the Creative Commons Attribution 4.0 License.
the Creative Commons Attribution 4.0 License.
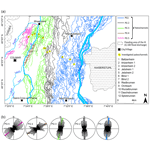
Morpho-sedimentary characteristics of Holocene paleochannels in the Upper Rhine alluvial plain, France
Stoil Chapkanski
Damien Ertlen
Haider Mahmood
Edward Obioha
Frank Preusser
Claire Rambeau
Ferréol Salomon
Marco Schiemann
Laurent Schmitt
Abdulkarim, M., Chapkanski, S., Ertlen, D., Mahmood, H., Obioha, E., Preusser, F., Rambeau, C., Salomon, F., Schiemann, M., and Schmitt, L.: Morpho-sedimentary characteristics of Holocene paleochannels in the Upper Rhine alluvial plain, France, E&G Quaternary Sci. J., 71, 191–212, https://doi.org/10.5194/egqsj-71-191-2022, 2022.
The French Upper Rhine alluvial plain is characterized by a complex system of paleochannels inherited from Late Glacial to Holocene fluvial dynamics of the Rhine and Ill river systems, among other smaller rivers. These paleochannels represent valuable archives for understanding and reconstructing the fluvial and landscape evolution of the area. However, the Holocene temporal trajectories of the paleochannels, in response to a range of environmental changes, remain poorly understood. This study presents a detailed and systematic mapping and characterization of an extensive network of paleochannels spanning the entire width (19 km) of a reach of the central French Rhine plain. Based on qualitative and quantitative lidar analysis, field investigations, and provenance investigations of paleochannel infill sequences, five distinct paleochannel groups (PG 1 to PG 5) were identified in the study area. These paleochannel groups differ considerably regarding their channel patterns, morphological characteristics, and sedimentary sources of the infilling sediments. The interpretation of the different datasets indicates that the development of these different paleomorphologies is attributed to significant changes in hydro-geomorphodynamic processes in the area during the Holocene, especially lateral migrations of the Rhine and Ill rivers. The findings reported here are promising and will have significant implications for reconstructing the long-term (Late Glacial to Holocene) evolution of the Upper Rhine fluvial hydrosystem in response to various controlling factors.
Die französische Oberrheinebene ist durch ein komplexes System von ehemaligen Fließrinnen gekennzeichnet, die aus der spätglazialen bis holozänen fluvialen Dynamik des Rhein- und Ill-Systems sowie einiger anderer kleinerer Flüsse stammen. Diese Fließrinnen stellen wertvolle Archive dar, die für das Verständnis und die Rekonstruktion der fluvialen und landschaftlichen Entwicklung des Gebietes von zentraler Bedeutung sind. Die zeitliche Entwicklung der Fließrinnen im Holozän, als Reaktion auf die Veränderung einer Reihe von Umweltbedingungen, ist jedoch nach wie vor nur unzureichend bekannt. Diese Studie präsentiert eine detaillierte und systematische Kartierung und Charakterisierung eines ausgedehnten Netzwerks von ehemaligen Fließrinnen, das sich über die gesamte Breite (19 km) eines Abschnitts der zentralen französischen Rheinebene erstreckt. Auf Grundlage von qualitativen und quantitativen lidar-Analysen, sowie durch Feld- und Provenienzuntersuchungen von Sedimentfüllungen wurden im Untersuchungsgebiet fünf verschiedene Rinnengruppen (PG 1 bis PG 5) identifiziert. Diese Gruppen unterscheiden sich erheblich in Bezug auf ihre Rinnenmuster, morphologischen Merkmale und Sedimentherkunft. Die Interpretation der verschiedenen Datensätze deutet darauf hin, dass die Entwicklung dieser unterschiedlichen Paläo-Morphologien auf signifikante Veränderungen der hydro-geomorphodynamischen Prozesse in diesem Gebiet während des Holozäns zurückzuführen ist, insbesondere auf die seitlichen Verlagerungen von Rhein und Ill. Die hier vorgestellten Ergebnisse sind vielversprechend und bilden die Basis für eine Rekonstruktion der langfristigen (spätglazialen bis holozänen) Entwicklung des fluvialen Oberrhein-Hydrosystems in Abhängigkeit von verschiedenen Einflussfaktoren.
- Article
(13574 KB) -
Supplement
(14690 KB) - BibTeX
- EndNote
The French Upper Rhine alluvial plain (eastern France) is characterized by a complex pattern of paleochannels inherited from the Late Glacial to Holocene fluvial dynamics of the Rhine and Ill rivers, as well as some other tributaries or sub-tributaries of the Rhine (Hirth, 1971; Carbiener, 1983a; Striedter, 1988). From Colmar to Sélestat, which is a part of the so-called “Ried central d'Alsace” (Carbiener, 1983a), the formation and evolution of the paleochannel network is attributed to significant landscape changes throughout the Holocene, as exemplified by the narrowing and eastward lateral migration of the Rhine fluvial hydrosystem and a westward displacement for its main tributary, the Ill River (Schmitt et al., 2016). Consequently, these paleochannels inherited from these lateral displacements preserve a comprehensive record of Holocene environmental changes, which is invaluable for understanding past landscape evolution and fluvial processes, as well as providing insights into the factors that controlled these changes (Bowler, 1978; Page et al., 1996; Dambeck and Thiemeyer, 2002; Sylvia and Galloway, 2006; Kemp and Spooner, 2007; Bisson et al., 2011; Nandini et al., 2013; Resmi et al., 2017). However, both the internal structure and organization of the extensive and well-preserved network of paleochannels, as well as the temporal development of trajectories in response to environmental change during the Holocene remain poorly understood.
Around the world, paleochannels have been extensively studied using a variety of methodological approaches in order to reconstruct the evolution of fluvial hydrosystems and associated landscapes in the geological and historical past (e.g., Page et al., 1996; Dambeck and Thiemeyer, 2002; Bos et al., 2008; Rossetti and Góes, 2008; Erkens et al., 2011; Plotzki et al., 2015; Jotheri et al., 2016; Resmi et al., 2017; Scorpio et al., 2018; Candel et al., 2020; Khosravichenar et al., 2020; von Suchodoletz et al., 2022). Likewise, in the Ried central d'Alsace, pioneering studies (e.g., Carbiener, 1969, 1983a; Hirth, 1971; Al Siddik, 1986; Boës et al., 2007; Schmitt et al., 2016) have investigated paleochannels and paleoenvironments with the aim to reconstruct the Holocene evolution of the landscape and/or study past human–river interactions. However, these research efforts lacked precision (e.g., no location of the sampling sites, no provenance study of fine sediments) or were, in many cases, concentrated around some archeological sites located close the village of Mussig (Boës et al., 2007) and focused on a rather limited number of paleochannels between the Rhine and Ill rivers. No study has yet extensively mapped and characterized systematically the paleochannel network over the entire width of the large and complex alluvial plain.
Against this backdrop, the present study aims at providing new insights into the formation and evolution of the paleochannels. The study specifically aims to (1) map the paleochannels and measure their morphometric properties using remote sensing data, (2) characterize paleochannel geometry and stratigraphic infillings of selected paleochannels using hand-augured core data, and (3) determine the provenance of paleochannel infillings through mid-infrared spectroscopic analysis. In addition, as the chosen research tools have barely been used, this study may also provide some methodological outputs.
The study area is located in the French Rhine alluvial plain, within the Upper Rhine Graben (URG). The URG is a 30–40 km wide rift valley extending 300 km from the Jura Mountains to the southern border of the Rhenish Massif (Fig. 1). The valley is bounded to the west by the Vosges and Pfälzerwald (Palatinate Forest) mountains and to the east by the Black Forest and Odenwald mountains. This rift structure ensued from the formation of the Alps starting during the middle Eocene, with the main phase of its rifting occurring during the Oligocene and Miocene, as well as extending into the Pleistocene and Holocene (Przyrowski and Schäfer, 2015). Today, it forms a down-faulted trough through which the Rhine has flown continuously since the start of the Pleistocene (Preusser, 2008). During this time period, the URG was an important sink for sediments from the Rhine River and its tributaries, mostly unconsolidated fluvial sediments of up to several hundreds of meters thickness (e.g., Haimberger et al., 2005; Gabriel et al., 2013). In the French Rhine alluvial plain, the Pleistocene sediments, primarily gravel and sand deposited during glacial periods, intercalate with fine-grain layers (sand, silt, and clay) attributed to warm periods. Hence, the superficial Holocene deposits generally have finer textures than the Pleistocene sediments (Simler et al., 1979), except on the historical Rhine braided belt (Schmitt, 2001; Schmitt et al., 2016).
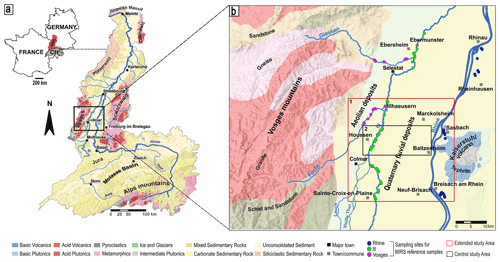
Figure 1(a) Geological setting and location of the Upper Rhine Graben (URG). (b) Location of the two spatial scales of the study area within the French Rhine alluvial plain: (1) the extended study area (red) on which the lidar DEM was studied and (2) the central study area (black) on which the paleochannels were bored by hand auger. The figure also shows the sampling sites for MIRS reference samples. Modified from Chapkanski et al. (2020). Source of data: ESRI, BRGM.
Following the Older Dryas (13.9 ka cal BP; van Raden et al., 2013), the longitudinal profile of the Rhine readjusted in response to several controlling factors: (i) a decrease in water and sediment fluxes due to Holocene climate warming (Hirth, 1971); (ii) trapping of sediment in Swiss lakes (e.g., Lake Constance) for 60 % of the Rhine basin area upstream of Basel after their formation by the retreat of Alpine glaciers, which decreased the sediment load and intensity of floods (Walser, 1959; Hirth, 1971); and (iii) positive tectonic movements (uplift) upstream and downstream of Mulhouse (Nivière et al., 2006; Kock et al., 2009) and negative (downward) tectonic movements around Marckolsheim (Jung and Schlumberger, 1936; Illies and Greiner, 1978) or even over the entire Marckolsheim–Strasbourg sector and north of Strasbourg (Jung and Schlumberger, 1936; Illies and Greiner, 1978; Vogt, 1992).
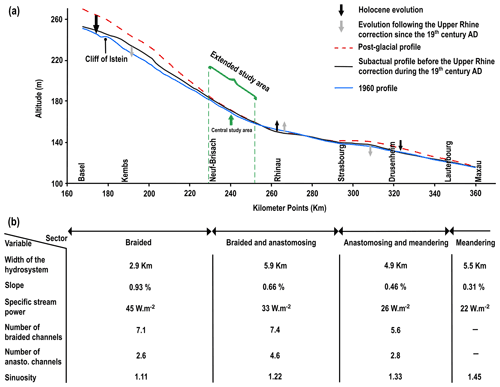
Figure 2(a) Post-glacial and Holocene Upper Rhine longitudinal profiles. (b) Some hydromorphological characteristics according to the Upper Rhine sectorization (adapted from Commission Internationale de l'Hydrologie du Bassin du Rhin, 1977; Carbiener and Dillmann, 1992; Schmitt, 2001; and Schmitt et al., 2019).
Consequently, the longitudinal profile of the Rhine became more incised between the Basel–Neuf-Brisach and Strasbourg–Lauterbourg sections during the Holocene and remained unchanged or even rose slightly in the Neuf-Brisach–Strasbourg section (Carbiener, 1969, 1983a). Thus, the Holocene Upper Rhine and its alluvial plain are classified into four major longitudinal sections based on geomorphological characteristics and dynamics (Carbiener, 1969, 1983a; Schmitt et al., 2016; Fig. 2). The upstream sector (from Basel to Neuf-Brisach) is characterized by a braided channel pattern, while a braided-anastomosing channel pattern characterizes the middle sector, from Neuf-Brisach to Strasbourg. The downstream sector (from Strasbourg to Karlsruhe–Maxau) is distinguished by a combination of anastomoses and incipient meanders, whereas further north, beyond the French Rhine Plain, the Rhine River is characterized by an almost pure meandering style. It has to be noted that the natural channel pattern and dynamics have been completely destroyed by human regulation works (correction, regularization, and canalization) since the 19th century (e.g., Eschbach et al., 2018).
The central study area of our research, within the French Rhine alluvial plain, corresponds roughly to a large transect (14 km) from Houssen to Baltzenheim along which paleochannels were cored (Fig. 1b). To gain insight into the channel pattern of these paleochannels, we studied the surface topography using a light detection and ranging (lidar) digital elevation model (DEM) over a larger spatial scale, which corresponds to an extended studied area (Fig. 1b). The area stretches between the villages of Neuf-Brisach and Sainte-Croix-en-Plaine in the south and Illhaeusern and Marckolsheim in the north, covering an area of approximately 572 km2 (Fig. 1b). This area corresponds mainly to the braided-anastomosing sector and is delimited by the Rhine River in the west and the Ill River in the east. It corresponds also to the southern part of the so-called “Grand Ried central d'Alsace”, which extends up to south of Strasbourg and corresponds to a large, wet alluvial area whose biodiversity is extremely rich (Carbiener, 1983a). The Fecht River represents a major western tributary of the Ill River in this area, which joins the Ill at Illhaeusern. Until the end of the Pleistocene, the Ill River did not exist as the Rhine fluvial hydrosystem (with mostly braided channel pattern) occupied almost the entire width of the plain (Schmitt et al., 2016). At the start of the Holocene, a change in the dynamics and narrowing of the Rhine fluvial hydrosystem resulted in the formation of the Ill River (Schmitt et al., 2016).
The Rhine and Ill rivers then continuously moved laterally across the plain until human interference, with fine sediments deposited on the alluvial plain with an average thickness of 0.5–1 m but reaching several meters in channel structures (Schmitt et al., 2016). As a consequence, the current floodplain features a complex network of paleochannels. These paleochannels are generally embedded into the Rhine gravels, incompletely filled, and are therefore visible as geomorphological features (depressions and levees) in the surface topography. The former channels have been filled with clastic and organic sediments and are mainly bereft of flowing water, although some remain active today due to perennial or intermittent upstream hydrological connection to the Ill or Rhine rivers or to groundwater (Carbiener, 1983a, b; Trémolières et al., 1993; Schmitt, 2001; Schmitt et al., 2016), forming phreatic and semi-phreatic rivers (Carbiener, 1983a, b; Schmitt et al., 2007). These streams support an abundance of biodiversity, in particular aquatic macrophytes (Carbiener, 1983b; Trémolières et al., 1993).
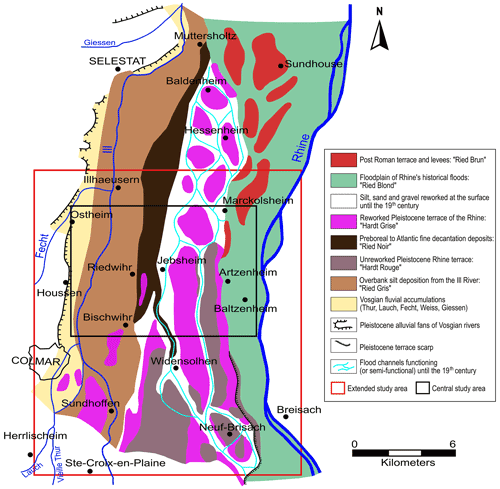
Figure 3Geomorphological map of a part of the French Rhine alluvial plain whose southern part corresponds to our extended study area (Colmar-Sélestat; Hirth, 1971, modified by Schmitt, 2001, and Schmitt et al., 2016). Figure modified from Schmitt et al. (2016).
The alluvial plain is generally categorized into two main geomorphological units based on geomorphological, hydrological, pedological, and ecological properties: the “Hardt” and the “Ried” (Carbiener, 1969; Hirth, 1971; Ollive et al., 2006, Fig. 3). The “Hardt” is an area with gravelly islands associated with the Late Pleistocene alluvial fan of the Rhine, primarily located in the central part of the alluvial plain. The “Ried” comprises low-lying, swampy areas primarily consisting of fine sand, silt, clay, and organic matter, which may be mixed together (Hirth, 1971; Kremer et al., 1978; Al Siddik, 1986; Ollive et al., 2006). These two zones are further subdivided into six subunits: “Hardt Rouge”, “Hardt Grise”, “Ried Blond”, “Ried Brun”, “Ried Noir”, and “Ried Gris” (Hirth, 1971; Kremer et al., 1978, Fig. 3).
The Hardt Rouge represents the intact Pleistocene alluvial fan, whereas the Hardt Grise has been remobilized at the surface. The Ried Blond is the sub-modern floodplain of the Rhine prior to the correction works (width of about 5–7 km), composed of sandy/silty and calcareous alluvium and in some places overlapped by young soils (Kremer et al., 1978). Ried Brun corresponds to a series of moderately overhanging post-Roman Rhine levees and terraces located within the sub-modern floodplain of the Rhine, with limestone-rich soils and highly mineralized organic matter. The Ried Noir, situated further to the west of the plain, corresponds to marshy depressions with permanent or semi-permanent contact to groundwater. It is composed of black hydromorphic soils rich in organic matter and locally peat (Carbiener, 1969, 1983a; Hirth, 1971). The westernmost component, the Ried Gris, represents the area regularly flooded by the Ill River, consisting of fine floodplain sediments, mainly fine sand, silt, and clay (Carbiener, 1969, 1983a; Hirth, 1971; Al Siddik, 1986).
3.1 Remote sensing
Paleochannels within the study area were identified and comprehensively mapped through the combined use of a lidar-derived DEM, a photogrammetric digital terrain model (DTM), aerial photos, and historical maps from the 18th and 19th centuries. The elevation data, aerial photos, and historical maps are found in the Supplement. The primary data used are a lidar-generated DEM with a resolution of 50 cm and pixel values ranging from 132.47 to 556.82 m. However, a portion of the research area (approximately 65 km2) currently lacks lidar coverage (Fig. 4); it is covered by a photogrammetric DTM with a 1 m resolution. The photogrammetric DTM was developed in 2018 and obtained from the RGE ALTI® database of the National Institute of Geographic and Forestry Information, France (IGN-F). In addition to the elevation data, aerial photographs, and historical maps dating from 1702 CE to 1838 CE were geo-referenced and digitized to complement the identification and mapping of paleochannels. Prior to mapping, the datasets were processed, and enhancement techniques (e.g., contrast stretching, hill shading, and slope maps) were applied to improve visualization and enhance the appearance of the microtopography and detectability of the geomorphologic features of interest (paleochannels).
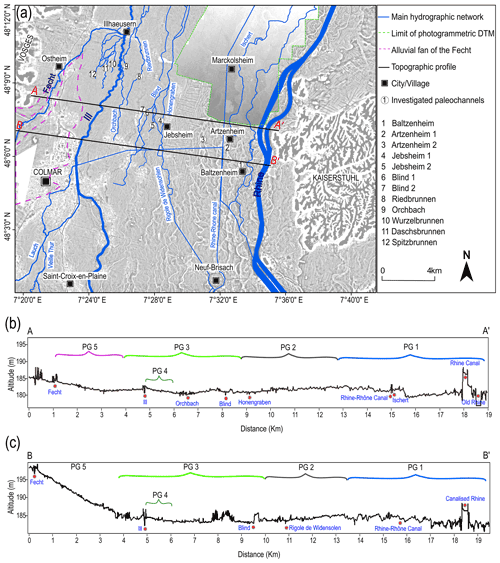
Figure 4(a) Lidar DEM and photogrammetric DTM of the study area, with the main river network and coring sites of the investigated paleochannels. (b) Topographic profile along line A–A′. (c) Topographic profile along line B–B′. Data sources: lidar DEM: Regional Council of Grand Est and the European Collectivity of Alsace; photogrammetric DTM: IGN France.
After their mapping, the paleochannels were further characterized in relation to their surface topographic properties, including channel width, channel depth, width / depth ratio, sinuosity, and orientation (paleochannel direction). For the determination of these geometrical properties, four transect lines were drawn across the study area, perpendicular to the orientation of the main valley (Fig. S3 in the Supplement). Based on the surface topography, paleochannel width and depth were measured at points where they intersect with these profile lines using the Measure tool and 3D Analyst tool in ArcMap 10.4. The sinuosity index (P) of the paleochannels was also estimated using the Measure tool in ArcMap 10.4, following the method of Fuller et al. (2013). All morphometric parameters were determined three times, with the average values reported. Except for the measurements of paleochannel orientations, which were performed using the open-source GIS program QGIS V10.3, all dataset processing, paleochannel mapping, and planform measurements were carried out using ESRI ArcGIS 10.4.1.
3.2 Paleochannel coring
A total of 16 paleochannels within the alluvial plain were originally chosen for this study. The selection strategy was designed to include all paleochannels from the Rhine to the left (western) side of the Ill River (Figs. 4a; 5a). Furthermore, the paleochannels were chosen based on a number of criteria, including the absence of significant anthropogenic disturbances and whether or not they had been previously investigated. Only 12 of the 16 paleochannels could be investigated (Fig. 4) due to inaccessibility or the lack of authorization by landowners. The paleochannels with current activity were given names based on their present streams, while others that are completely abandoned and filled were assigned names reflecting their geographical location. Borings with an Edelman hand auger were used to characterize the subsurface geometry and sedimentary infillings of the selected paleochannels. The cores were placed at irregular (3–5 m) intervals along transects that spanned the entire width of the paleochannels, while the thickness of fine sediment infill determined the depth of the cores. Almost every coring penetrated fine sediment (clay, silt, and sand) and terminated in underlying gravel beds. The gravel deposits were encountered at depths ranging from less than 0.5 m near the channel margins to depths up to 5 m at the deepest portions of some paleochannels. Sediment samples were collected at 20 cm depth intervals and described in terms of dominant grain size, color, and organic matter content (qualitatively). These data were compiled to delineate stratigraphic units filling the paleochannels.
3.3 Provenance analysis
The source region of sediments and the contributions from different drainage basins within the same sedimentary basin can be determined by performing provenance studies (Haughton et al., 1991). The data from these analyses will facilitate a better understanding of fluvial paleodynamics at large spatiotemporal scales (Chapkanski et al., 2020). Following Chapkanski et al. (2020), the provenance of paleochannel infills and basal gravel deposits was determined by combining mid-infrared spectroscopy (MIRS) measurements and discriminant analyses (DAs). MIRS is a molecular-based, non-destructive and rapid method permitting the characterization of both mineralogical and bio-chemical properties of sediments (Bertaux et al., 1998). Therefore, using mid-infrared spectra to feed discriminant analysis allows mineralogical similarities to be established between samples from a sedimentary reference dataset and samples from paleochannel infills (Chapkanski et al., 2020). The reference dataset used in this study contains 196 (sub-)surface samples covering the main potential sedimentary sources in the study area (i.e., Rhine River, Ill River, and Vosges tributaries; Chapkanski et al., 2020; see also Fig. 1b). A total of 46 additional reference samples were collected and incorporated into the original reference dataset. A total of 73 samples from the paleochannel infills (referred to as target samples) were collected in the 12 paleochannels from different stratigraphic units.
Both the reference and target samples were dried for 7 d at 30 ∘C before sieving through a 2 mm sieve. The samples were then ground for 3 min using a vibratory disc mill equipped with an agate grinding set to obtain a fine, homogenous powder. Spectroscopic measurements were performed on a Fourier-transform infrared spectroscopy (FT-IR) frontier spectrometer (PerkinElmer, USA) with KBr beam splitter equipped with a diffuse reflectance sampling accessory. The powdered samples were scanned from 4000 to 450 cm−1 with a 2 cm−1 resolution. Each spectrum is the average of 20 scans of each sample. Following Chapkanski et al. (2020), spectra resolution was averaged over a 16 cm−1 interval to improve spectra pattern recognition. Standard normal variates, baseline, and second derivate pre-treatments were performed to reduce scatter effects and improve the spectral alignment. Moreover, only the fingerprint mid-infrared spectral region (from 1500 to 450 cm−1) was selected prior to statistical treatments in order to reduce misleading results due to organic matter absorbing in the regions between 3750–3450 cm−1 (Bertaux et al., 1998) and 2950–2850 cm−1 (Kaufhold et al., 2012). A stepwise discriminant analysis was applied using the Mahalanobis distance to assess the spectral resemblances between the reference and the target samples. The sedimentary provenance of target samples was deduced as the function of the nearest barycenter of reference groups. Detailed statistical treatments are described by Ertlen et al. (2010) and Chapkanski et al. (2020).
In order to evaluate the effect of organic matter on spectra and thus misleading provenance prediction, samples collected in paleochannels (target samples) were analyzed before and after organic matter removal by hydrogen peroxide (H2O2). The deviation of the Mahalanobis distance of samples before and after organic matter removal was used to assess the effects of organic matter on provenance determination.
4.1 Remote-sensing-based mapping and analysis
We were able to map a detailed and extensive network of paleochannels (about 19 km wide) covering the entire study area by integrating elevation data and aerial images. A map of the area was created (Fig. 5), revealing far more information about the paleochannels than the lidar data provided. The area was divided into five paleochannel groups (PG 1 to PG 5) based on the expected genesis of the paleochannels by showing where the channels are coming from, their location within the alluvial plain, direction, and general channel pattern. Quantitative (lidar) analyses revealed further differences in the surface topographical properties (channel width, depth, sinuosity, and orientation) of these paleochannel groups (Figs. 5 and 6, Table 1).
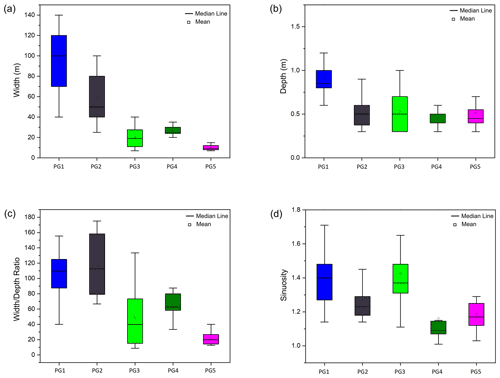
Figure 6Box plot diagrams of four morphometric parameters for the five paleochannel groups. (a) Channel width, (b) channel depth, (c) width / depth ratio, and (d) sinuosity.
Table 1Summary data of four morphometric parameters for the surface topography of the paleochannel groups (PG 1 to PG 5). The values of each morphometric parameter are arithmetic means and variation coefficients (in brackets).
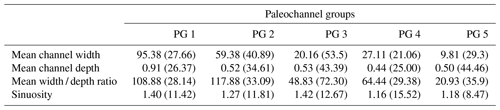
PG 1. The paleochannels span a 7 km wide corridor in the eastern section of the study area, close to the current Rhine fluvial hydrosystem. This group comprises multi-threaded channel networks with a high degree of sinuosity (average 1.40). The paleochannels follow a south-southwest–north-northeast trend and are on average 95 m wide and 1 m deep (surface topography). The majority of these paleochannels are located on the lowest topographical level of the alluvial plain, but there is a general increase in surface elevation moving east to west, with a sharp elevation increase in the center of the group (Fig. 4b).
PG 2. This paleochannel group is located in the central sector of the study area and is generally south-southeast–north-northwest trending. Similar to PG 1, the paleochannels exhibit a multi-threaded channel pattern but are less sinuous (average 1.27) than the paleochannels of PG 1. The paleochannel surface topography exhibits an average width of 60 m and an average depth of 0.5 m. The trace of these paleochannels becomes less visible downstream around the village of Jebsheim due to agricultural activity in the area, which has smoothed the microtopography over time. The PG 2 paleochannels are situated on the same topographical level as the western portion of the PG 1 paleochannels, but the elevation decreases progressively from east to west (Fig. 4b and c).
PG 3. These paleochannels extend approximately 6 km from the central portion of the study area, where they appear to intersect the paleochannels of PG 2, to the western side of the Ill River. The paleochannels exhibit both single- and multi-threaded channel patterns, as well as a high degree of sinuosity (average 1.43). They run south-southwest–north-northeast and are significantly smaller than PG 1 and PG 2 paleochannels, with the channel size decreasing from east to west. Their widths range from less than 10 to 45 m, with an average width of 20 m and a depth of 0.5 m. The PG 3 paleochannels are on a similar topographic level as PG 2, but the elevation increases towards the current channel of the Ill River.
PG 4. The paleochannels are localized very close to the current channel of the Ill River and are associated with the paleochannels of PG 3. Although these paleochannels are associated with the PG 3 paleochannels, they all originate on the Ill River's eastern bank, where they cut into the Ill levee before connecting to the PG 3 paleochannels. In comparison to PG 3 paleochannels, these are low sinuosity (average 1.16), single-thread channels that average 27 m in width and 0.4 m in depth. The PG 4 paleochannels are at a relatively high elevation, which corresponds to the levee of the Ill River. However, there is a progressive decrease in elevation from west to east (Fig. 4b and c). These channels are only visible with lidar. They are barely noticeable in the field or in aerial photos, as they are hidden by dense grassland or in some sectors by the dike of the Ill River.
PG 5. These paleochannels are limited to the northwestern sector of the study area, covering an area of about 2.5 km width. The paleochannels originate on the Fecht River's western bank, from where they flow northeast towards the current channel of the Fecht around Illhaeusern, where they coalesce and gradually taper out (Fig. 5). The paleochannels are mainly multi-threaded to meandering channels (average sinuosity of 1.18) and are much smaller compared to the paleochannels of the other groups. They have an average width of 10 m and a depth of 0.5 m. These paleochannels are located on the highest topographical level of the alluvial plain, which corresponds to the downstream extremity of the alluvial fan of the Fecht River (Fig. 4b and c).
4.2 Paleochannels cross sections, infill sedimentary characteristics, and provenance
The geometry and internal architecture of the investigated paleochannels vary significantly across the study area. The paleochannels range from shallow and narrow channels less than 30 m wide to deep (ca. 5 m) and wide channels with lateral extents exceeding 80 m. The paleochannel infills (sequences) vary across the channels as well, but generally sequences fining upward are observed regardless of channel geometry. For nearly all paleochannels, sandy deposits at the basal parts are overlain by a heterogeneous succession of fine materials (silt, clay), interrupted by peat and organic mud units at some locations (Fig. 7).
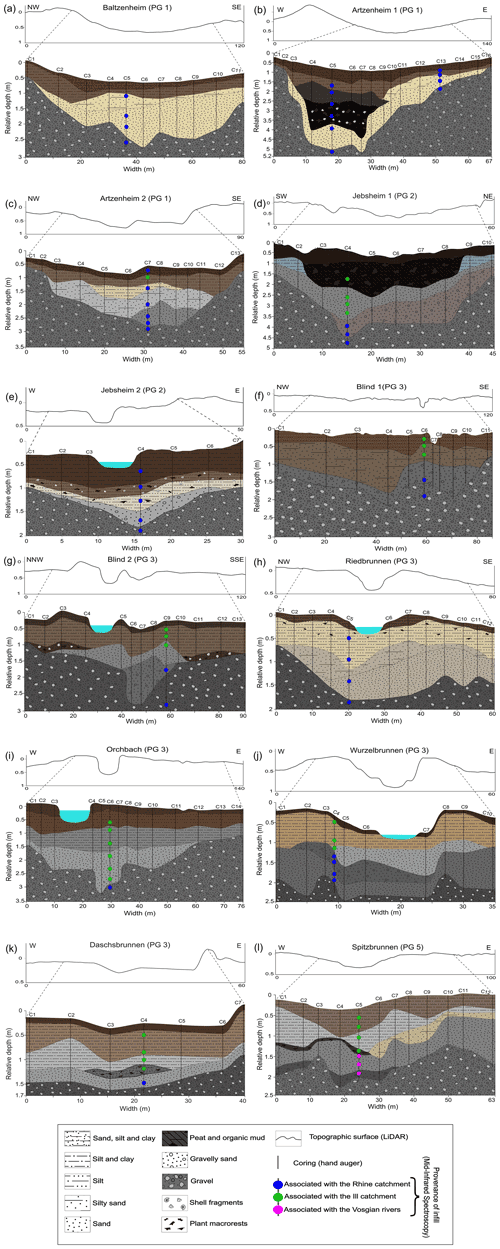
Figure 7Cross-sections of investigated paleochannels showing internal architecture and stratigraphy based on augured boreholes, as well as description and interpretation of the stratigraphical units. Using lidar data, surface topographical profiles were drawn beyond the limit of the corings to show the full extent of the banks and levees. The figure also shows the provenance of the sediments based on mid-infrared spectroscopy analysis (MIRS) (see also Fig. 8).
4.2.1 PG 1: Channel 1: Baltzenheim (48∘05′53.6′′ N, 7∘33′1.9′′ E)
The paleochannel of Baltzenheim is located 2 km west of the current Rhine channel. It is the most eastern of the paleochannels investigated, located within the historical floodplain of the Rhine (“Ried Blond”), and is part of PG 1. This paleochannel has a width of about 80 m and a maximum depth of 2.8 m. The base of the channel infill is composed of 1.5 m thick beige medium sand that extends the entire width of the channel. This unit gradually changes into a subsequent thin layer of beige silty sand. The sandy deposits (medium sand and silty sand) are overlain by brown clayey silt, capped by dark brown silt with some sand that covers the entire width of the channel. MIRS provenance studies indicate that the sedimentary infills of this paleochannel are primarily attributed to the Rhine catchment (Figs. 7a and 8b).
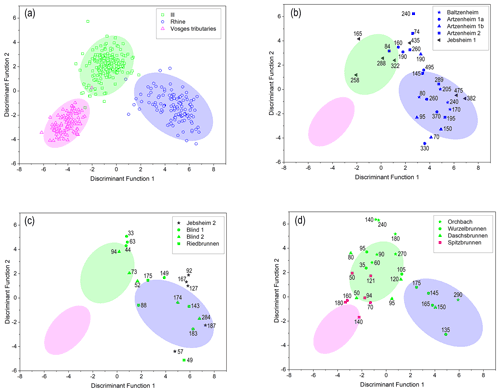
Figure 8(a) Two-dimensional scatter plots showing the MIRS reference samples classified into three distinct source groups based on discriminant analysis (DA). Panels (b), (c), and (d) show the DA scores of the paleochannel (target) samples compared to reference samples. The ellipses show the area where 90 % of the different reference samples are concentrated, while the numbers indicate the depths (in cm) at which the target samples were taken.
4.2.2 PG 1: Channel 2: Artzenheim 1 (48∘06′28.8′′ N, 7∘32′15.2′′ E)
This paleochannel is located 2.5 km west of the present-day Rhine channel within the historical floodplain of the Rhine River and is part of PG 1. However, it exhibits a more complex morphologic and stratigraphic character than the Baltzenheim paleochannel. The paleochannel reaches a maximum depth of around 5 m and a maximum width of 60 m. The lowermost section of the channel is formed of beige-colored fine to medium sand that extends the channel's entire width. Peat and organic mud which range in color from dark brown to black and are approximately 1.5 m thick overlie the sand unit but are restricted to the western and deepest portion of the channel. This peat unit contains abundant plant macrorests and is interbedded with thin, laterally discontinuous clay and carbonate layers (carbonate concretions and shell fragments). The peat/organic mud is topped by a dark gray clayey silt unit marked by shell fragments, pebbles, and thin layers of bright orange coarse sand. On the eastern side of the channel, adjacent to the clayey silt unit, is a layer of beige silty sand with a maximum thickness of 0.8 m. The succession of medium sand, peat, clay, and silty sand is overlain by dark brown clayey silt that spans the entire width of the paleochannel. All units are capped by a 0.5 m thick dark brown organic-rich silt and sand, replete with pebbles, plant macrorests, and traces of plowing. Similar to the sediments of the Baltzenheim paleochannel, all the stratigraphic units of this paleochannel also show a complete Rhine provenance affinity (Figs. 7b and 8b).
4.2.3 PG 1: Channel 3: Artzenheim 2 (48∘06′50.3′′ N, 7∘30′46.3′′ E)
This paleochannel is located about 5 km west of the present-day Rhine channel and is associated with PG 1. The paleochannel has a maximum recorded depth of 2.8 m and is approximately 50 m wide. The sequence of units consists of gray medium sand with intermittent pebbles at the lowermost part of the channel, which is confined to the eastern portion of the channel. The unit gradually changes to gray silty-fine sand with a maximum thickness of ca. 1 m which extends almost the full width of the channel. A thin (ca. 0.5 m thick) beige silt unit follows but is confined only to the center of the channel. This silt is completely covered by a greyish-brown clayey silt unit that spans the channel's width. A dark brown sandy clayey silt with pebbles and plant macrorests completes the sequence. MIRS investigations indicate a Rhine origin for all the stratigraphic units, except for the silt unit, which shows an Ill provenance affinity (Figs. 7c and 8c).
4.2.4 PG 2: Channel 4: Jebsheim 1 (48∘07′33.3′′ N, 7∘28′06.1′′ E)
This paleochannel is located in a forested area and belongs to PG 2. The paleochannel is approximately 4.5 m deep but relatively narrow (ca. 40 m wide). The basal channel fills are composed of reddish-gray gravelly sand at the lowest level, which gradually changes to an overlying unit of gray medium sand with occasional gravel lenses. The sandy deposits are overlain by a 1 m thick layer of dark brown to black peat with intermittent layers of organic mud and clay. Numerous plant macrorests and shell fragments are found in the peat layer. Laterally adjacent to the peat on both sides is a bluish-gray clayey silt unit with a maximum thickness of 0.5 m. The peat and clayey silt layers are topped with a dark brown organic-rich clayey silt. The unit contains roots, partially decomposed organic debris, and shell fragments. MIRS data indicate that the gravelly sand unit at the base of this paleochannel is Rhine-sourced. In contrast, the overlying medium sand and peat units are in good correspondence with an Ill provenance source (Figs. 7d and 8b).
4.2.5 PG 2: Channel 5: Jebsheim 2 (48∘07′23.7′′ N, 7∘27′42.1′′ E)
This paleochannel is located 600 m southwest of the Jebsheim 1 paleochannel and is also classified as PG 2. This paleochannel, which serves as the course of an unnamed stream, is relatively narrow (about 25 m wide) and shallow, with a maximum recorded depth of 1.7 m. The infill succession of the paleochannel begins with coarse gravelly sand at its lowermost section, followed by beige silt with abundant plant fragments, and eventually a transition to brown organic-rich clayey silt. This clayey unit contains abundant shell fragments and plant macrorests, capped by a dark brown clayey silt with some pebbles. In contrast to the Jebsheim 1 paleochannel, which contains sediments from both the Rhine and Ill systems, Jebsheim 2 shows only Rhine-derived infill sediments (Figs. 7e and 8c).
4.2.6 PG 3: Channels 6 and 7: Blind River (Blind 1 and Blind 2)
This location comprises two paleochannels: one that serves as the course for an inactive tributary of the Blind River (Blind 1: 48∘07′52.9′′ N, 7∘27′13.4′′ E), colloquially named “Old Blind”, and another that serves as the current course for the Blind River (Blind 2: 48∘07′55.7′′ N, 7∘27′08.3′′ E). Both paleochannels are classified as PG 3 and are located within the “Ried Gris”.
The “Old Blind” paleochannel (Blind 1) is relatively wide (about 80 m) but shallow, reaching a maximum depth of 1.8 m. The infill sediment sequence shows a basal gravelly sand unit (maximum thickness 0.7 m) overlain by a 1 m thick brownish-gray silty sand unit which gradually transitions to a brown clayey silt unit that caps the sequence. The gravelly sand at the base of the paleochannel has a Rhine origin, while the overlying units are composed of Ill-derived sediments (Figs. 7f and 8c).
The paleochannel of Blind 2 is also relatively wide (approximately 80 m) and has a depth of 2.8 m. The Blind River does not flow through the deepest section of the paleochannel but rather around 10 m to the west. The flowing channel of Blind 2 appears to be artificial, with a regular trapezoidal shape and an artificial right bank levee. Furthermore, at the top of the deepest part of the paleochannel, the surface topography preserves a former channel that likely corresponds to the natural channel (Fig. 7g). At Blind 2, the paleochannel infill sequence consists of, from bottom to top, a 1 m thick gravelly sand unit concentrated in the middle of the paleochannel, overlain by gray fine to medium sand that reaches a maximum thickness of 0.6 m. Overlying the medium sand on both the eastern and western channel margins is a layer of dark brown organic clay with shell fragments and some pebbles. A brown clayey silt unit (0.8 m thick) lies above the organic clay, followed by an organic-rich silty-clay unit. MIRS results show an origin from the Rhine for the gravelly sand unit, while the overlying fine sand and clayey silt units have an Ill origin (Figs. 7g and 8c).
4.2.7 PG 3: Channel 8: Riedbrunnen (48∘09′19.4′′ N, 7∘26′46.2′′ E)
The Riedbrunnen paleochannel is classed as PG 3 and contains the current course of the Riedbrunnen River, which flows near the deepest section of the paleochannel. The paleochannel is relatively shallow and narrow, reaching a maximum depth of 1.9 m and a width of about 60 m. At the channel's base, a greige color, fine to medium sand unit (maximum thickness 0.8 m) is overlain by a greige silty sand unit (maximum thickness 0.5 m). Following this unit is a succession of beige to light gray sandy silt with abundant plant detritus in its upper part. The upper 0.2 m of the channel infill is formed of dark brown organic-rich silty clay with partially decomposed plant macrorests. Sediment provenance analyses indicate that all the infill sediments of the Riedbrunnen paleochannel originate from the Rhine catchment (Figs. 7h and 8c).
4.2.8 PG 3: Channel 9: Orchbach (48∘09′45.7′′ N, 7∘25′54.7′′ E)
The Orchbach is another PG 3 paleochannel within the floodplain of the Ill (“Ried Gris”). This paleochannel is located about 350 m east of the Ill River and contains the current course of the Orchbach River. The paleochannel has a maximum depth of 3.2 m and a width of approximately 65 m. The Orchbach River's current channel is about 10 m west of the center of the paleochannel. The lowest section of the paleochannel comprises gray medium to coarse sand with a maximum recorded thickness of 1.5 m. A 1 m thick gray clayey silt unit covering the entire channel width overlies the sand, followed progressively by a brown clayey silt (0.7 m thick) unit with shell fragments and plant macrorests. Dark brown clayey silt with some sand caps the infill succession. Unlike the sediments of the nearby Riedbrunnen paleochannel, which have a Rhine origin, all of the infill sediments of the Orchbach paleochannel are of Ill provenance (Figs. 7i and 8d).
4.2.9 PG 3: Channel 10: Wurzelbrunnen (48∘09′51.6′′ N, 7∘25′07.9′′ E)
Wurzelbrunnen is the first of three paleochannels that have been investigated west of the Ill River. It is also of PG 3 and located around 450 m west of the Ill River in the “Ried Gris”. The paleochannel is currently active and serves as the course of the shallow Wurzelbrunnen stream. The paleochannel is roughly 35 m wide and reaches a maximum depth of 2.4 m. The infill of the paleochannel begins with a gray medium sand unit with a maximum thickness of 1.2 m, which is overlain by gray gravelly sand concentrated in the western and central sections of the paleochannel. The gravelly sand is overlain by another unit of gray medium sand (maximum thickness 1.2 m) that runs the channel's central and eastern sections. The medium sand is covered by a beige-brown clayey silt unit that extends the full width of the paleochannel and is topped by dark brown clayey silt with root fragments and traces of plowing. MIRS analysis indicates that the first two sandy units at the base of the paleochannel are composed of sediments derived from the Rhine provenance area. In contrast, the upper sand unit is primarily associated with the Ill, albeit with a little Rhine influence. The clayey silt has a pure Ill origin (Figs. 7j and 8d).
4.2.10 PG 3: Channel 11: Daschsbrunnen (48∘09′44.8′′ N, 7∘24′43.0′′ E)
The Daschsbrunnen paleochannel is located 450 m west of Wurzelbrunnen and is also a part of PG 3 on the western side of the Ill River. The paleochannel is approximately 40 m wide and 1.6 m deep. The basal 0.5 m are gray fine to medium sand with scattered pebbles, followed by 0.2 m of dark brown to gray silty sand with abundant plant fragments. This silty sand unit is restricted to the mid-section of the paleochannel. The overlying unit consists of gray 1.2 m thick clayey silt, which gradually changes to greyish brown in the upper 0.5 m. The topmost 0.2 m of the paleochannel is built up of dark brown clayey silt. MIRS data reflect a Rhine origin for the basal sand infill, while the overlying silty sand is Ill-derived with some Rhine influence. In contrast, the clayey silt units bear a pure Ill signature (Figs. 7k and 8d).
4.2.11 PG 5: Channel 12: Spitzbrunnen (48∘09′25.5′′ N, 7∘24′0.8′′ E)
Spitzbrunnen is the westernmost of the studied paleochannels, located about 1.9 km west of the Ill River and 2.2 km east of the Fecht River. It is the only paleochannel of PG 5 that has been investigated. The paleochannel reaches a maximum depth of 2.2 m and is approximately 65 m wide. At its base, the paleochannel is filled with dark gray gravelly sand localized in the middle of the channel. A gravel bank separates this gravelly sand from an identical unit on the western side of the channel. The unit directly overlying the gravelly sand is made of gray medium to coarse sand that reaches a thickness of about 1 m. Above the medium sand is a layer of beige silty sand that extends from the center of the channel to the eastern bank. This layer is flanked by a dark gray, organic-rich silty sand that gradually changes to gray clayey silt that stretches the entire channel width. All the units are then topped by brownish-gray clayey silt with a maximum thickness of 0.6 m. Provenance analysis (MIRS) shows that the basal sand units (gravelly sand and medium to coarse sand) of the Spitzbrunnen paleochannel originated primarily from the Vosges source area. In contrast, the overlying silty units are composed of Ill-derived sediments (Figs. 7l and 8d).
5.1 Consolidation of the MIRS method to identify sediment provenance
Chapkanski et al. (2020) pioneered the use of MIRS and discriminant analysis (DA) to identify the provenance of paleochannel infills within the Upper Rhine alluvial plain. However, since the methodology was only tested on one paleochannel, it remained unknown whether the MIRS–DA technique could be widely applied to determine the provenance of paleochannel infillings across a large part of the alluvial plain. As a result, we employed the MIRS–DA approach to determine the provenance of several paleochannel infillings over the whole width of the French Upper Rhine plain. Our findings clearly demonstrate the robustness of this method in identifying the sources of paleochannel infilling, which may have changed during the filling for some paleochannels. The Baltzenheim 1 and Baltzenheim 2 paleochannels, for example, are entirely filled with Rhine sediments, whereas the Orchbach paleochannel is filled with Ill sediments. In contrast, the filling of paleochannels such as Blind, Wurzelbrunnen, and Daschsbrunnen began with Rhine sediments and subsequently transitioned to Ill deposits (Figs. 7 and 8).
Table 2Provenance attribution of target samples containing more than 5 % organic matter (OM) before and after organic matter treatment. The table also shows the deviation of each sample after organic matter removal. F1: discriminant function 1; F2: discriminant function 2.
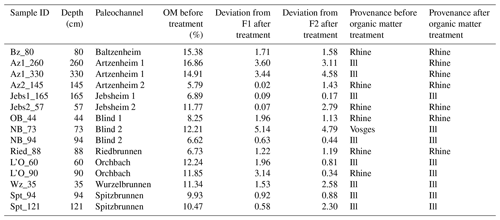
While the MIRS–DA technique has demonstrated great potential for determining the provenance of sediments, Chapkanski et al. (2020) suggested that high organic matter (more than 5 % to 10 %) in sediment samples might affect the mid-infrared spectra, resulting in less accurate measurements. To address this potential constraint, we pre-treated target samples with an organic matter content of more than 5 % (see Sect. 3.3). The results (Table 2) show that the deviations of the samples from their initial position before OM removal remain within a reasonable range of < 1.5 (Mahalanobis distance). However, those samples containing more than 10 % of organic matter recorded deviations ranging from 1.5 to 5, which could induce modification of provenance attribution. However, the results here showed no significant inconsistencies in the attribution of sediment provenance except for two samples (Az1_260 and Az1_330) from peaty deposits and two other samples (NB_73 and L'O_90) from organic-rich units. Therefore, these confirm suggestions provided by Chapkanski et al. (2020), and we recommend systematically pre-treating samples containing more than 10 % of organic matter.
Overall, our findings confirm that the MIRS–DA technique is capable of determining the provenance of paleochannel infills within the French Rhine alluvial plain with high specificity. Moreover, the method is a low-cost and efficient alternative for provenance investigations of deposits in large and complex fluvial systems. The MIRS–DA technique, however, has so far only been applied in the Upper Rhine Valley, and it remains to be tested in other large river floodplains.
5.2 Establishment of sedimentary facies
The subsurface morphology of the investigated paleochannels exhibits a range of geometric forms, as well as varied sedimentary infill (Fig. 7). Several other paleochannels within the French Rhine alluvial plain have shown a similar pattern of varying subsurface morphology and infill stratigraphy (e.g., Schmitt et al., 2016; Chapkanski et al., 2020). These differences in internal sedimentary infills across the plain indicate infilling by rivers with varying dynamics, reflecting lateral dynamics of the Rhine, Ill, and Fecht rivers. To better understand the various dynamics and channel activity over time, we classified the stratigraphic units of our investigated paleochannels into four genetic groups (“Gravel”, “Active”, “Infill”, and “Surficial” facies). These classifications are based on the characterization and interpretations of various Late Pleistocene and Holocene fluvial deposits from the Upper Rhine Valley (Dambeck and Thiemeyer, 2002; Schirmer et al., 2005; Erkens et al., 2009, 2011; Schmitt et al., 2016). By deciphering the different stages of channel activity and assigning a provenance of filling dynamics to the stages, we determined the river system(s) that contributed to the infilling of the investigated paleochannels.
The gravelly sediments at the very bottom of the paleochannels are categorized as “Gravel” deposits. These sediments form the base and lateral limits of the paleochannels and are easily differentiated from the overlying fill sediments by their significantly larger grain sizes, with gravel dominating. This facies is interpreted to have formed by high-energy depositional conditions via non-selective bedload transport (Chardon et al., 2021). Moreover, Gravel is ubiquitously present throughout the study area, and MIRS spectroscopy reveals an unambiguous Rhine origin for these deposits (Figs. 7 and 8). The ubiquity of these gravels, as well as their sedimentary characteristics and provenance, indicates that they are deposits of the Rhine braided belt, which covered nearly the entire plain of the URG during the Late Pleistocene (Lang et al., 2003; Kock et al., 2009). It is worth noting that these sediments do not directly constitute the infilling sequences of the paleochannels. However, some fluvial reworking of the sediments and their deposition within the stratigraphic infills may occur, thus forming essential components for understanding channel dynamics.
The “Active” facies constitute the sand-rich stratigraphic units (gravelly sands, sands, and silty sands) at the basal parts of the paleochannels and overlying the Pleistocene gravel (Gravel). These sandy strata are interpreted as channel deposits, comprising gravelly channel lags (bedload) and sandy channel fills (bedload and graduated suspension; Plotzki et al., 2015; Delile et al., 2016). The deposits indicate deposition during moderate- to high-energy periods of flow at the beginning of infill. So this facies reveals deposition during a permanent or intermittent connection to a basin-fed river. Bank accretion may also have occurred during such periods. Thus, by identifying the Active deposits and their provenance, we were able to discern which rivers were active in infilling of each paleochannel. In contrast to the gravel deposits, which are exclusively Rhine-sourced, the sandy channel deposits (Active) show Rhine, Ill, and Vosges signatures as revealed by MIRS (Figs. 7 and 8). This disparity in provenance sources indicates that the infilling sources changed over time in some cases, highlighting active past lateral dynamics of the main rivers within the alluvial plain.
The succession of silty and clayey layers, interspersed with peat and organic mud, are classified as “Infill” facies. Unlike the Active channel deposits, which are generally restricted to the paleochannels' deepest and central sections, the Infill sediments mostly form a blanket of fine-grained materials that covers the whole width of the paleochannels (Fig. 7). Furthermore, these units are characterized by an abundance of plant macrorests and shell fragments. Accordingly, these units' stratigraphic position and sedimentary characteristics represent low- (silt) to very low-energy (clay, organic mud, peat) depositional environments. Silt and clay transport (and deposition) also indicates a homogeneous suspension (Delile et al., 2016). In such a context, channels have no (or a weak) upstream connection to a basin-fed river. The peat and organic mud, which can be considered a sub-class of the Infill facies (given the close association in the successions), indicate autochthonous sedimentation and starvation or minimal input of clastic sediments in the channels (Hoffmann et al., 2008; Toonen et al., 2012).
Consequently, the Infill sediments serve as key stratigraphic markers for determining an important filling phase associated with reduced fluvial activity and a partial or total disconnection from feeding rivers. Such depositions occurred mostly during floods which extended to the channels. Furthermore, establishing the provenance of these sediments was critical for determining which river(s) contributed to the paleochannel filling phase (after their abandonment). Provenance (MIRS) investigations reveal a mixture of Rhine, Ill, and Vosges signatures for the Infill sediments (Figs. 7 and 8), confirming the lateral movements of the basin-fed rivers across the alluvial plain.
The uppermost sequences of the paleochannels are classed as “Surficial” facies and are typically formed of clays and silts (and in some places, reworked floodplain sands and gravel). Additionally, this facies contains biogenic materials and some anthropogenic materials locally. Typically, the uppermost portion of these floodplain surface deposits shows organic soils and traces of agricultural activity (plowing). The pedogenesis indicates (quasi-)total channel abandonment and a very weak sedimentation rate for centuries. In the cases where a current stream runs in the paleochannels, the presence of this surface facies indicates that the current channel is in a (quasi-)steady state (which may be linked in some cases to some human dredging activities; Schmitt, 2001; Schmitt et al., 2007, 2011). Considering the Upper Rhine valley is highly anthropized (Lang et al., 2003; Wantzen et al., 2021), these units are considered to be affected by direct human alteration. Hence, they are only used to develop an understanding of soil formation processes and agricultural practices in the immediate environment. They are not used to make direct inferences regarding the fluvial processes within the alluvial plain.
5.3 Reconstructing the temporal trajectories of the five paleochannel groups
Remote sensing combined with qualitative and quantitative morphometric analysis of the surface topography provided a basis for classifying five paleochannel groups within our study area. Additional datasets from the fieldwork and provenance analysis provide information into further complexities amongst these paleochannel groups. In the following section, we combine all of the datasets, as well as information from earlier studies, to provide insights into the origins and the temporal trajectories of these paleochannel groups and speculate on the various basin-fed rivers with which they may be associated.
5.3.1 PG 1
The PG 1 paleochannels in the easternmost sector of the study area are clearly visible in the landscape, with the highest values of width and depth of all the paleochannel groups (Fig. 6; Table 1). These paleochannels exhibit a relatively high degree of sinuosity (average 1.4), as well as a multi-threaded channel pattern, and are almost entirely composed of Rhine-sourced infill sediments, as shown by the paleochannels Baltzenheim, Artzenheim 1, and Artzenheim 2 (Fig. 7a–c). The topographic surfaces and lidar analysis indicate a braided channel pattern. However, the remaining streams in this part of the alluvial plain exhibit an anastomosing channel pattern (Schmitt et al., 2007, 2011). Furthermore, as depicted on historical maps, the sub-modern Rhine system in this region was characterized by a similar anastomosing channel pattern (see Supplement). Thus, we consider the PG 1 paleochannels to consist of two main generations of paleochannels: an initial system with a braided channel pattern, which metamorphosed (fluvial metamorphosis) to the current anastomosing (and paleo-anastomosing, i.e., disconnected upstream) system. Accordingly, the PG 1 paleochannel group can be described as a palimpsest, consisting of the current and paleo-anastomosing patterns, with a low level of flow energy, superimposed on – and controlled by – the former braided channel pattern of the highly dynamic (with a high level of energy) Late Glacial Rhine fluvial hydrosystem (Schmitt, 2010).
5.3.2 PG 2
The south-southeast–north-northwest-oriented PG 2 paleochannels exhibit a similar multi-threaded pattern to PG 1. They are, however, less sinuous (average 1.27) than the PG 1 paleochannels. The surface channel pattern of these paleochannels is obscured by agricultural activity in the area, which has smoothed much of the microtopography. However, visual inspection of the elevation data and aerial photographs reveals traces of some features that appear to reflect a braided channel pattern. This is reinforced by high values of the width / depth ratio (Schmitt et al., 2007). Furthermore, the PG 1 and PG 3 paleochannels to the east and west respectively appear to cross and intersect the PG 2 paleochannels, which may indicate an older age for the PG 2 paleochannels. Based on these observations, it can thus be suggested that the PG 2 paleochannels are relicts of the Late Glacial braided Rhine system, with a more south–north flow direction. This fluvial style was the dominant character of the Rhine system during the Late Pleistocene, occupying almost the entirety of the alluvial plain (Lang et al., 2003; Schmitt et al., 2016). At the start of the Holocene, the individualization of the Rhine and Ill rivers resulted in the abandonment and partial filling up of the PG 2 paleochannels.
In comparison to the PG 1 paleochannels, which contain only Rhine-sourced sediments, it is worth noting that the Active facies of the Jebsheim 1 paleochannel show both Rhine and Ill sediments. Gravelly sand at the basal part of paleochannel is Rhine-sourced, while the overlying medium sand has an ill origin (Fig. 7). In contrast, all the stratigraphic units of the Jebsheim 2 paleochannel are Rhine-sourced (Figs. 7 and 8). A similar mixed Rhine–Ill sediment infilling pattern was observed in a northwest-trending paleochannel about 3 km north (downstream) of the Jebsheim 1 paleochannel (Chapkanski et al., 2020). Thus, the presence of Ill-derived sediments in the braided Rhine paleochannels can be explained by branches of the ensuing Holocene anastomosing Ill system re-occupying parts of the paleochannels before it migrated westward as well (Schmitt et al., 2016). Following the lateral migrations of the Rhine and Ill systems and the abandonment of the PG 2 paleochannels, the central area of the alluvial plain most likely remained dry except for periodic large floods.
The channel pattern is relatively more anastomosing in the downstream part of this group (north of Colmar), which is likely due to a general slope decrease.
5.3.3 PG 3
The PG 3 paleochannels are generally south-southwest–north-northeast trending and correspond to paleochannels of the main course of the Ill River, as well as former main and flood channels of this river at the eastern and western banks of the Ill. The surface topography shows these paleochannels to be narrower (average 20.13) than those of the PG 1 and PG 2 groups. The channel pattern shows a relatively high sinuosity, with a general multi-threaded channel pattern (Fig. 5). These paleochannels correspond to Ill-inherited geomorphic features. However, there are significant differences in their internal stratigraphy and sediment provenance. The Blind paleochannels, for example, are first filled by Rhine sediments and afterward by Ill sediments (Fig. 7f and g). The Riedbrunnen paleochannel is entirely filled by Rhine deposits, whereas the nearby Orchbach paleochannel is completely filled with Ill sediments (Figs. 7 and 8). On the western side of the Ill River, a similar Rhine sequence, followed by an Ill infilling sequence, is also recorded for the smaller Wurzelbrunnen and Daschsbrunnen paleochannels. Based on these findings, we propose that the PG 3 paleochannels are actually a mixture of Rhine and Ill paleochannels. The Rhine not only transported and deposited gravelly Active sediments, but Rhine discharge also shaped some of the paleochannels. However, there is no prior record of coeval activity of the Rhine and Ill systems in this area. Following the individualization of the Rhine and Ill, the Rhine shifted westwards progressively, and the channels were abandoned after some of them were more or less filled by Rhine sediments. The ensuing Ill system then reoccupied and modified several of these ancient Rhine paleochannels, depositing Ill sediments.
The investigated PG 3 paleochannels provide an excellent illustration of these dynamics. The Blind paleochannels, for instance, are defined by a meandering/anastomosing channel pattern in the lidar DEM, suggestive of the Ill system. However, the fill stratigraphy reveals “a wide paleochannel” with coarse-grained Active deposits from the Rhine, overlain by Active and Infill sediments bearing an Ill signature. Thus, we presume that the Blind paleochannel was created and used by the Rhine. Following its abandonment, the Ill re-occupied and modified the channel, depositing Ill sediments. This conjecture is consistent with the findings of Carbiener (1969), Hirth (1971), and Schmitt et al. (2016), the latter suggesting that the current Blind River is flowing in a former main channel of the Ill River.
In comparison, the Riedbrunnen paleochannel, located close to the contemporary Ill River, is completely filled with Rhine-derived sediments. This atypical sediment provenance can be explained by a former Rhine channel that was abandoned and completely filled by the Rhine, probably before it moved far further east. As a result, this paleochannel was not re-occupied by the Ill system. Interestingly, the Orchbach paleochannel, 1.5 km east of the Riedbrunnen, contains only Ill-derived sediments. Looking at the spatial position, planform morphology, and infilling of the Orchbach paleochannel, it appears that the Orchbach paleochannel was a former main channel of the Ill River that was created and used solely by the Ill River.
Other paleochannels documented in this section of the alluvial plain (Al Siddik, 1986; Schmitt et al., 2016) show paleochannels of mixed origin, as well as those of pure Rhine and pure Ill origin. Some with a pure Ill provenance were also recorded further downstream of our study area. Based on these findings, we can conclude that the PG 3 paleochannels are best described as a mixture of older Rhine and younger Ill paleochannels. This distribution pattern is also the result of the Ill being relatively smaller than the Rhine, and it did not wholly occupy large areas of the Holocene alluvial plain. This explains why a small part of the Rhine “Hardt Grise” exists close to the Ill River, southwest of the village of Bischwihr (Fig. 3; Hirth, 1971).
5.3.4 PG 4
The PG 4 paleochannels constitute distinctively southwest–northeast-trending paleochannels that originate from the eastern bank of the current Ill River channel. Although field investigations and provenance analysis remain to be undertaken for these paleochannels, analysis of their surface topography and spatial distribution indicates that they are most likely natural flood channels cutting the eastern bank of the Ill River. They were most likely only active during high floods, diverting floodwaters away from the Ill River towards the floodplain. Owing to the lack of a temporal context for these paleochannels, it is unknown if they are contemporaneous with some PG 3 paleochannels or if they were periodically active during historical times. These paleochannels are predominantly concentrated along the regulated reach of the Ill River (where no floods occur anymore), upstream of the Ill River floodplain (Q100 flood discharge; Fig. 5). Therefore, these paleochannels are not currently active, but they were probably active prior to the mid-19th century regulation of the Ill River.
5.3.5 PG 5
The PG 5 paleochannels, the westernmost component, are the narrowest (average 10 m), primarily single-threaded channels with low sinuosity (average 1.18). These paleochannels have a southwest–northeast orientation and a fluvial style different from those of the Rhine and Ill, which may reflect a link with the Fecht River fluvial hydrosystem. Moreover, the infilling of the Spitzbrunnen paleochannel shows an Active facies with Vosges signature, overlain by Infill sediments of Ill provenance (Fig. 7l). This filling pattern indicates that the Spitzbrunnen paleochannel was carved by a Vosgian river and later filled with overbank sediments from the Ill following its abandonment. A detailed examination of the Spitzbrunnen's surface topography indicates parallels to the modern Fecht River. As a result, it can be argued that the Spitzbrunnen paleochannel was most likely a former bed of the Fecht River. Considering the morphology and infill of the Spitzbrunnen, as well as the character and placement of the other PG 5 paleochannels, we propose that the PG 5 paleochannels are very likely to be the former channels and side channels of the Fecht River. Accordingly, we assume that the Fecht River was located farther east within the alluvial plain, and it moved westwards towards its current position over the course of its evolution, naturally and/or by human activities (Schmitt, 2001).
This delineation of a putative Fecht paleochannel cluster and the absence of true Ill paleochannels beyond the Spitzbrunnen imply that the Ill River did not extend beyond the Spitzbrunnen paleochannel in its western lateral migration. This observation is consistent with the suggestion of Schmitt et al. (2016) that the Ill River's western migration during the Holocene was limited to somewhere near the Wurzelbrunnen paleochannel. However, our findings show that the Ill did migrate slightly westward, up to the Daschsbrunnen paleochannel. As a result, we assume that the Ill River's Holocene displacement limit was somewhere between the Daschsbrunnen and Spitzbrunnen paleochannels. This boundary corresponds to an area near the eastern limit of the Fecht's alluvial fan.
Using remote sensing data, we identified and mapped a detailed and extensive network of paleochannels spanning a 19 km wide corridor within the French Upper Rhine alluvial plain. The mapped paleochannels exhibit a range of surface morphological characteristics, including planform character, geometry, and paleo-flow orientations. Additional data from field investigations and provenance analysis revealed considerable differences in the sedimentary characteristics and provenance of the infillings of the paleochannels, indicating significant changes in hydro-geomorphodynamic processes, especially lateral displacements of the main rivers.
Five paleochannel groups (PG 1 to PG 5) were delineated in the study area based on assessments and interpretations of the remotely sensed data, hand-augured core data, and mid-infrared spectroscopic analysis. The PG 1 paleochannels correspond to remnants of the Holocene braided Rhine hydrosystem which is currently predominantly an anastomosing system, while the PG 2 paleochannels represent relicts of the Late Pleistocene braided Rhine. The PG 3 paleochannels, on the other hand, are a complex mixture of paleochannels from the Ill hydrosystem superimposed on the Rhine braided channels. The PG 4 paleochannels are ancient Ill flood channels, while the Fecht river system formed the PG 5 paleochannels.
While our findings unequivocally establish the presence of at least five paleochannel groups in the area, their temporal trajectory remains vague. Age assignments for the paleochannel systems, as well as additional sedimentological analyses, need to be established. Accordingly, further studies on these paleochannels will be carried out to develop these datasets, which will provide new insights into the chronology of paleochannel development, the paleoenvironments in which they formed, and the processes that controlled their formation, all mainly linked to the Holocene lateral dynamics of the Rhine, Ill, and Fecht rivers.
All data relevant for this study are either presented in the main text or the Supplement.
The supplement related to this article is available online at: https://doi.org/10.5194/egqsj-71-191-2022-supplement.
FP and LS designed and supervised the study. Funding was acquired by FP and MA. Fieldwork was carried out by MA, HM, EO, MS, CR, and FS. MA, CR, FS, SC, and DE were responsible for methodology and formal analysis under the supervision of LS. EO, HM, and MS assisted with methodology. MA wrote the original draft with input from SC. The manuscript was reviewed and edited by LS and FP. All authors commented on and approved the final version of the manuscript.
The contact author has declared that none of the authors has any competing interests.
Publisher’s note: Copernicus Publications remains neutral with regard to jurisdictional claims in published maps and institutional affiliations.
This article is part of the special issue “Quaternary research from and inspired by the first virtual DEUQUA conference”. It is a result of the vDEUQUA2021 online conference in September/October 2021.
Special thanks to Alexander Fülling, Elijah Fon, Armelle Ballian, and Amir Bangash for their assistance in the field. Thanks go to Grégoire Skupinski and Jérome Houssier for processing and treating the raw lidar data. We thank the local administrators of the various communes for giving us permission to conduct the fieldwork. We also express our gratitude to the landowners who permitted us to drill on their property. The Regional Council of Grand Est and the European Collectivity of Alsace are also acknowledged for providing the lidar data.
This research has been supported by the Landesgraduiertenförderung Baden-Württemberg (state graduate funding) through a PhD scholarship to Mubarak Abdulkarim.
This open-access publication was funded by the University of Freiburg.
This paper was edited by Christian Zeeden and reviewed by Dominik Faust and one anonymous referee.
Al Siddik, A. M.: Contribution à l'étude de la dynamique de l'humification des sols hydromorphes du Ried Ello-Rhénan (Région d'Illhaeusern, Haut-Rhin), PhD thesis, Nancy 1 University, France, 95 pp., unpublished, 1986.
Bertaux, J., Frohlich, F., and Ildefonse, P.: Multicomponent analysis of FTIR spectra; quantification of amorphous and crystallized mineral phases in synthetic and natural sediments, J. Sediment. Res., 68, 440–447, https://doi.org/10.2110/jsr.68.440, 1998.
Bisson, M., Piccinini, S., and Zanchetta, G.: A multidisciplinary GIS-Based approach for mapping paleoriver migration: A case study of the Serchio River (Lucca Alluvial Plain, Tuscany), GIsci Remote Sens., 48, 566–582, https://doi.org/10.2747/1548-1603.48.4.566, 2011.
Boës, E., Schmitt, L., Schwartz, D., Gebhardt, A., Goepp, S., and Lasserre, M.: L'anthropisation des zones humides de la plaine d'Alsace au cours de la Protohistoire: problématiques d'études à partir des fouilles récentes menées sur les tumulus de Mussig Plaetze (Bas-Rhin), in: L'âge du Fer dans l'arc jurassien et ses marges. Dépôts, lieux sacrés et territorialité à l'âge du Fer. Annales Littéraires, Série “Environnement, sociétés et archéologie”, edited by: Barral, P., Daubigney, A., Dunning, C., Kaenel, G., and Roulière-Lambert, M. J., Presses Universitaires de Franche-Comté, 113–118, ISBN 978-2848672014, 2007.
Bos, J. A. A., Dambeck, R., Kalis, A. J., Schweizer, A., and Thiemeyer, H.: Palaeoenvironmental changes and vegetation history of the northern Upper Rhine graben (southwestern Germany) since the Lateglacial, Neth. J. Geosci., 87, 67–90, https://doi.org/10.1017/S0016774600024057, 2008.
Bowler, J. M.: Quaternary climate and tectonics in the evolution of the Riverine Plain, southeastern Australia, in: Landform evolution in Australasia, edited by: Davies, J. L., and Williams, M. A. J., Australian National University Press, Canberra, 70–112, ISBN 978-0-7081-1194-9, 1978.
Candel, J. H., Makaske, B., Kijm, N., Kleinhans, M. G., Storms, J. E., and Wallinga, J.: Self-constraining of low-energy rivers explains low channel mobility and tortuous planforms, Depos. Rec., 6, 648–669, https://doi.org/10.1002/dep2.112, 2020.
Carbiener, R.: Le Grand Ried d'Alsace, Ecologie d'un paysage, Bull. Soc. Ind. Mulhouse, 1, 15–44, 1969.
Carbiener, R.: Le grand Ried central d'Alsace: ecologie et évolution d'une zone humide d'origine fluviale rhénane, Bull. Écol., 14, 249–277, 1983a.
Carbiener, R.: Brunnenwasser, Encyclopedie de l'Alsace, 2, Publitotal, Strasbourg, 891–900, 1983b.
Carbiener R. and Dillmann E.: Cas type de Rhinau-Daubensand: l'évolution du paysage rhénan dans la région de Rhinau, au coeur du secteur des Giessen, des Muhlbach et Brunnenwasser, in: Die Auen am Oberrhein/Les Zones alluviales du Rhin Supérieur, edited by: Gallusser, W. A., and Schencker, A, Birkhäuser, Switzerland, 113–136, https://doi.org/10.1007/978-3-0348-6237-0_13, 1992.
Chapkanski, S., Ertlen, D., Rambeau, C., and Schmitt, L.: Provenance discrimination of fine sediments by mid-infrared spectroscopy: Calibration and application to fluvial palaeo-environmental reconstruction, Sedimentology, 67, 1114–1134, https://doi.org/10.1111/sed.12678, 2020.
Chardon, V., Schmitt, L., Arnaud, F., Piégay, H., and Clutier A.: Efficiency and sustainability of gravel augmentation to restore large regulated rivers: insights from three experiments on the Rhine River (France/Germany), Geomorphology, 380, 107639, https://doi.org/10.1016/j.geomorph.2021.107639, 2021.
Commission Internationale de l'Hydrologie du Bassin du Rhin: Le bassin du Rhin: monographie hydrologique, ISBN 978-90-12-01775-6, 1977.
Dambeck, R. and Thiemeyer, H.: Fluvial History of the northern Upper Rhine river (south-western Germany) during the Lateglacial and Holocene times, Quatern. Int., 93–94, 53–63, https://doi.org/10.1016/S1040-6182(02)00006-X, 2002.
Delile, H., Schmitt, L., Jacob-Rousseau, N., Grospretre, L., Privolt, G., and Preusser, F.: Headwater valley response to climate and land use changes during the Little Ice Age in the Massif Central (Yzeron basin, France), Geomorphology, 257, 179–197, https://doi.org/10.1016/j.geomorph.2016.01.010, 2016.
Erkens, G., Dambeck, R., Volleberg, K. P., Bouman, M. T. I. J., Bos, J. A. A., Cohen, K. M., Wallinga, J., and Hoek, W. Z.: Fluvial terrace formation in the northern Upper Rhine Graben during the last 20 000 years as a result of allogenic controls and autogenic evolution, Geomorphology, 103, 476–495, https://doi.org/10.1016/j.geomorph.2008.07.021, 2009.
Erkens, G., Hoffmann, T., Gerlach, R., and Klostermann J.: Complex fluvial response to Lateglacial and Holocene allogenic forcing in the Lower Rhine Valley (Germany), Quaternary Sci. Rev. 30, 611–627, https://doi.org/10.1016/j.quascirev.2010.11.019, 2011.
Ertlen, D., Schwartz, D., Trautmann, M., Webster, R., and Brunet, D.: Discriminating between organic matter in soil from grass and forest by near-infrared spectroscopy, Eur. J. Soil Sci., 61, 207–216, https://doi.org/10.1111/j.1365-2389.2009.01219.x, 2010.
Eschbach, D., Schmitt, L., Imfeld, G., May, J.-H., Payraudeau, S., Preusser, F., Trauerstein, M., and Skupinski, G.: Long-term temporal trajectories to enhance restoration efficiency and sustainability on large rivers: an interdisciplinary study, Hydrol. Earth Syst. Sci., 22, 2717–2737, https://doi.org/10.5194/hess-22-2717-2018, 2018.
Fuller, I. C., Reid, H. E., and Brierley, G. J.: Methods in geomorphology: investigating river channel form, in: Treatise on geomorphology, edited by: Shroder, J. F., Academic Press, San Diego, 73–91, https://doi.org/10.1016/B978-0-12-374739-6.00374-2, 2013.
Gabriel, G., Ellwanger, D., Hoselmann, C., Weidenfeller, M., and Wielandt-Schuster, U.: The Heidelberg basin, Upper Rhine Graben (Germany): A unique archive of Quaternary sediments in Central Europe, Quatern. Int., 292, 43–58, https://doi.org/10.1016/j.quaint.2012.10.044, 2013.
Haimberger, R., Hoppe, A., and Schäfer, A.: High-resolution seismic survey on the Rhine River in the northern Upper Rhine Graben, Int. J. Earth Sci. (Geol. Rundsch.), 94, 657–668, https://doi.org/10.1007/s00531-005-0514-z, 2005.
Haughton, P. D. W., Todd, S. P., and Morton, A.C.: Sedimentary provenance studies, Geol. Soc. London. Spec. Publ., 57, 1–11, https://doi.org/10.1144/GSL.SP.1991.057.01.01, 1991.
Hirth, C.: Eléments d'explication à la formation des Rieds ello-rhénans au nord de Colmar du début du Post-glaciaire à la canalisation du Rhin au XIXè siècle, Bull. Soc. Hist. nat. Colmar, 54, 21–44, 1971.
Hoffmann, T., Lang, A., and Dikau, R.: Holocene river activity: analysing 14C-dated fluvial and colluvial sediments from Germany, Quaternary Sci. Rev., 27, 2031–2040, https://doi.org/10.1016/j.quascirev.2008.06.014, 2008.
Illies, J. H. and Greiner, G.: Rhinegraben and the Alpine system, Geol. Soc. Am. Bull., 89, 770–782, https://doi.org/10.1130/0016-7606(1978)89<770:RATAS>2.0.CO;2, 1978.
Jotheri, J., Allen, M. B., and Wilkinson, T. J.: Holocene avulsions of the Euphrates River in the Najaf area of western Mesopotamia: Impacts on human settlement patterns, Geoarchaeology, 31, 175–193, https://doi.org/10.1002/gea.21548, 2016.
Jung, J. and Schlumberger, C.: Soulèvement des alluvions du Rhin par des intrusions salines diapires de Haute-Alsace: déformation de la surface de la basse terrasse du Rhin et tectonique du bassin salifère de Haute-Alsace, Bull. du Service de la Carte géologique d'Alsace et de Lorraine, 3, 77–86, 1936.
Kaufhold, S., Hein, M., Dohrmann, R., and Ufer, K.: Quantification of the mineralogical composition of clays using FTIR spectroscopy, Vib. Spectrosc., 59, 29–39, https://doi.org/10.1016/j.vibspec.2011.12.012, 2012.
Kemp, J. and Spooner, N. A.: Evidence for regionally wet conditions before the LGM in southeast Australia: OSL ages from a large palaeochannel in the Lachlan Valley, J. Quaternary Sci., 22, 423–427, https://doi.org/10.1002/jqs.1125, 2007.
Khosravichenar, A., Fattahi, M., Amini, H., and von Suchodoletz, H.: The potential of small mountain river systems for paleoenvironmental reconstructions in drylands – An example from the Binaloud Mountains in Northeastern Iran, Geosci., 10, 448, https://doi.org/10.3390/geosciences10110448, 2020.
Kock, S., Huggenberger, P., Preusser, F., Rentzel, P., and Wetzel, A.: Formation and evolution of the Lower Terrace of the Rhine River in the area of Basel, Swiss J. Geosci., 102, 307–321, https://doi.org/10.1007/s00015-009-1325-1, 2009.
Kremer, M., Rieb, J.-P., Rebholtz, C., and Delecolle, J.-C.: Écologie des Cératopogonidés de la plaine d'Alsace – I. – Le genre Culicoides des sols humides du Ried, Ann. Parasitol. Hum. Comp., 53, 101–115, https://doi.org/10.1051/parasite/1978531101, 1978.
Lang, A., Bork, H. R., Mäckel, R., Preston, N., Wunderlich, J., and Dikau, R.: Changes in sediment flux and storage within a fluvial system: some examples from the Rhine catchment, Hydrol. Process., 17, 3321–3334, https://doi.org/10.1002/hyp.1389, 2003.
Nandini, C. V., Sanjeevi, S., and Bhaskar, A. S.: An integrated approach to map certain palaeochannels of South India using remote sensing, geophysics, and sedimentological techniques, Int. J. Remote Sens., 34, 6507–6528, https://doi.org/10.1080/01431161.2013.803629, 2013.
Nivière, B., Giamboni, M., Innocent, C., and Winter, T.: Kinematic evolution of a tectonic wedge above a flat-lying décollement: The Alpine foreland at the interface between the Jura Mountains (Northern Alps) and the Upper Rhine graben, Geology, 34, 469–472, https://doi.org/10.1130/G22334.1, 2006.
Ollive, V., Petit, C., Garcia, J. P., and Reddé, M.: Rhine flood deposits recorded in the Gallo-Roman site of Oedenburg (Haut-Rhin, France), Quatern. Int., 150, 28–40, https://doi.org/10.1016/j.quaint.2006.01.006, 2006.
Page, K., Nanson, G., and Price, D.: Chronology of Murrumbidgee River palaeochannels on the Riverine Plain, southeastern Australia, J. Quaternary Sci., 11, 311–326, https://doi.org/10.1002/(SICI)1099-1417(199607/08)11:4<311::AID-JQS256>3.0.CO;2-1, 1996.
Plotzki, A., May, J. H., Preusser, F., Roesti, B., Denier, S., Lombardo, U., and Veit, H.: Geomorphology and evolution of the late Pleistocene to Holocene fluvial system in the south-eastern Llanos de Moxos, Bolivian Amazon, Catena, 127, 102–115, https://doi.org/10.1016/j.catena.2014.12.019, 2015.
Preusser, F.: Characterisation and evolution of the River Rhine system, Neth. J. Geosci., 87, 7–19, https://doi.org/10.1017/s0016774600024008, 2008.
Przyrowski, R. and Schäfer, A.: Quaternary fluvial basin of northern Upper Rhine Graben, Z. Dtsch. Ges. Geowiss., 166, 71–98, https://doi.org/10.1127/1860-1804/2014/0080, 2015.
Resmi, M. R., Achyuthan, H., and Jaiswal, M. K.: Middle to late Holocene paleochannels and migration of the Palar River, Tamil Nadu: Implications of neotectonic activity, Quatern. Int., 443, 211–222, https://doi.org/10.1016/j.quaint.2016.05.002, 2017.
Rossetti, D. F. and Góes, A. M.: Late Quaternary drainage dynamics in northern Brazil based on the study of a large paleochannel from southwestern Marajó Island, An. Acad. Bras. Cienc., 80, 579–593, https://doi.org/10.1590/s0001-37652008000300017, 2008.
Schirmer, W., Bos, J. A., Dambeck, R., Hinderer, M., Preston, N., Schulte, A., Schwalb, A., and Wessels, M.: Holocene fluviatile processes and valley history in the River Rhine catchment, Erdkunde, 59, 199–215, https://doi.org/10.3112/erdkunde.2005.03.03, 2005.
Schmitt, L.: Typologie hydro-géomorphologique fonctionnelle de cours d'eau: recherche méthodologique appliquée aux systèmes fluviaux d'Alsace, PhD thesis, University of Strasbourg, France, 348 pp., unpublished, 2001.
Schmitt, L.: Dynamiques fluviales et gestion environnementale durable des hydrosystèmes. Application à une grande plaine alluviale (Rhin-Ill, Alsace) et à hydrosystème périurbain (Yzeron, Ouest Lyonnais), Habilitation thesis, Lumière University Lyon 2, France, 296 pp., unpublished, 2010.
Schmitt, L., Tremolieres, M., Nobelis, P., and Maire, G.: Complémentarité entre typologies hydro-géomorphologique et biologique de rivières dans l'hydrosystème fluvial ello-rhénan français, in: Protéger, restaurer et gérer les zones alluviales. Pourquoi et comment?/Floodplain protection, restoration, management. Why and How?, edited by: Tremolieres, M., Schnitzler, A., and Silan, P, Editions TEC & DOC, Lavoisier, Paris, 123–136, ISBN 978-2-7430-1011-9, 2007.
Schmitt, L., Lafont, M., Tremolieres, M., Jezequel, C., Vivier, A., Breil, P., Namour, P., Valin, K., and Valette, L.: Using hydro-geomorphological typologies in functional ecology: preliminary results in contrasted hydrosystems, Phys. Chem. Earth, 36, 539–548, https://doi.org/10.1016/j.pce.2009.11.011, 2011.
Schmitt, L., Houssier, J., Martin, B., Beiner, M., Skupinski, G., Boës, E., Schwartz, D., Ertlen, D., Argant, J., Gebhardt, A., Schneider, N., Lasserre, M., Trintafillidis, G., and Ollive, V.: Paléo-dynamique fluviale holocène dans le compartiment sud-occidental du fossé rhénan (France), Rev. Archéologique l'Est, 42, 15–33, 2016.
Schmitt, L., Beisel, J. N., Preusser, F., de Jong, C., Wantzen, K. M., Chardon, V., Staentzel, C., Eschbach, D., Damm, C., Rixhon, G., Salomon, F., Glaser, R., Himmelsbach, I., Meinard, Y., Dumont, S., Hardion, L., Jérôme, H., Rambeau, C., Chapkanski, S., and Brackhane, S.: Sustainable management of the Upper Rhine River and its alluvial plain: Lessons from interdisciplinary research in France and Germany, in: Sustainability research in the Upper Rhine region, concepts and case studies, edited by: Hamman, P. and Vuilleumier, S., Presses Universitaires de Strasbourg, Strasbourg, 201–226, ISBN 978-2-86820-549-0, 2019.
Scorpio, V., Surian, N., Cucato, M., Dai Prá, E., Zolezzi, G., and Comiti, F.: Channel changes of the Adige River (Eastern Italian Alps) over the last 1000 years and identification of the historical fluvial corridor, J. Maps, 14, 680–691, https://doi.org/10.1080/17445647.2018.1531074, 2018.
Simler, L., Valentin, L., and Duprat A.: La nappe phréatique de la plaine du Rhin en Alsace, Sci. Geol., 60, 1–266, 1979.
Striedter, K.: Holozäne Talgeschichte im Unterelsass, PhD thesis, University of Düsseldorf, Germany, 235 pp., unpublished, 1988.
Sylvia, D. A. and Galloway, W. E.: Morphology and stratigraphy of the late Quaternary lower Brazos valley: Implications for paleo-climate, discharge and sediment delivery, Sediment. Geol., 190, 159–175, https://doi.org/10.1016/j.sedgeo.2006.05.023, 2006.
Toonen, W. H., Kleinhans, M. G., and Cohen, K. M.: Sedimentary architecture of abandoned channel fills, Earth Surf. Proc. Land., 37, 459–472, https://doi.org/10.1002/esp.3189, 2012.
Trémolières, M., Eglin, I., Roeck, U., and Carbiener, R.: The exchange process between river and groundwater on the Central Alsace floodplain (Eastern France), Hydrobiologia, 254, 133–148, https://doi.org/10.1007/bf00014108, 1993.
Van Raden, U. J., Colombaroli, D., Gillia, A., Schwander, J., Bernasconi, S.M., van Leeuwen, J., Leuenberger, M., and Eicher, U.: High-resolution late-glacial chronology for the Gerzensee lake record (Switzerland): δ18O correlation between a Gerzensee-stack and NGRIP, Palaeogeogr. Palaeoclimatol. Palaeoecol, 391, 13–24, https://doi.org/10.1016/j.palaeo.2012.05.017, 2013.
Vogt, H.: Le relief en Alsace. Etude géomorphologique du rebord sud-occidental du fossé rhénan, Oberlin, Strasbourg, 239 pp., ISBN 9782853691284, 1992.
von Suchodoletz, H., Pohle, M., Khosravichenar, A., Ulrich, M., Hein, M., Tinapp, C., Schultz, J., Ballasus, H., Veit, U., Ettel, P., Werther, L., Zielhofer, C., and Werban, U.: The fluvial architecture of buried floodplain sediments of the Weiße Elster River (Germany) revealed by a novel method combination of drill cores with two-dimensional and spatially resolved geophysical measurements, Earth Surf. Proc. Land., 47, 955–976, https://doi.org/10.1002/esp.5296, 2022.
Walser, E.: Le bassin du Rhin à l'amont de Bâle et l'influence des lacs sur le régime du fleuve, Houille Blanche, 45, 115–124, https://doi.org/10.1051/lhb/1959028, 1959.
Wantzen, K. M., Uehlinger, U., Van der Velde, G., Leuven, R. S. E. W., Schmitt, L., and Beisel, J. N.: The Rhine River basin, in: Rivers of Europe, 2nd edn., edited by: Tockner, K., Zarfl, C., and Robinson, C. T., Academic Press, London, 331–389, ISBN 9780081026137, 2021.