a key site for understanding Roman urbanization on the Iberian Peninsula (Schattner, 2005) since it is one of the few places in Spain that has been systematically excavated for the past 65 years. The local economy was particularly based on copper and iron mining in the surroundings of the town, and Munigua was the largest iron-producer in the Sierra Morena in the 1st and 2nd centuries CE (Schattner, 2019b). Income from the mining activities provided financial prosperity for the citizens and an architectural climax, which culminated in the construction of the impressive terrace sanctuary situated on the highest point in the west of the city (Schattner, 2019a, 2021). In addition to the mining industry, olive oil presses and an oil cellar (cella olearia) were located inside the city, indicating the processing of agricultural by-products within the urban area (Schattner, 2003; Peña Cervantes, 2010; Teichner and Peña Cervantes, 2012). Furthermore, wine production and quarrying contributed to economic output even though they were much less significant than the mining operations (Hanel, 1989; Schattner, 2019b).
Current opinion on the food supply strategy of Munigua is based on the assumption that food was obtained in particular from the fertile lower Guadalquivir valley, which was and still is the agricultural center of the region, located just 10 km south of Munigua. Additionally, fish bones and oyster shells indicate connections to the sea (Boessneck and von den Driesch, 1980). In contrast, the local production of agricultural goods is thought to have been of minor relevance (Schattner, 2019b). In this context, it has been suggested that regional soil conditions are currently unsuitable for extensive agriculture and therefore seem to suggest that an ancient agrarian use of the region was also unlikely (Schattner, 2019b). However, so far there has been a lack of evidence-based studies on the agricultural suitability and potential agricultural use of the surroundings of Munigua, and it is only recently that an archeological study has challenged the idea of food imports having been dominant (Krug, 2018). Based on the investigation of small finds, the study stated that tools such as shovels, spades, sickles and billhooks suggest the existence of gardens, meadows for smaller animals and trees which had to be tended and harvested in the wider surroundings of the town (Krug, 2018).
In this paper, we present initial findings from a geoarcheological pilot project and provide new insights into the regional land use history of the Munigua site. Firstly, physico-chemical analysis of 14 representative soil and sediment exposures provides a first evidence-based evaluation of present-day agro-potential. Secondly, we present site-specific evidence of prehistoric and historic land use in the surroundings of the city and thus improve understanding of its food supply strategy. In this way we, thirdly, aim to contribute a pedo-geomorphological view to the archeological debate on the diversification of the urban economy in the Roman province of Baetica (e.g., Bowman and Wilson 2009, 2011), a topic that has not yet been developed for this region of the Roman Empire.
2.1 Environmental setting
Munigua is located in the southern part of the Sierra Morena about 50 km northeast of the Andalusian capital Seville (Fig. 1a). To the south the region is bordered by the wide valley of the lower Guadalquivir River, which drains large parts of Andalusia and today flows into the Atlantic Ocean southwest of Seville. The region experiences a dry, sub-humid, semi-oceanic Mediterranean climate, with arid summers and winter rains due to low-pressure systems from the Atlantic Ocean (Zazo et al., 2008). Average rainfall is around 580 mm yr−1, and the annual average temperature is 18.0 ∘C (Gómez-Zotano et al., 2015), leading to species-rich Mediterranean vegetation cover with evergreen woody plants such as Quercus faginea, Quercus suber, Quercus rotundifolia, Olea europaea L. and Chamaerops humilis (Frey and Lösch, 2010). In steeper areas, the taller trees are replaced by maquis shrubland, mainly composed of hardwood bushes (e.g., Cistus spp.). However, as in the entire Sierra Morena, vegetation has been greatly altered by centuries of agrosilvopastoral farming, in Spanish known as dehesa farming (Joffre et al., 1988), resulting in scattered forest cover accompanied by grassland (Fig. 1c and d).
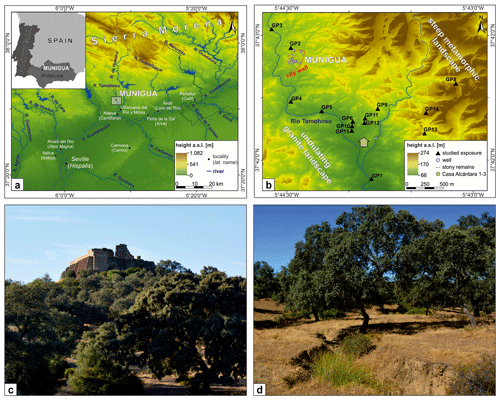
Figure 1(a) Location of Munigua (grey box) in modern SW Spain. (b) Investigated soil and sediment exposures within the surroundings of Munigua. GP8, GP13 and GP14 are located in a metamorphic landscape southeast of the city. GP2, GP4, GP6, GP9, GP10, GP11, GP12 and GP15 are in granite areas, and GP3, GP5 and GP7 are within the floodplain of the Tamohoso River. (c) South view of Munigua's terrace sanctuary surrounded by scattered forests and grassland. (d) Undulated granite landscape with loose holm oak (Quercus rotundifolia) and grass understory, typical for the Munigua region. The surface is dissected by a gully about 1.3 m in depth, indicating modern linear soil erosion processes.
Geologically, the region belongs to the Ossa-Morena Zone (OMZ), a geotectonic unit of the Iberian Massif (Strauss and Madel, 1974; Tornos et al., 2004; Ribeiro et al., 2010). Granite dominates the petrography in the region (Sanz et al., 1973; Schattner et al., 2005). Geomorphologically, the granite area is mainly characterized by flat to slightly undulating landforms with moderately steep slopes, small depressions and ridges (Fig. 1b). They frequently show linear erosion channels (gullies) and are mostly located close to animal pathways. Steep gully flanks and exposed roots of the existing vegetation crossing the gullies indicate modern gully formation (Fig. 1d).
The granite landscape is surrounded by mountains consisting of metamorphic Paleozoic rocks, mainly shale, metagreywacke and quartzite (Sanz et al., 1973). This landscape is located in the east of the city and is characterized by much steeper relief, exceeding the altitude of the granite landscape by up to 100 m (Fig. 1b). The main settlement of Munigua with its residential houses and monumental buildings is situated on a morphologically very resistant rhyolite dike (Sanz et al., 1973; Schattner et al., 2005), forming a gently inclined hill that is up to 30 m higher than the adjacent granite landscape (Fig. 1c). Along the Tamohoso River, flowing through the west of Munigua and draining into the Guadalquivir River via the Huesna River (Fig. 1a), a flat alluvial plain has developed in wider valley sections, covering the denudated granite basement (Sanz et al., 1973).
Regional soil investigations in the western sector of the Sierra Morena show poorly developed Regosols, Leptosols and Cambisols. There are frequent erosional features which are often associated with increases in land use over the past two millennia. In consequence, more advanced soil development, leading to the formation of Luvisols or Acrisols, is restricted to small areas (e.g., Paneque and Bellinfante, 1964; Nuñez and Recio, 2002; Recio et al., 2002).
2.2 Archeological background
Munigua is located on the edge of the Iberian Pyrite Belt (IPB), one of the largest and most important volcanogenic polymetallic sulfide districts worldwide, which has been exploited since late prehistoric times (Nocete et al., 2011). The mining importance of Munigua is based in particular on the copper- and iron-bearing ores (e.g., pyrite, chalcopyrite) found near the town (Schattner et al., 2005; Schattner, 2019a).
The oldest archeological finds indicate the presence of humans since the Epipaleolithic period. Especially for the Early (approx. 1750–1550 BCE) and Middle Bronze Age (approx. 1550–1150 BCE), several fortified settlements have been identified and linked to copper mining and the processing and export of mining products to the Guadalquivir valley, a region with limited mineral resources that was highly dependent on continuous supplies from the Sierra Morena mines (Schattner, 2019b). In the 4th century BCE (approx. 360–330 BCE), a small pre-Roman (Turdetan) settlement was founded on the top of the aforementioned rhyolite dike. Traces of urban structure are noticeable from the first half of the 1st century BCE, for instance documented by the construction of thermae (Chic García, 1997; Gutiérrez-Rodríguez et al., 2019; Schattner, 2019b). In 74 CE the settlement was awarded the rank of a municipium after Emperor Vespasian's edict and experienced its heyday. This is revealed by comprehensive urban development and the construction of imposing religious and public buildings like, for example, the terrace sanctuary and the forum. Thus, Munigua possessed a large variety of the typical structural features of a Roman city, although the total size of the settlement was only about 3.8 ha (Ullrich et al., 2007; Schattner, 2019b). Some villas and smaller settlements were established in the surroundings of the city in this period, for example the Roman house complex Casa de Alcántara 1, 2 and 3 located about 1 km southeast of the city (Fig. 1b; Meyer et al., 2007; Schattner, 2019b). At this time, Munigua was part of a dense urban network consisting of some 195 towns and nucleated settlements established in central and western Baetica between approx. 500 BCE and 200 CE (Keay and Earl, 2011).
In the late and post-Roman period (3rd century CE), urban development reached an inflection point expressed by massive depopulation and drastic changes in urban structure. This is thought to be related to seismic activities that caused structural damage to the city, and similar occurrences are also recorded in other Roman cities of the Baetica province (e.g., Rodríguez-Pascua et al., 2011; Giner-Robles et al., 2016; Ruiz-Bueno, 2017). Smaller population groups retreated to the hills in the surroundings where there are traces of a human presence until the end of the 4th century CE (Grünhagen, 1959). The existence of a settlement is documented for Munigua until at least the 7th century CE, even though it is uncertain if ore mining and smelting were still practiced at that time (Eger, 2016). An Arab coin, typical Islamic vessels, oil lamps made of terracotta and burials of Muslim individuals indicate an Islamic settlement on the hill of Munigua from approximately the 8th to the 12th century CE (Teichner, 1998; Eger, 2016). Probably due to its exposed location, Munigua was not reoccupied until its rediscovery in the 16th to 17th century CE (Schattner, 2005). With the industrial expansion of carboniferous coal extraction in the nearby city of Villanueva del Río y Minas (Fig. 1a), regional population density and land use intensity (especially grazing) massively increased during the 19th and 20th centuries CE (Tomás García, 1991).
3.1 Field methods
A total of 14 representative exposures were selected for field investigations: three sites with metamorphic lithology (GP8, GP13 and GP14), eight sites in granite areas (GP2, GP4, GP6, GP9, GP10, GP11, GP12 and GP15) and three floodplain sites (GP3, GP5 and GP7; Fig. 1b). Taking the local relief conditions into account, the soil profiles were arranged in a catenary manner along hillslopes (Semmel, 1977; Evans and Hartemink, 2014; Borden et al., 2020).
Macroscale features were subsequently described by following the German soil mapping instructions (Ad-hoc-AG Boden, 2005). Identified soil horizons were designated according to Jahn et al. (2006) and Zádorová and Penížek (2018). Soil classifications were conducted according to the IUSS Working Group WRB (2015). An exception was made only for colluvium or colluvial deposits, which were used as geoarchives documenting human-induced soil erosion (Dotterweich, 2008; Kittel, 2014), following the definition by Leopold and Völkel (2007, p. 134). Accordingly, colluvium and colluvial deposits are defined as “sediments deposited due to anthropogenic-induced soil erosion, caused by settling, clearing, mining, grazing, and/or farming.” According to Zádorová and Penížek (2018) the soil horizon symbol “M” (migrare [lat.]; Ad-hoc-AG Boden, 2005) was added, and multiple layers of colluvial deposits within a profile were separated by using suffix numbers, in which the number 1 was always given to the uppermost colluvium. If the overall thickness of colluvial deposits exceeded 50 cm, the soil type was classified as Colluvisol. Otherwise, the term “colluvic” was used as a prefixed qualifier.
In contrast, slope sediments that were mainly mobilized and relocated by natural erosion were classified as slope deposits. Field diagnostic properties have been used to separate colluvial and slope deposits. Slope deposits dominated by properties from the geological subsurface show a denser packing and do not contain anthropogenic remains. In contrast colluvial deposits frequently show a comparable loose packing and anthropogenic remains like pottery shards, slag fragments and bricks.
3.2 Laboratory methods
A total of 57 samples were taken from 10 of the 14 profiles studied in the field and were subjected to further laboratory treatment (Table S1 in the Supplement). Prior to physico-chemical analyses, samples were air dried and the fine-earth fraction (≤ 2.0 mm) was sieved. Grain size analyses utilized a combination of the Köhn pipette method (silt and clay fractions) and wet sieving (sand fractions) (DIN ISO 11277, 2002). Samples were pre-treated with 0.4 M sodium pyrophosphate (Na4P2O7) overnight and afterwards shaken for 6 h. When soil organic matter (SOM) exceeded 2.0 %, the samples were additionally pre-treated with 17.5 % hydrogen peroxide (H2O2). The sand fraction was divided into coarse (2000–630 µm), medium (630–200 µm) and fine (200–63 µm) sand by wet sieving using the vibratory sieve shaker AS 200 (Retsch). Coarse silt (63–20 µm), medium silt (20–6.3 µm), fine silt (6.3–2.0 µm) and clay (< 2.0 µm) were identified by the Köhn pipette method using the SEDIMAT 4–12 (UGT).
Total carbon (Ct) and total nitrogen (Nt) were measured on milled samples using the CN analyzer vario EL cube (Elementar). Due to the absence of inorganic carbon, as proven in pretests using hydrochloric acid (10 %), the Ct is equal to the amount of total organic carbon (TOC). TOC values were multiplied by 1.724 (Amelung et al., 2018) in order to obtain contents of soil organic matter (SOM). C N ratio was calculated by dividing TOC by Nt (Blume et al., 2011).
The plant-available macronutrients magnesium (Mg), calcium (Ca), sodium (Na) and potassium (K) were extracted with 1 M NH4NO3 and quantified by an atomic absorption spectrophotometer (Perkin Elmer PinAAcle 900T). Additionally, K2O and P2O5 were measured according to the calcium–acetate–lactate method (Schüller, 1969) using a Spectronic C301 spectrophotometer (Milton Roy). The pH value was measured in a 1 : 2.5 suspension in 0.01 M CaCl2 (Jahn et al., 2006).
The content of total phosphorus (Pt), used in archeology as an important indicator for human activity in agricultural and pre-agricultural societies (e.g., Holliday and Gartner, 2007; Weihrauch, 2018), was determined by colorimetry with the spectrophotometer C21 (Milton Roy) following ignition and then acid extraction based on Bleck (1965).
The soil agro-potential of the site was evaluated according to standard procedures (Blume et al., 2011; Amelung et al., 2018), including assessment of potential rootability, the available water-storage capacity and the nutrient budget within the uppermost 1 m. All evaluations consider the total particle-size fractions (coarse and fine-earth fractions) and further take account of the field-estimated bulk density (Ad-hoc-AG Boden, 2005). For stony soils and sediments, deductions have to be made from the available soil volume, which influences the nutrient budget and the water-storage capacity accordingly (Blume et al., 2011). The evaluation was based on a five-step classification including the following classes: (1) very favorable/very good, (2) favorable/good, (3) moderate, (4) unfavorable/weak and (5) very unfavorable/very weak. Blume et al. (2011) suggested the following values for the classification of potential rootability: (1) > 120 cm, (2) 70 to 120 cm, (3) 30 to < 70 cm, (4) 15 to < 30 cm and (5) < 15 cm. The available water-storage capacity was assessed according to the following values: (1) > 200 L m−2, (2) 140 to 200 L m−2, (3) 90 to < 140 L m−2, (4) 50 to < 90 L m−2 and (5) < 50 L m−2. The classification of the nutrient budget was mainly derived from the contents of macronutrients (Mg, Ca, Na and K), grain size distribution, SOM content and pH values, which primarily determine the base saturation and effective cation exchange capacity (Ad-hoc-AG Boden, 2005; Blume et al., 2011). Possible deficiencies in nitrogen and calcium-lactate-soluble phosphorus (P2O5) and potassium (K2O) contents were additionally checked and included in the assessment of the nutrient budget. For GP5, GP9, GP10 and GP14, the evaluation is based on field assessments and conclusions drawn by analogy, considering comparable nearby profiles from this study.
Phytolith extraction followed the procedures outlined by Albert et al. (1999). Approximately 1 g of air-dried sample (< 2 mm) was treated with 3 N HCl, 3 N HNO3 and H2O2 to remove carbonates, phosphates and organic material. The mineral components of the samples were separated according to their densities using ∼ 2.4 g mL−1 sodium polytungstate solution [Na6(H2W12O40)H2O]. Slides were prepared by weighing out about 1 mg of sample onto a microscope slide and mounting with Entellan new (Merck). Counting was performed using a KERN OBE-114 microscope at 400× magnification. A total of 200 phytoliths were identified and counted in each sample wherever possible. Unidentifiable phytoliths were counted and recorded as weathered morphotypes. Images of selected phytoliths were recorded using a KERN ODC 825 microscope camera. The numbers of phytoliths per gram of sample were estimated by relating the phytolith amounts and weights of the processed sample material to the initial sample weights. The morphological identification of phytoliths was based on standard literature (Twiss et al., 1969; Brown, 1984; Mulholland and Rapp, 1992; Piperno, 2006), as well as on modern plant reference collections from the Mediterranean area (Albert, 2000; Albert and Weiner, 2001; Tsartsidou et al., 2007; Portillo et al., 2014; Meister et al., 2017). The International Code for Phytolith Nomenclature was followed where possible (Madella et al., 2005; Neumann et al., 2019).
Radiocarbon dating was carried out on 17 charcoal samples at the Curt-Engelhorn-Centre of Archaeometry (MAMS) and at Beta Analytic (BETA) using the accelerator mass spectrometer (AMS) technique (e.g., Hajdas, 2008). Charcoal samples were pretreated using the acid–alkali–acid method to remove contamination by carbonates and humic acids (de Vries and Barendsen, 1954). Conventional 14C ages (BP) were calibrated using the IntCal 13 calibration set (Reimer et al., 2013).
4.1 Granite landscape
4.1.1 Casa de Alcántara house complex
A granite area southeast of urban Munigua was chosen for a detailed survey (Fig. 1b) since a Roman rural house complex known as Casa de Alcántara 1–3 and a nearby ancient well document the presence of Roman settlers who may have worked as farmers in ancient times (Meyer et al., 2007; Schattner, 2019b). The schematic catena (Fig. 2) shows shallow Leptosols (GP11) and moderately deep Cambisols (GP15) in upper slope positions and flatter ridge areas. Soil erosion has led to a shallowing of these profiles and frequently to the deposition of a thin colluvial cover (Fig. 2). Here, potential rootability, water-storage capacity and nutrient budget is very weak to weak (Fig. 3, Table S1).
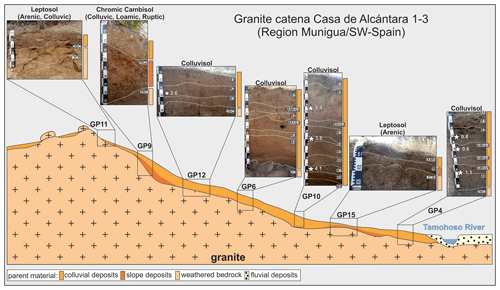
Figure 2Schematic soil catena for a granite landscape in the surroundings of the Roman house complex Casa de Alcántara 1–3. Soil horizon designations are shown in white boxes. Physico-chemical results are summarized in Table S1. White stars within the photographs indicate the positions of 14C-dated charcoal samples (the calibrated ages are given in ka). The shown catena is exaggerated for illustration purposes.
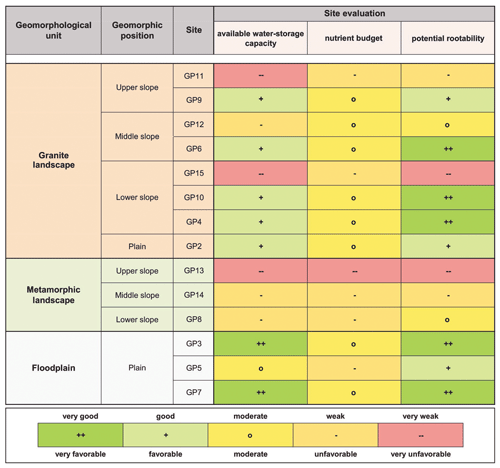
Figure 3Site evaluation of investigated soil and sediment exposures in the Munigua area, subdivided according to the studied geological and geomorphological units.
At the transition from upper to middle slopes Cambisols (GP9) and Colluvisols (GP12, GP6, GP10 and GP4) were mapped. They are deeper, favoring potential rootability and improving water-storage capacity (Fig. 3). Essential nutrients (Mg, Ca, Na, K, P2O5 and K2O) are available (Table S1) although with relatively low values of P2O5 (median = 5.8 mg kg−1, SD = 10.1, n = 18). The pH values range from 4.5 to 6.1 (median = 5.5, SD = 0.5, n = 18) and can accordingly be predominantly classified as slightly acidic (Table S1). The SOM (median = 0.5 %, SD = 1.4, n = 18) and Nt contents (median = 0.04 %, SD = 0.06, n = 18) decrease with depth and are generally low. In sum the nutrient budget of Cambisols and Colluvisols in the surroundings of the Roman house complex Casa de Alcántara 1–3 is moderate (Fig. 3).
For the temporal reconstruction of soil erosion processes around the Casa de Alcántara house complex, seven charcoal samples from three colluvial sequences (GP4, GP10 and GP12) were 14C-dated (Fig. 2, Table 1). The results indicate prehistoric soil erosion phases between around 4063 ± 82 and 3796 ± 76 cal BP (Copper Age to Early Bronze Age), as well as around 2601 ± 115 cal BP (Early Iron Age). Four additional 14C datings from GP10 and GP4 provided ages of 1424 ± 96, 1094 ± 155, 606 ± 51 and 421 ± 88 cal BP.
4.1.2 Profile GP2
Profile GP2 is located less than 100 m north of the fortified urban area of the Muniguan granite landscape (Fig. 1b) in the immediate surroundings of a small, temporary stream and an ancient well (Fig. 4a).
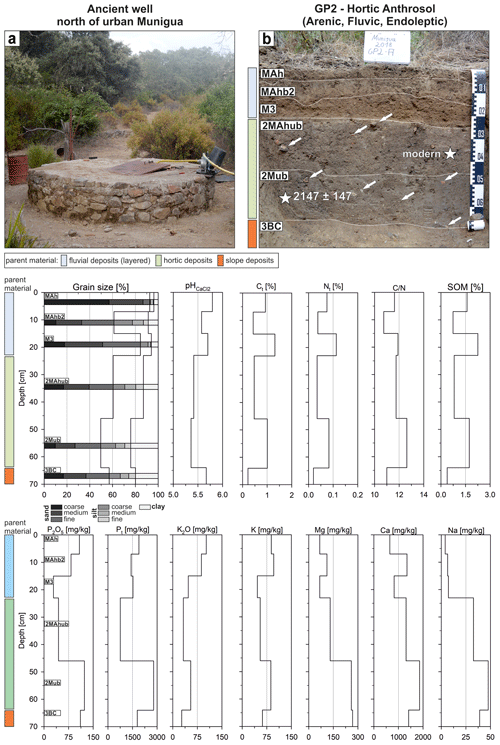
Figure 4(a) Ancient well located outside of Munigua's city wall (Fig. 1b). (b) Excavated hortic Anthrosol (arenic, fluvic, endoleptic). Identified parent material is marked on the left of the profile photo. Soil horizon designations are shown in white boxes. White arrows indicate some larger Roman artifacts (e.g., ceramics, brick). Sampling positions and calibrated 14C ages (cal BP) are marked with white stars. Lower section: laboratory results for the hortic Anthrosol (arenic, fluvic, endoleptic).
The profile was excavated down to granite-derived slope sediments (3BC horizon) present below approximately 64 cm. The uppermost 23 cm are formed by loosely deposited yellow-brown fluvial sediments (Fig. 4b). Missing pedogenic features and an oral report of a recent flood of the above-mentioned adjacent stream suggest that the sediments were deposited relatively recently. The texture is sandy (sand contents between 61 % and 93 %, Fig. 4), and the fluvial deposits mainly show moderate gravel contents (Table S1). Sharply defined, finer-grained substrate follows below 23 cm. This can be further subdivided into an upper (2MAhub, 23–46 cm) and a lower (2Mub, 46–64 cm) horizon by grain size and minor differences in color (10YR 3/3 vs. 2.5YR 3/3). Both horizons contain numerous pottery shards, slag fragments and bricks, all of which can be assigned to the Roman inventory and thus allow these horizons to be archeologically dated to the Roman period (terminus post quem). A 14C-dated charcoal taken at a depth of 56 cm revealed a Roman age of 2147 ± 147 cal BP (Fig. 4b, Table 1). A second piece of charcoal from a depth of 38 cm could not be dated due to its young age (after 1950). The lowermost profile section (64–70 cm) is formed by yellow-brown slope deposits (3BC horizon) showing a loamy texture and does not contain archeological remains.
Measured pH values are moderately acidic and vary slightly from 5.8 to 5.4 (Fig. 4, Table S1). SOM values are between 0.4 % and 2.3 %, and, interestingly, increased SOM content (1.7 %) is found in the lower section of the Roman horizon (2Mub horizon; 46–64 cm). C N ratios vary between 10.75 (MAhb2 horizon) and 12.63 (2Mub horizon). Calcium-lactate-soluble phosphorus (P2O5) and total phosphorus (Pt) show a first maximum of 108 mg kg−1 and 1.895 mg kg−1, respectively, in the uppermost sample (MAh horizon; 0–7 cm), decreasing downwards. However, this trend reverses, and, especially within the lower section of the Roman horizon (2Mub horizon; 46–64 cm), a second maximum is noticeable (123 and 2786 mg kg−1). The same holds true for the macro-nutrient elements Mg, Ca, Na, K and K2O, which are generally increased in the lower part of the Roman horizon and the lowermost slope deposits (Fig. 4, Table S1). Based on diagnostic criteria (color, determine base saturation, SOM, P2O5, thickness) the Roman horizon was classified as a hortic horizon (IUSS Working Group WRB, 2015). In terms of soil agro-potential this site is categorized as good to moderate (Fig. 3).
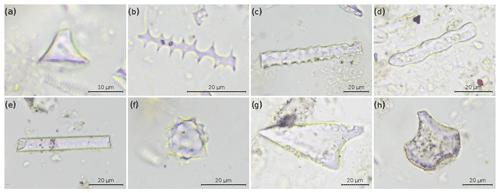
Figure 5Photomicrographs of selected phytolith morphotypes identified in the GP2 samples. The photographs were taken at 400× magnification. (a) Short cell rondel; (b) elongate dendritic; (c) elongate dentate; (d) elongate wavy; (e) elongate entire; (f) spheroid echinate; (g) prickle; (h) bulliform.
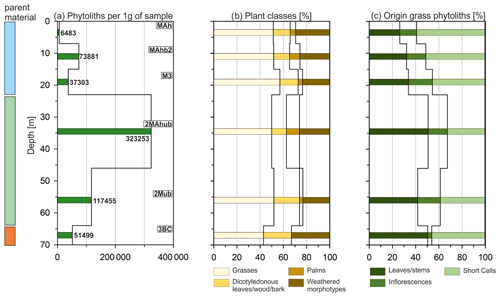
Figure 6Results of phytolith analyses conducted at site GP2. Identified parent material is marked to the left of the graph according to Fig. 4b. Soil horizon designations are shown in white boxes. (a) Phytolith concentrations, (b) relative abundances of phytoliths from different plant classes and (c) anatomical origin of grass phytoliths.
The amounts of phytoliths in the samples vary considerably, ranging from 6483 phytoliths per gram of sediment to 323 253 phytoliths per gram of sediment, while the highest concentrations were reached in the Roman hortic horizons (Fig. 6a; Table S2.2). A total of 33 different phytolith morphotypes were identified in the six samples studied (Fig. 5, Table S2.1). Overall, the samples are similar in their morphotype assemblages (Fig. 6b, Table S2.2). While about 26.8 % (SD = 3.8, n = 6) of the phytoliths were not morphologically identifiable, grass phytoliths, occurring at an average rate of 50.8 % (SD = 4.6, n = 6), were the most common group. The amounts of dicotyledonous leaf, wood and bark phytoliths are relatively low, with an average value of 17.1 % (SD = 4.8, n = 6). Parallelepipedal blocky phytoliths, one of the most common wood/bark morphotypes, for instance, were observed at an average concentration of 10.2 % (SD = 5.9, n = 6). Spheroid echinate phytoliths, commonly associated with palms (Arecaceae), reached an average concentration of 5.3 % (SD = 4.2, n = 6).
The short cell morphologies of the grass phytoliths reveal them to belong mostly to the C3 Pooid subfamily, with rondel and trapezoid short cells commonly produced in the leaves, stems and inflorescences of Pooids. Concentrations between 59.1 % in the uppermost sample and 32.7 % in the upper part of the Roman hortic horizon were found (mean = 45.6 %, SD = 9.3, n = 6; Fig. 6c; Table S2.2). The proportion of inflorescence phytoliths varies slightly around an average concentration of 15.2 % (SD = 6.2, n = 6). The concentrations of phytoliths from grass stems and leaves are higher in the lower profile section (23–70 cm), reaching a maximum of 50.8 % in the upper part of the Roman hortic horizon (mean = 39.2 %, SD = 10.1, n = 6). Due to the absence of multicellular phytoliths in the samples, however, it was not possible to identify the type of grasses.
4.2 Metamorphic landscape
The pedo-morphological conditions were also investigated for three sites (GP8, GP14 and GP13) within the metamorphic landscape, located in the southeast of Munigua (Fig. 1b). Here, the lithology is formed by quartzitic metagreywacke, and the soils were classified as Leptosols or Cambisols (Fig. 7). All observed soils in this metamorphic landscape unit are conspicuously shallow and stony (Table S1). Potential rootability, available water-storage capacity and nutrient budget are weak to very weak (Fig. 3). No geomorphological or pedological evidence of prehistoric or historic land use (e.g., colluvial deposits) is preserved in the observed metamorphic landscape so far.
4.3 Tamohoso River floodplain
The investigated fluvial sequence GP3 is located about 500 m upstream of Munigua's urban infrastructure, whereas GP7 is located about 600 m downstream of the Casa de Alcántara house complex (Fig. 1b). GP5 is situated between these two sites.
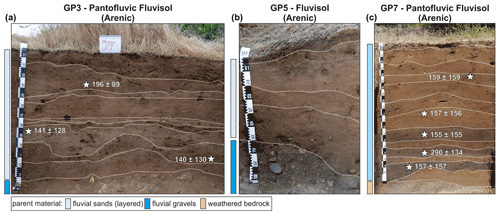
Figure 8Profile photos of fluvial sequences GP3 (a), GP5 (b) and GP7 (c). Identified parent material is marked on the left of the profile photos. Sampling positions for dated charcoal samples and calibrated 14C ages (cal BP) are marked with white stars.
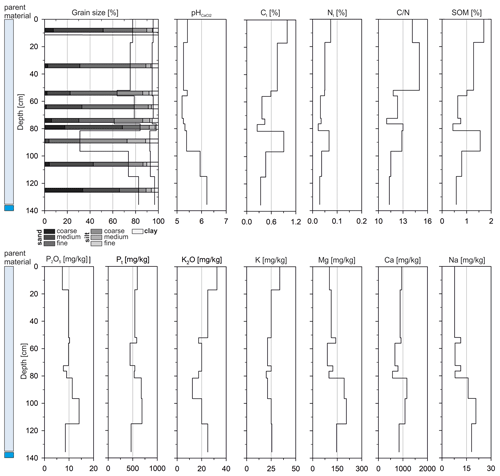
Figure 9Laboratory results for the pantofluvic Fluvisols (Arenic) at floodplain site GP3. Identified parent material is marked according to Fig. 8.
GP3 was excavated to a depth of 145 cm below the surface (Fig. 8). The base of the profile shows coarse-clastic riverbed deposits, classified as moderately rounded gravels or moderately rounded blocks. Upwards, this is followed by alternately deposited, predominantly cross-bedded sands (74 %–84 % sand), which are occasionally interstratified by finer-grained and in particular silty deposits (Figs. 8 and 9). The profile contains charcoal pieces of different sizes in almost all sections, which could indicate the former presence of activities involving fire in the catchment area.
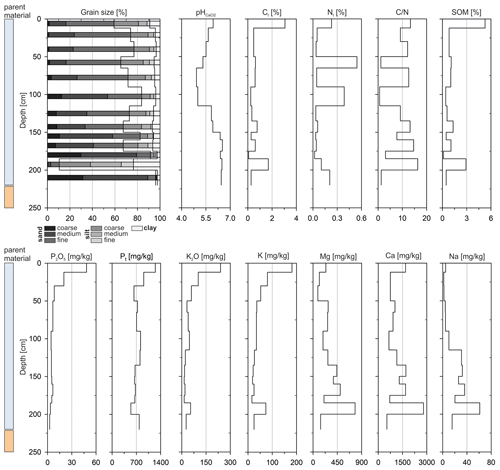
Figure 10Laboratory results for the pantofluvic Fluvisols (Arenic) at floodplain site GP7. Identified parent material is marked according to Fig. 8.
GP7 was excavated to a depth of 250 cm (Fig. 8). Below 220 cm, weakly weathered granite is present and forms the basis of the profile. Similar to profile GP3, predominantly charcoal-bearing, sand-dominated sediments (59 %–96 % sand) were deposited above 220 cm. Humic sections (Ahb horizons) are preserved at depths of 135–150, 160–175 and 185–200 cm below the surface (Figs. 8 and 10, Table S1).
GP5 is rather shallow and less than 90 cm deep (Fig. 8). Similar to GP3 and GP7, the stratigraphy shows charcoal-bearing brown sands (10YR 3/2 to 4/3) in its upper section. They are relatively loose and overlie rounded gravels and blocks exposed below 60 cm. Due to its stratigraphical similarity to GP3 and GP7, as well as comparably low depth, GP5 was only investigated in the field and not sampled for further laboratory analysis.
Due to the thickness of the fluvial deposits (Fluvisols), potential rootability for plants and available water-storage capacity are very good at sites GP3 and GP7 and are respectively moderate to good at site GP5 (Fig. 3). The nutrient situation of GP3 and GP7 is evaluated as moderate (Table S1, Figs. 9 and 10), and once more only P2O5 shows relatively low values (median = 7.5 mg kg−1, SD = 9.4, n = 22). The pH values range between 4.9 and 6.5 (median = 5.5, SD = 0.5, n = 22). Nt content is low again (median = 0.05 %, SD = 0.13, n = 22), and SOM shows average values of 0.83 % (SD = 1.1, n = 22).
The 14C dating results from GP3 and GP7 show modern ages for all eight charcoal samples with an oldest age of 290 ± 134 cal BP (Fig. 8, Table 1).
5.1 Land use and its potential in granite landscape of the Munigua region
Catenary soil mapping in the surroundings of the Roman house complex Casa de Alcántara 1–3 indicates a heterogeneous soil pattern, with soil types clearly depending on geomorphological position (Fig. 2). Most likely this is due to modifications by past soil erosion and related colluviation, leading to profile shallowing in upper slope sites and the deposition of a colluvial cover in middle to lower slope positions and depressions. Frequent occurrences of embedded bricks, shards and charcoal indicate that mobilization and translocation processes were mainly triggered by anthropogenic factors like settling, clearing and/or local agriculture (Leopold and Völkel, 2007). This is in agreement with other investigations in the western Sierra Morena that highlight erosive processes triggered by accelerated human impact (e.g., Recio Espejo et al., 2002). The 14C dating results of this pilot study (Fig. 2, Table 1) provide evidence for past soil erosion phases in pre-Roman, late Roman and post-Roman times and therefore strongly suggest that farming has recurred within the investigated granite landscape and has been a typical land use strategy over very long periods. This observation chronologically reasonably matches with recent investigations in Spanish river catchments. May et al. (2021) reported on increased fluvial and alluvial deposition during Roman or post-Roman times (after approx. 2100 cal BP) and suggest that this is related to a high anthropogenic impact on the local landscape. Similarly, Faust and Wolf (2017) describe a supply of top soil material to many western Mediterranean floodplains that started about 2.2 kyr ago and seems to be related to anthropogenic influences (e.g., agriculture) especially in the lowest-order catchments. The achieved results of this study support this assumption and therefore refer to larger-scale erosion patterns in the entire region. Along with the in situ evidence for soil erosion triggered by anthropogenic land use activities, this finding furthermore indicates the basic suitability of the granite-derived soils for agricultural purposes. The unexpected fact that no colluvia from Munigua's heyday have been found so far might be related to the limited number of age datings, the applied dating technique and the number of locations investigated in this pilot project. In our opinion it does not necessarily mean that there was no colluviation during this period, and further research is needed for a conclusive assessment on soil erosion and colluviation in the region.
The heterogeneous soil inventory revealed variations in present-day soil agro-potential in the surroundings of Casa de Alcántara 1–3. Truncated Leptosols to moderately deep Cambisols in upper slope positions and flatter ridge areas (Fig. 2) show unfavorable to very unfavorable conditions today (Fig. 3). In particular, the low soil depths of these sites and the sandy soil texture (Table S1) have an unfavorable effect on potential rootability, available water-storage capacity and the nutrient budget. The overall soil agro-potential of these sites can be evaluated as unfavorable to very unfavorable (Fig. 3, Table S1).
In contrast, currently moderate to good soil potential exists in middle to lower slope positions, where multi-layered colluvial deposits almost ubiquitously cover the weathered granite (Fig. 3). Here the potential rootability for cultivated plants ranges from moderate to very good due to increased soil depths at these sites. This also favors available water-storage capacity, which can be evaluated as moderate to good. The nutrient situation is mostly reasonable and would certainly allow the cultivation of agricultural crops. Consequently, the cultivation of cereals, such as emmer, barley or millet, which were part of the Roman diet (Flach, 1990; Gerlach, 2001), as well as vegetables, should definitely be possible at many sites within the regional granite landscape. This assumption is supported by the fact that even today, wild oats grow in this landscape and could also have been cultivated as green fodder or hay in Roman times, if not necessarily for human consumption.
Our observations in the surroundings of the Roman house complex Casa de Alcántara 1–3 therefore do not confirm the aforementioned assumptions that the regional soil conditions (i) are currently unsuitable for extensive agriculture and (ii) generally speak against ancient agricultural use in the region (Schattner, 2019b). Instead, the multiphase deposition of colluvium over longer periods and the moderate to good soil agro-potential rather support archeological assumptions concerning agricultural production (Peña Cervantes, 2010; Teichner and Peña Cervantes, 2012; Krug, 2018) involving fields and gardens, as well as pastures for small animals and trees in the wider surroundings of the town.
However, a challenging question in this context is the extent to which the characteristics of current soils provide information on past soil potential. Extensive studies from the Mediterranean supply clear evidence for soil degradation and decreased soil productivity due to the long-term history of land use (e.g., Redman, 1999; García-Ruiz, 2010; Bellin et al., 2013; García-Ruiz et al., 2013; Dotterweich, 2013). It follows that various areas may have been more productive during Roman times, especially sites in erosion-prone hillslope positions (GP11, GP15) that were evaluated as unfavorable to very unfavorable for the present day. The actual status assigned to the sites thus underestimates the ancient soil agro-potential. Vice versa, it can be assumed that the soil potential in aggradational locations, like flat hillslope positions or slope depressions, has improved. Here, colluviation has led to increased soil thickness, which generally favors potential rootability and available water-storage capacity. However, the 14C-derived chronology provides evidence that colluviation had already taken place, at least in part, before the Roman period (Fig. 2, Table 1), which is why the ancient soil agro-potential in these locations was probably not significantly different to that of the present day. We therefore argue that the prevailing moderate to good soil potential of the granite landscape around Munigua today probably also pertained for antiquity.
Further robust evidence for Roman agriculture was gained in a granite area close to the urban center of Munigua (Fig. 1b). Since this area is located outside the fortified urban area, the withdrawal of water for possible irrigation or livestock seems likely. Less than 5 m away from a Roman well, an enrichment of SOM, P2O5, Pt, K2O and other nutrients (Mg, Ca, Na and K) was detected, especially in the lowermost section of a Roman-dated hortic horizon (Fig. 4, Table S1). We interpret these results as indicating intentional humus or compost fertilization intended to further improve the site and secure yields. In this context, it is assumed that organic, nutrient-enriched waste, settlement residues (e.g., pottery, slag fragments or bricks) and possibly also animal excrements were frequently distributed across the surface after harvesting and mixed into the granitoid slope deposits, leading to the gradual development of the Roman hortic horizon. The lower SOM, phosphate and nutrient contents in the upper part of the hortic horizon (23–46 cm) could be due to multiple causes. On the one hand, the abandonment of the site and the associated lack of phosphate and organically enriched fertilizers could have led to an increased decomposition of SOM and increased phosphate and nutrient consumption by vegetation. On the other hand, it is well-known that larger mammals (e.g., wild boars) and soil organisms impact the physical and chemical properties of soil (e.g., Mohr et al., 2005; Risch et al., 2010; Wirthner et al., 2011). This could have led to a mixing of the substrate in post-Roman times and ultimately to a relative depletion of SOM, phosphate and nutrient concentrations in the upper part of the Roman horizon. Additionally, this mixing process would plausibly explain the incorporation of the modern charcoal at 38 cm (Fig. 4b, Table 1) when this profile section formed the terrain surface.
The greatly enhanced phytolith concentrations within the Roman-dated hortic horizon (Fig. 6a) generally indicate increased plant input and can be plausibly explained by phytolith enrichment through agricultural activities (e.g., Cabanes et al., 2011; Madella and Lancelotti, 2012; Meister et al., 2017). This supports the geochemical interpretation of a Roman hortic soil at site GP2. The observed phytolith assemblages are dominated by Pooids containing some of the major Mediterranean crops, such as barley, wheat or rye (Schiemann, 1948), which could indicate the cultivation of cereals. This is supported by the fact that leaf and stem grass phytoliths within the lower profile section dominate over grass phytoliths formed in the inflorescences or in other plant organs (Fig. 6c). The accumulation of the former could be a consequence of harvesting activities, when only the ears (inflorescences) are collected and the stems and leaves are left on the fields (Flach, 1990; Dietrich et al., 2019; Scherer et al., 2021). It is also conceivable that food crops other than cereals might have been cultivated in this area since, for example, the consumption of vegetables is well documented for the Roman period (Bockisch, 1988; Demandt, 2008). Moreover, in their works on agricultural and horticultural practices, the famous ancient authors Columella (De re rustica) and Palladius (Opus agriculturae) described a crop rotation system in which different cereals and vegetables were grown in successive years on the same fields (Flach, 1990). However, although phytoliths have been documented, for instance, for numerous specimens from the Fabaceae (Leguminosae) family, phytolith production varies substantially among different subfamilies (Cummings, 1992), while other important vegetable plants (e.g., root vegetables) produce no or only small amounts of phytoliths (Piperno, 2006). Phytolith analysis thus provides no indication of whether the area was used exclusively for cereals or whether vegetables were also cultivated.
Summing up, it can be asserted that both geochemical and phytolith analyses suggest an ancient agrarian use of site GP2. The exclusive use of the site for pasturing or feeding seems rather unlikely since the large number of Roman artifacts incorporated into the Roman hortic horizon clearly document invasive soil cultivation in the sense of digging or hoeing. Since water supply is guaranteed by the nearby well, the site offers suitable conditions for irrigated agriculture almost all year round.
5.2 Land use and its potential in the metamorphic landscape of the Munigua region
A contrasting land use evaluation has to be made for soils within metamorphic landscape, Here, mostly shallow and sandy to stony soils occur (Fig. 7, Table S1), strongly limiting potential rootability and resulting in unfavorable to very unfavorable water-storage capacity and nutrient budget (Fig. 3). Thus the present-day soil agro-potential is evaluated as unfavorable to very unfavorable at these metamorphic sites.
In addition to the pedological limitations, geomorphological circumstances render agriculture rather unprofitable in these areas. The slopes in the metamorphic landscape are generally steeper, and cultivation would be more exhausting in this landscape unit (Fig. 1b). Furthermore, limits on water availability prevent the all-season agricultural use of these sites since streams are commonly deeply incised and natural springs are rare here, rendering irrigation of agricultural areas difficult. These pedological and geomorphological characteristics make it unlikely that the metamorphic landscape was used for agriculture even in Roman times. Lastly, this assumption is supported by the fact that thick colluvial deposits clearly indicate land use in the granitic landscape but are absent in the observed metamorphic landscape. If there had been historical agricultural use, such deposits should have been preserved at least in places.
5.3 Land use and its potential in the Tamohoso floodplain
Floodplains are usually known to be fertile areas and have therefore been used for agriculture around the globe for thousands of years (Brown, 1997). The nearby lower Guadalquivir valley, for example, is a settlement chamber that has been continuously used for agriculture since the Copper Age at the latest and remains a preferred area for agricultural production (Nocete et al., 2008; García Sanjuán et al., 2013). It therefore seems likely that the alluvial deposits of the nearby Tamohoso River could also have been a favored area for agriculture during Roman times, contributing to the food supply of Munigua.
The analyses of the floodplain sites mostly show moderate to good soil agro-potential (Fig. 3). In particular, thicknesses > 1 m (Fig. 8, Table S1) favor potential rootability and available water-storage capacity. Additionally, the nutrient budget is moderate, resulting in the floodplain soils having overall moderate to good agrarian potential from today's perspective. Moreover, in addition to the physico-chemical properties, the flat terrain of the alluvial plain and the easy access to water favor the agricultural use of the floodplain sites.
Nevertheless, so far we have no information on the appearance of the Tamohoso floodplain in Roman times. Eight 14C ages from fluvial sequences indicate deposition within an active meander belt in modern times (Fig. 8, Table 1). This might have been caused by intensified modern land use activities in the 19th and 20th centuries CE (Tomás García, 1991), as also seen in the widespread juvenile gully systems within the area (Fig. 1d). Regarding the ancient situation, it seems likely that comparable conditions existed in the Tamohoso floodplain at least locally during the heyday of Munigua since past soil erosion should already have led to sediment transfer into the streams. However, associated sediments were probably eroded and relocated due to contemporary active meander dynamics.
5.4 A pedo-geomorphological perspective on the Roman economy
The economy of small cities in the Roman province of Baetica was enormously diverse but commonly specialized around a certain economic activity (e.g., Blázquez Martinez, 1967; Chic García, 1997; Bowman and Wilson, 2009, 2011; Remesal Rodríguez, 2020). In the case of Munigua, metallurgy was without doubt the main and most profitable economic activity and led to considerable wealth in the region (Schattner, 2019b). However, multi-layered colluvial deposits in the surroundings of Munigua (Sect. 4.1.1) and a preserved Roman hortic Anthrosol (Sect. 4.1.2) provide proper pedo-geomorphological evidence of prehistoric and historic agrarian land use in granite areas around Munigua. This indicates that there was at least a second economic activity. It can thus rather be assumed that Roman cities were economically diversified rather than being dedicated to a single economic activity. Using a pedo-morphological approach, the present study therefore generally confirms the concept of an economic diversification of Roman towns in the province of Baetica and Hispania.
Using a pedo-geomorphological approach, the investigation underlines that the soils around Munigua currently have the potential for at least limited agricultural use in larger areas. The granite landscape and the floodplain areas of the Tamohoso River show conditions that would certainly allow the production of basic agricultural goods, not only today but most likely also in Roman times.
Whereas the existence of tilled floodplain soils during Roman times cannot be proven by this study, clear evidence of agrarian use is detectable in granite areas. Here, profile truncation and multi-layered colluvial deposits indicate multiple soil erosion phases in the surroundings of a Roman rural house complex southeast of the urban center of Munigua. Based on our initial 14C-results, anthropogenic soil erosion and colluviation occurred in pre-Roman, late Roman and post-Roman times, which strongly suggests the long-term agrarian utilization of the area. For a granite area close to Munigua, phytolith and geochemical analyses provide evidence of the existence of a Roman garden, where cereals and vegetables might have been cultivated at least on a small scale. The site was most likely fertilized by humus or compost to improve and secure crop yields, confirming the Roman population's extensive knowledge of agricultural practices, already described by ancient authors like, for instance, Columella or Palladius. Hence, the results of investigations in the granite landscape surrounding Munigua indicate a local cultivation of agricultural products that made an active contribution to the food supply of the area. This seems even more likely if the variety of agricultural equipment (Krug, 2018) and olive oil presses (Peña Cervantes, 2010; Teichner and Peña Cervantes, 2012) excavated in the town are also taken into account. This study thus could not confirm the hypothesis that deficient pedological conditions in the surroundings of Munigua generally prevented the agricultural use of the area during Roman times and that therefore the population was mainly dependent on food imports from the lower Guadalquivir valley (Schattner, 2019b). However, there remain many open questions for future research in the coming years, i.e., concerning the extent and production rates of agricultural activities, the cultivated products, or the adaptation techniques applied to cope with seasonal water deficiencies.
In addition to the site-specific information, the study also provides geoarcheological evidence supporting the concept of an economic diversification of Roman cities in Baetica province and Hispania. Even though metallurgy was without doubt the main and most profitable activity in the Munigua region, the economy was certainly not only dedicated to mining activities. Whereas specialty goods were imported, basic agrarian products (e.g., crops, olive oil, wine) were also part of Munigua's economic portfolio and exemplify the economic diversity of urban settlements in the Roman Baetica province.
Data relating to this paper can be found in the Supplement or are available from the corresponding author upon reasonable request.
The supplement related to this article is available online at: https://doi.org/10.5194/egqsj-71-123-2022-supplement.
AK, NH and TGS organized the project. AK, NH and PM carried out the fieldwork. The concept and structure of the paper were organized by AK and NH. The laboratory work was mainly done by PM, IM and JM. AK took the lead in writing the manuscript, with input from NH, JM and TGS. All authors discussed the results and contributed to the final manuscript.
At least one of the (co-)authors is a member of the editorial board of E&G Quaternary Science Journal. The peer-review process was guided by an independent editor, and the authors also have no other competing interests to declare.
Publisher’s note: Copernicus Publications remains neutral with regard to jurisdictional claims in published maps and institutional affiliations.
This article is part of the special issue “Quaternary research from and inspired by the first virtual DEUQUA conference”. It is a result of the vDEUQUA2021 online conference in September/October 2021.
The authors are very grateful to Heinrich Thiemeyer, Doris Bergmann-Dörr and Dagmar Schneider (all Goethe University Frankfurt) for the conscientious implementation of selected soil analyses. Furthermore, Katharine Thomas is acknowledged for improving the language of the manuscript.
This research was financially supported by the Madrid Department of the German Archaeological Institute. This publication was supported by the open-access publication fund of the University of Wuerzburg.
This paper was edited by Tobias Sprafke and reviewed by two anonymous referees.
Ad-hoc-AG Boden: Bodenkundliche Kartieranleitung, 5th edn., Schweizerbart, Stuttgart, 438 pp., ISBN 9783510959204, 2005.
Albert, R. M.: Study of ash layers through phytolith analyses from the Middle Paleolithic levels of Kebara and Tabun caves, Universitat de Barcelona, Barcelona, ISBN 9788459244265, 2000.
Albert, R. M. and Weiner, S.: Study of phytoliths in prehistoric ash layers using a quantitative approach, in: Phytoliths, Applications in Earth Sciences and Human History, edited by: Meunier, J. D. and Colin, F., A.A. Balkema Publishers, Lisse, 251–266, ISBN 9789058093455, 2001.
Albert, R. M., Lavi, O., Estroff, L., Weiner, S., Tsatskin, A., Ronen, A., and Lev-Yadun, S.: Mode of occupation of Tabun Cave, Mt Carmel, Israel during the Mousterian Period: a study of the sediments and phytoliths, J. Archaeol. Sci., 26, 1249–1260, https://doi.org/10.1006/jasc.1999.0355, 1999.
Amelung, W., Blume, H.-P., Fleige, H., Horn, R., Kandeler, E., Kögel-Knabner, I., Kretzschmar, R., Stahr, K., and Wilke, B.-M.: Scheffer/Schachtschabel: Lehrbuch der Bodenkunde, 17th edn., Springer Spektrum, Berlin, Heidelberg, 750 pp., https://doi.org/10.1007/978-3-662-55871-3, 2018.
Bellin, N., Vanacker, V., and De Baets, S.: Anthropogenic and climatic impact on Holocene sediment dynamics in SE Spain: A review, Quatern. Int., 308, 112–129, https://doi.org/10.1016/j.quaint.2013.03.015, 2013.
Blázquez Martínez, J. M.: Estructura económica de la Bética al final de la República romana y a comienzos del Imperio (años 72 a.C.–100 d.C.), Hispania, 27, 7–62, 1967.
Bleck, R.-D.: Zur Durchführung der Phosphatmethode, Ausgrabungen und Funde, 10, 213–218, 1965.
Blume, H.-P., Stahr, K., and Leinweber, P.: Bodenkundliches Praktikum, 3rd edn., Springer Spektrum, Berlin, Heidelberg 255 pp., https://doi.org/10.1007/978-3-8274-2733-5, 2011.
Bockisch, G.: Essen und Trinken im alten Rom, Das Altertum, 34, 87–95, 1988.
Boessneck, J. and von den Driesch, A.: Knochenfunde aus dem römischen Munigua (Mulva), Sierra Morena. Studien über frühe Knochenfunde von der Iberischen Halbinsel, München Uni-Druck, 160–193, 1980.
Borden, W. R., Baillie, I. C., and Hallett, I. C.: The East African contribution to the formalisation of the soil catena concept, Catena, 185, 104291, https://doi.org/10.1016/j.catena.2019.104291, 2020.
Bowman, A. and Wilson, A.: Quantifying the Roman Economy: Methods and Approaches (Oxford Studies on the Roman Economy 1), Oxford University Press, Oxford, 376 pp., https://doi.org/10.1093/acprof:oso/9780199562596.001.0001, 2009.
Bowman, A. and Wilson, A.: Settlement, Urbanization, and Population, Oxford University Press, Oxford, 362 pp., https://doi.org/10.1093/acprof:oso/9780199602353.001.0001, 2011.
Brown, A. G.: Alluvial Geoarchaeology. Floodplain Archaeology and Environmental Change, Cambridge University Press, Cambridge, 377 pp., https://doi.org/10.1017/CBO9780511607820, 1997.
Brown, D. A.: Prospects and limits of a phytolith key for grasses in the central United States, J. Archaeol. Sci., 11, 345–368, https://doi.org/10.1016/0305-4403(84)90016-5, 1984.
Cabanes, D., Weiner, S., and Shahack-Gross, R.: Stability of phytoliths in the archaeological record: a dissolution study of modern and fossil phytoliths, J. Archaeol. Sci., 38, 2480–2490, https://doi.org/10.1016/j.jas.2011.05.020, 2011.
Chic García, G.: Historia económica de la. Bética en la época de Augusto, Padilla Libros, Sevilla, ISBN 84-89769-18-4, 1997.
Cummings, L. S.: Illustrated phytoliths from assorted food plants, in: Phytolith Systematics: Emerging Issues, Advances in Archaeological and Museum Science, edited by: Rapp Jr., G. and Mulholland, S. C., Springer Science & Business Media, 175–192, ISBN 978-1489911575, 1992.
Demandt, A.: Geschichte der Spätantike: Das Römische Reich von Diocletian bis Justinian 284–565 n. Chr., 2nd edn., C. H. Beck, München, 604 pp., ISBN 978-3-406-70032-3, 2008.
de Vries, H. and Barendsen, G. W.: Measurements of age by the carbon-14 technique, Nature, 174, 1138–1141, 1954.
Dietrich, L., Meister, J., Dietrich, O., Notroff, J., Kiep, J., Heeb, J., Beuger, A., and Schütt, B.: Cereal processing at Early Neolithic Göbekli Tepe, southeastern Turkey, PLOS ONE, 14, e0215214, https://doi.org/10.1371/journal.pone.0215214, 2019.
DIN ISO 11277: Soil Quality – Determination of particle size distribution in mineral soil material – method by sieving and sedimentation, Beuth, Berlin, 2002.
Dotterweich, M.: The history of soil erosion and fluvial deposits in small catchments of Central Europe: deciphering the long-term interaction between humans and the environment – a review, Geomorphology, 101, 192–208, https://doi.org/10.1016/j.geomorph.2008.05.023, 2008.
Dotterweich, M.: The history of human-induced soil erosion: Geomorphic legacies, early descriptions and research, and the development of soil conservation – A global synopsis, Geomorphology, 201, 1–34, https://doi.org/10.1016/j.geomorph.2013.07.021, 2013.
Eger, C.: Frühislamische Bestattungen in Munigua (Prov. Sevilla/E), Archäologisches Korrespondenzblatt, 46, 255–269, 2016.
Evans, D. M. and Hartemink, A. E.: Terra Rossa catenas in Wisconsin, USA, Catena, 123, 148–152, https://doi.org/10.1016/j.catena.2014.07.007, 2014.
Faust, D. and Wolf, D.: Interpreting drivers of change in fluvial archives of the Western Mediterranean – A critical view, Earth-Sci. Rev., 174, 53–83, https://doi.org/10.1016/j.earscirev.2017.09.011, 2017.
Flach, D.: Römische Agrargeschichte, C. H. Beck, München, 347 pp., ISBN 978-3-406-33989-9, 1990.
Frey, W. and Lösch, R.: Geobotanik. Pflanze und Vegetation in Raum und Zeit, 3rd edn., Springer Spektrum, Berlin, Heidelberg, 638 pp., https://doi.org/10.1007/978-3-8274-2336-8, 2010.
García-Ruiz, J. M.: The effects of land use on soil erosion in Spain. A review, Catena, 81, 1–11, https://doi.org/10.1016/j.catena.2010.01.001, 2010.
García-Ruiz, J. M., Nadal-Romero, E., Lana-Renault, N., and Beguería, S.: Erosion in Mediterranean landscapes. Changes and future challenges, Geomorphology, 198, 20–36, https://doi.org/10.1016/j.geomorph.2013.05.023, 2013.
García Sanjuán, L., Luciañez Triviño, M., Schuhmacher, T., Wheatley, D., and Banerjee, A.: Ivory Craftsmanship, Trade and Social Significance in the Southern Iberian Copper Age: The Evidence from the PP4-Montelirio Sector of Valencina de la Concepción (Seville, Spain), Eur. J. Archaeol., 16, 610–635, https://doi.org/10.1179/1461957113Y.0000000037, 2013.
Gerlach, G.: Zu Tisch bei den alten Römern: Eine Kulturgeschichte des Essens und Trinkens, Konrad Theiss, Stuttgart, 112 pp., ISBN 978-3806213539, 2001.
Giner-Robles, J. L., Bardají, T., Rodríguez-Pascua, M. A., Silva, P. G., Roquero, E., Elez, J., Perucha, M. A., Baena, R., Guerrero, I., Fernández-Caro, J. J., Pérez-López, R., and Rodríguez-Escudero, E: Análisis arqueosismológico del conjunto arqueológico romano de Mulva- Munigua (Sevilla, España), Geotemas, 16, 605–608, 2016.
Gómez-Zotano, J., Alcántara-Manzanares, J., Olmedo-Cobo, J.-A., and Martínez-Ibarra, E.: La sistematiza-cíon del clima mediterráneo: identificacíon. Clasificacíon y caracterizacíon climática de Andalucía (España), Rev. Geogr. Norte Gd., 61, 161–180, https://doi.org/10.4067/S0718-34022015000200009, 2015.
Grünhagen, W.: Die Ausgrabungen des Terrassenheiligtums von Munigua, in: Neue deutsche Ausgrabungen im Mittelmeergebiet und im Vorderen Orient, edited by: Boehringer, E., 329–343, 1959.
Gutiérrez-Rodríguez, M., Goldberg, P., Martín Peinado, F. J., Schattner, T. G., Martini, W., Orfila, M., and Bashore Acero, C.: Melting, bathing and melting again. Urban transformation processes of the Roman city of Munigua: the public thermae, Archaeol. Anthropol. Sci., 11, 51–67, https://doi.org/10.1007/s12520-017-0527-0, 2019.
Hajdas, I.: Radiocarbon dating and its applications in Quaternary studies, E&G Quaternary Sci. J., 57, 2–24, https://doi.org/10.3285/eg.57.1-2.1, 2008.
Hanel, N.: Römische Öl- und Weinproduktion auf der Iberischen Halbinsel am Beispiel von Munigua und Milreu, Madrider Mitteilungen, 30, 205–238, 1989.
Holliday, V. T. and Gartner, W. G.: Methods of soil P analysis in archaeology, J. Archaeol. Sci., 34, 301–333, https://doi.org/10.1016/j.jas.2006.05.004, 2007.
IUSS Working Group WRB: World Reference Base for Soil Resources 2014, update 2015. International soil classification system for naming soils and creating legends for soil maps, World Soil Resources Reports No. 106, FAO, Rome, ISBN 978-92-5-108369-7, 2015.
Jahn, R., Blume, H.-P., Asio, V. B., Spaargaren, O., and Schad, P.: Guidelines for soil description, FAO, Rome, 97 pp., ISBN 92-5-105521-1, 2006.
Joffre, R., Vacher, J., del los Llanos, C., and Long, G.: The Dehesa: an agrosilvopastoral system of the Mediterranean region with special reference to the Sierra Morena area of Spain, Agroforest Syst., 6, 71–96, https://doi.org/10.1007/BF02220110, 1988.
Keay, S. and Earl, G.: Towns and Territories in Roman Baetica, in: Settlement, Urbanization, and Population, edited by: Bowman, A., and Wilson, A., Oxford University Press, 276–316, https://doi.org/10.1093/acprof:oso/9780199602353.001.0001, 2011.
Kittel, P.: Slope deposits as an indicator of anthropopressure in the light of research in Central Poland, Quatern. Int., 324, 34–55, https://doi.org/10.1016/j.quaint.2013.07.021, 2014.
Krug, A.: Mulva VI: Die Kleinfunde/ Los hallazgos menores, Madrider Beiträge, 36, 436 pp., ISBN 978-3954902378, 2018.
Leopold, M. and Völkel, J.: Colluvium: definition, differentiation, and possible suitability for reconstructing Holocene climate data, Quatern. Int., 162–163, 133–140, https://doi.org/10.1016/j.quaint.2006.10.030, 2007.
Madella, M. and Lancelotti, C.: Taphonomy and phytoliths: A user manual, Quatern. Int., 275, 76–83, doi10.1016/j.quaint.2011.09.008, 2012.
Madella, M., Alexandre, A., and Ball, T.: International Code for Phytolith Nomenclature 1.0, Ann. Bot., 96, 253–260, https://doi.org/10.1093/aob/mci172, 2005.
May, S. M., Norpoth, M., Pint, A. Shumilovskikh, L., Raith, K., Brill, D., Rixhon, G., Moret, P. Jiménez-Vialás, H., Grau-Mira, I., García-Jiménez, I., Marzoli, D., León-Martín, C., Reicherter, K., and Brückner, H.: Mid- to late Holocene environmental changes and human-environment interactions in the surroundings of La Silla del Papa, SW Spain, Geoarchaeology, 36, 573–600, https://doi.org/10.1002/gea.21846, 2021.
Meister, J., Krause, J., Müller-Neuhof, B., Portillo, M., Reimann, T., and Schütt, B.: Desert agricultural systems at EBA Jawa (Jordan): Integrating archaeological and paleoenvironmental records, Quatern. Int., 434, 33–50, https://doi.org/10.1016/j.quaint.2015.12.086, 2017.
Meyer, C., Ullrich, B., and Barlieb C. D. M.: Archaeological Questions and Geophysical Solutions: Ground-Penetrating Radar and Induced Polarization Investigations in Munigua, Spain, Archaeol. Prospect., 14, 202–212, https://doi.org/10.1002/arp.314, 2007.
Mohr, D., Cohnstaedt, L. D., and Topp, W.: Wild boar and red deer affect soil nutrients and soil biota in steep oak stands of the Eifel, Soil Biol. Biochem., 37, 693–700, https://doi.org/10.1016/j.soilbio.2004.10.002, 2005.
Mulholland, S. C. and Rapp Jr., G.: A morphological classification of grass silica-bodies, in: Phytolith Systematics: Emerging Issues, Advances in Archaeological and Museum Science, edited by: Rapp Jr., G. and Mulholland, S. C., Springer Science & Business Media, 65–89, ISBN 978-1489911575, 1992.
Neumann, K., Strömberg, C. A. E., Ball, T., Albert, R. M., Vrydaghs, L., and Cummings, L. S.: International code for phytolith nomenclature (ICPN) 2.0, Ann. Bot., 124, 189–199, https://doi.org/10.1093/aob/mcz064, 2019.
Nocete, F., Queipo, G., Sáez, R., Nieto, J. M., Inácio, N., Bayona, M. R., Peramo, A., Vargas, J. M., Cruz-Auñón, R., Gil-Ibarguchi, J. I., and Santos, J. F.: The Smelting Quarter of Valencina de la Concepción (Seville, Spain): The Specialised Copper Industry in a Political Centre of the Guadalquivir Valley During the Third Millennium BC (2750–2500 BC), J. Archaeol. Sci., 35, 717–732, https://doi.org/10.1016/j.jas.2007.05.019, 2008.
Nocete, F., Sáez, R., Bayona, M. R., Peramo, A., Inacio, N., and Abril, D.: Direct chronometry (14C AMS) of the earliest copper metallurgy in the Guadalquivir Basin (Spain) during the third millennium BC: first regional database, J. Archaeol. Sci., 38, 3278–3295, https://doi.org/10.1016/j.jas.2011.07.008, 2011.
Nuñez, M. A. and Recio, J. M.: Holocene pedoenvironmental situations in the eastern Sierra Morena region (Andújar, Spain), Quatern. Int., 93–94, 191–195, https://doi.org/10.1016/S1040-6182(02)00017-4, 2002.
Paneque, G. and Bellinfante, N.: Mediterranean brown forest soils of the Sierra Morena (Spain), their micromorphology and petrography, in: Soil Micromorphology, edited by: Jongerius, A., 189–200, ISBN 978-0444403254, 1964.
Peña Cervantes, Y.: Torcularia: la producción de vino y aceite en Hispania, Tarragona: ICAC, Institut Català d'Arqueologia Clàssica, 284 pp., ISBN 978-84-937734-1-0, 2010.
Piperno, D. R.: Phytoliths: A Comprehensive Guide for Archaeologists and Paleoecologists, Rowman Altamira, Lanham, New York, Toronto, Oxford, 238 pp., https://doi.org/10.1017/S0016756807003159, 2006.
Portillo, M., Kadowaki, S., Nishiaki, Y., and Albert, R. M.: Early Neolithic household behavior at Tell Seker al-Aheimar (Upper Khabur, Syria): a comparison to ethnoarchaeological study of phytoliths and dung spherulites, J. Archaeol. Sci., 42, 107–118, https://doi.org/10.1016/j.jas.2013.10.038, 2014.
Recio Espejo, J. M., Faust, D., and Nuñez Granados, M. A.: The origin of the Sierra de Aracena Hollows in the Sierra Morena, Huelva, Andalucia, Spain, Geomorphology, 45, 197–209, https://doi.org/10.1016/S0169-555X(01)00154-4, 2002.
Redman C. L.: Human impact on ancient environments, University of Arizona Press, Tucson, 256 pp., ISBN 978-0816519637, 1999.
Reimer, P., Bard, E., Bayliss, A., Beck, J. W., Blackwell, P. G., Ramsey, C. B., Buck, C. E., Cheng, H., Edwards, R. L., Friedrich, M., Grootes, P. M., Guilderson, T. P., Haflidason H., Hajdas, I., Hatte, C., Heaton, T. J., Hoffmann, D. L., Hogg, A. G., Hughen, K. A., Kaiser, K. F., Kromer, B., Manning, S. W., Niu, M., Reimer, R. W., Richards, D. A., Scott, E. M., Southon, J. R., Staff, R. A., Turney, C. S. M., and van der Plicht, J.: IntCal13 and Marine13 radiocarbon age calibration curves 0–50 000 years cal BP, Radiocarbon, 55, 1869–1887, https://doi.org/10.2458/azu_js_rc.55.16947, 2013.
Remesal Rodríguez, J.: Die politische und wirtschaftliche Entwicklung der Baetica in der Kaiserzeit, Madrider Mitteilungen, 61, 115–132, 2020.
Ribeiro, A., Munhá, J., Fonseca, P. E., Araújo, A., Pedro, J.C., Mateus, A., Tassinari, C., Machado, G., and Jesus, A.: Variscan ophiolite belts in the Ossa-Morena Zone (Southwest Iberia): Geological characterization and geodynamic significance, Gondwana Res., 17, 408–421, https://doi.org/10.1016/j.gr.2009.09.005, 2010.
Risch, A. C., Wirthner, S., Busse M. D., Page-Dumroese, D. S., and Schütz M.: Grubbing by wild boars (Sus scrofa) and its impact on hardwood forest soil carbon dioxide emissions in Switzerland, Oecologia, 164, 773–784, https://doi.org/10.1007/s00442-010-1665-6, 2010.
Rodríguez-Pascua, M. A., Pérez-López, R., Silva, P. G., Giner-Robles, J. L., Garduño-Monroy, V. H., and Reicherter, K.: A comprehensive classification of Earthquake Archaeological Effects (EAE) for archaeoseismology: application to ancient remains of Roman and Mesoamerican cultures, Quatern. Int., 242, 20–30, https://doi.org/10.1016/j.quaint.2011.04.044, 2011.
Ruiz-Bueno, M. D.: Actividad sísmica en el mediodía ibérico durante el siglo III d.C. La incidencia arqueológica en Corduba (Córdoba), Pyrenae, 48, 29–51, https://doi.org/10.1344/Pyrenae2017.vol48num2.2, 2017.
Sanz, T., Sampelayo, H., and Ledesma Garcia, F.: Mapa Geológica de España. Escala 1 : 50.000, edited by: Instituto geológico y minero de España (IGME), 941, 13–38, 1973.
Schattner, T. G.: Munigua: Cuarenta años de investigaciones, Sevilla: Junta de Andalucía, Instituto Arqueológico Alemán, 335 pp., ISBN 84-8266-364-X, 2003.
Schattner, T. G.: Die Wiederentdeckung von Munigua: Abriss der Forschungsgeschichte, Madrider Mitteilungen, 46, 267–288, 2005.
Schattner, T. G.: Antike “Mondlandschaften”, Archäologie Weltweit, 7, 26–31, 2019a.
Schattner, T. G.: Munigua: Un recorrido por la arqueología del Municipium Flavium Muniguense, Editorial Universidad de Sevilla-Secretariado de Publicaciones, Sevilla, 225 pp., ISBN 978-8447219179, 2019b.
Schattner, T. G., Ovejero Zappino, G., and Pérez Macías, J. A.: Avances sobre la producción metalúrgica en Munigua, Habis, 36, 253–276, 2005.
Scherer, S., Höpfer, B., Deckers, K., Fischer, E., Fuchs, M., Kandeler, E., Lechterbeck, J., Lehndorff, E., Lomax, J., Marhan, S., Marinova, E., Meister, J., Poll, C., Rahimova, H., Rösch, M., Wroth, K., Zastrow, J., Knopf, T., Scholten, T., and Kühn, P.: Middle Bronze Age land use practices in the northwestern Alpine foreland – a multi-proxy study of colluvial deposits, archaeological features and peat bogs, SOIL, 7, 269–304, https://doi.org/10.5194/soil-7-269-2021, 2021.
Schiemann, E.: Weizen, Roggen, Gerste. Systematik, Geschichte und Verwendung, Gustav Fischer Verlag, Jena, 102 pp., 1948.
Schüller, H.: Die CAL-Methode, eine neue Methode zur Bestimmung des pflanzenverfügbaren Phosphates in Böden, Zeitschrift für Pflanzenernährung, Düngung und Bodenkunde, 123, 48–63, 1969.
Semmel, A.: Grundzüge der Bodengeographie, Teubner, Stuttgart, 101 pp., ISBN 978-3519134084, 1977.
Strauss, G. K. and Madel, J.: Geology of massive sulphide deposits in the Spanish-Portuguese Pyrite Belt, Geol. Rundsch., 63, 191–211, 1974.
Teichner, F.: Spuren islamischer Besiedlung auf dem Castillo de Mulva (Villanueva del Río y Minas, prov. Sevilla)?, Madrider Mitteilungen, 39, 336–355, 1998.
Teichner, F. and Peña Cervantes, Y.: Archäologisches zur Herstellung von Olivenöl und Wein im römischen Hispanien, Bonner Jahrbücher, 210/211, 375–458, 2012.
Tomás García, L. J.: La Minería sevillana del carbón. Minas de La Reunión y la Compañía de los Ferrocarriles de M.Z.A, Diputación Provincial de Sevilla, Sevilla, ISBN 84-7798-059-4, 1991.
Tornos, F., Inverno, C. M. C., Casquet, C., Mateus, A., Ortiz, G., and Olivera, V.: The metallogenic evolution of the Ossa-Morena Zone, J. Iber. Geol., 30, 143–181, 2004.
Tsartsidou, G., Lev-Yadun, S., Albert, R.-M., Miller-Rosen, A., Efstratiou, N., and Weiner, S.: The phytolith archaeological record: strengths and weaknesses evaluated based on a quantitative modern reference collection from Greece, J. Archaeol. Sci., 34, 1262–1275, https://doi.org/10.1016/j.jas.2006.10.017, 2007.
Twiss, P. C., Suess, E., and Smith, R. M.: Morphological classification of grass phytoliths, Soil Sci. Soc. Am. Pro., 33, 109–115, https://doi.org/10.2136/sssaj1969.03615995003300010030x, 1969.
Ullrich, B., Meyer, C., and Weller, A.: Geoelektrik und Georadar in der archäologischen Forschung: geophysikalische 3D-Untersuchungen in Munigua (Spanien), in: Einführung in die Archäometrie, edited by: Wagner, G. A., Springer, 76–93, https://doi.org/10.1007/978-3-540-71937-3, 2007.
Weihrauch, C.: Phosphor-Dynamiken in Böden. Grundlagen, Konzepte und Untersuchungen zur räumlichen Verteilung des Nährstoffs, Springer Spektrum, Wiesbaden, 369 pp., https://doi.org/10.1007/978-3-658-22348-9, 2018.
Wirthner, S., Frey, B., Busse, M. D., Schütz M., and Risch A. C.: Effects of wild boar (Sus scrofa) rooting on the bacterial community structure in mixed-hardwood forest soils in Switzerland, Eur. J. Soil Biol., 47, 296–302, https://doi.org/10.1016/j.ejsobi.2011.07.003, 2011.
Zádorová, T. and Penížek, V.: Formation, morphology and classification of colluvial soils: A review, Eur. J. Soil Sci., 69, 577–591, https://doi.org/10.1111/ejss.12673 2018.
Zazo, C., Dabrio, C. J., Goy, J. L., Lario, J., Cabero, A., Silva, P. G., Bardají, T., Mercier, N., Borja, F., and Roquero, E.: The coastal archives of the last 15ka in the Atlantic–Mediterranean Spanish linkage area: Sea level and climate changes, Quatern. Int., 181, 72–87, https://doi.org/10.1016/j.quaint.2007.05.021, 2008.