the Creative Commons Attribution 4.0 License.
the Creative Commons Attribution 4.0 License.
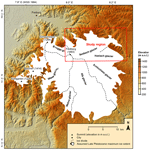
Revisiting ice-marginal positions north-east of Feldberg, southern Black Forest, south-west Germany
Felix Martin Hofmann
Frank Preusser
Hofmann, F. M. and Preusser, F.: Revisiting ice-marginal positions north-east of Feldberg, southern Black Forest, south-west Germany, E&G Quaternary Sci. J., 74, 1–35, https://doi.org/10.5194/egqsj-74-1-2025, 2025.
Whilst previous work has successfully reconstructed the evolution of temperatures in the region north of the Alps during the final phase of the Late Pleistocene, precipitation patterns still remain largely unknown. Recent studies have shown that reconstructing former glaciers in the mid-elevation mountain ranges in central Europe, calculating equilibrium line altitudes (ELAs), and using of empirical relationships between summer temperature and precipitation at the ELAs of modern glaciers could be a promising avenue to fill this gap. Since non-climatic factors probably had only a minor influence on glacier dynamics, the numerous ice-marginal landforms north-east of the highest summit of the Black Forest, Feldberg (1493 m a.s.l. (metres above sea level)), represent prime candidates for climate reconstruction. Since detailed geomorphological and chronological investigations must precede such a study, this work aims to re-examine these landforms with the aid of high-resolution remote sensing data and field mapping. This study allowed for the mapping of glacial landforms in unprecedented detail and for the selection of targets for future dating studies. The re-examination of the glacial record north-east of Feldberg largely confirmed existing studies, and only a few previously mapped ice-marginal landforms must be rejected. At the same time, however, this study identified numerous landforms at former ice-marginal positions that have not yet been described in the literature. These results underline once again that dating studies should always be based on detailed geomorphological mapping. Red-relief image maps (RRIMs) derived from digital elevation models (DEMs) proved to be an extremely helpful visualisation method for the identification of ice-marginal landforms. We therefore advocate for the more frequent use of these maps in glacio-geomorphological studies. Determination of the age of glacial landforms with the aid of cosmic-ray exposure (CRE) dating and luminescence methods will allow for the establishment of an integrated regional stratigraphical model. Once the stratigraphy is constructed, glacier, ELA, and precipitation reconstruction can be undertaken.
Während frühere Arbeiten die Temperaturentwicklung in der Region nördlich der Alpen während der Schlussphase des Spätpleistozäns erfolgreich rekonstruiert haben, sind Niederschlagsmuster noch weitgehend unbekannt. Jüngst erschienene Studien haben gezeigt, dass die Rekonstruktion von ehemaligen Gletschern in den Mittelgebirgen Zentraleuropas, die Berechnung von Gleichgewichtslinien und die Verwendung empirischer Beziehungen zwischen der Sommertemperatur und dem Niederschlag an Gleichgewichtslinien aktueller Gletscher ein vielversprechender Weg sein könnten, um diese Lücke zu schließen. Da nicht-klimatische Faktoren wahrscheinlich nur einen geringen Einfluss auf die Gletscherdynamik hatten, stellen die zahlreichen Landformen an ehemaligen Eisrändern nordöstlich des Feldbergs erstklassige Kandidaten für die Klimarekonstruktion dar. Da einer solchen Studie detaillierte geomorphologische und chronologische Untersuchungen vorausgehen müssen, zielt diese Arbeit darauf ab, diese Landformen mit Hilfe von hochauflösenden Fernerkundungsdaten und Feldkartierungen neu zu untersuchen. Diese Studie ermöglichte es, glaziale Landformen in noch nie dagewesener Detailtreue zu kartieren und Ziele für zukünftige Datierungsstudien auszuwählen. Die erneute Untersuchung des glazialen Formenschatzes nordöstlich des Feldbergs bestätigte weitgehend die bisherigen Studien und nur einige wenige zuvor kartierte Landformen müssen verworfen werden. Gleichzeitig wurden in dieser Studie aber auch zahlreiche Landformen an ehemaligen Eisrandlagen identifiziert, die in der Literatur noch nicht beschrieben wurden. Diese Ergebnisse unterstreichen abermals, dass Datierungsstudien immer auf einer detaillierten geomorphologischen Kartierung beruhen sollten. Aus digitalen Höhenmodellen abgeleitete rote Reliefbildkarten (Red-Relief Image Maps) erwiesen sich als äußerst hilfreiche Visualisierungsmethode für die Identifizierung von Landformen an ehemaligen Eisrandlagen. Deshalb befürworten wir den häufigeren Gebrauch von diesen Karten in glazialgeomorphologischen Studien. Die Bestimmung des Alters der glazialen Landformen mit Hilfe von Oberflächenexpositionsdatierungen und Lumineszenzmethoden wird es ermöglichen, ein integriertes regionales stratigraphisches Modell zu etablieren. Sobald die Stratigraphie erstellt ist, können Gletscher, Gleichgewichtslinien und Niederschläge rekonstruiert werden.
- Article
(34071 KB) - Full-text XML
- BibTeX
- EndNote
During marine oxygen isotope stage (MIS) 2 (29–14 ka; Lisiecki and Raymo, 2005), north-western and northern Europe hosted large ice sheets (Hughes et al., 2016; Batchelor et al., 2019; Clark et al., 2022), whilst a network of valley glaciers and piedmont lobe glaciers covered the Alps and their forelands, respectively (Ivy-Ochs et al., 2008; Preusser et al., 2011; Ivy-Ochs, 2015). Chronological data from glacially transported boulders indicate that ice caps and glaciers also temporarily covered the currently ice-free mid-elevation mountainous regions of central Europe (Fig. 1), including the Jura (Graf et al., 2015), the Vosges (Mercier et al., 1999; Valenti et al., 2024), the Black Forest (Hofmann et al., 2022, 2024a, 2024b; see Hofmann, 2023a, for a synthesis of recent work), the Bavarian Forest (Reuther, 2007), the Bohemian Forest (Mentlík et al., 2013), and the Giant Mountains (Mercier et al., 2000; Engel et al., 2011, 2014). As vast areas are situated above 1000 m a.s.l. (metres above sea level) in its southern part, the Black Forest was one of the most extensively glaciated mid-elevation mountain ranges. Previous studies have pointed towards the existence of four presumably interconnected ice caps with an area of about 1000 km2 that temporarily covered this region during the Late Pleistocene (Fig. 2; Liehl, 1982; Metz and Saurer, 2012; Hofmann et al., 2020). The largest ice cap rested on the highest summit of the Black Forest, Feldberg (1493 m a.s.l. according to the current vertical datum in Germany)), and on the surrounding region (Fig. 2). Its outlet glaciers attained a maximum length and maximum thickness of about 25 km and 440 m, respectively (Sawatzki, 1992; Hemmerle et al., 2016). Both the ice cap on Feldberg and a significantly smaller ice cap on Schauinsland (1284 m a.s.l.) fed the Brugga outlet glacier (Giermann, 1964). Firn fields probably linked the ice cap on Feldberg to two significantly smaller ice caps further west and south-west, covering the summit areas of Köhlgarten (1224 m a.s.l.) and Belchen (1414 m a.s.l.) and the surrounding valleys (Fig. 2; Rahm, 1987).
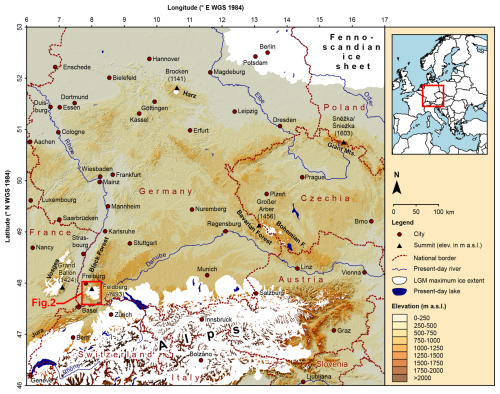
Figure 1Topographical map of central Europe showing the Last Glacial Maximum (LGM) ice extent (Ehlers et al., 2011) and sites discussed in the text. ©DLR 2023 for the digital elevation model (TanDEM-X 30m edited DEM; González et al., 2020) in the background. Rivers and lakes according to European Environment Agency (2008).
Cooling episodes punctuated the general warming trend in central Europe during the last glacial termination (see Heiri et al., 2014, and Palacios et al., 2023, for overviews), informally defined as the period between the Last Glacial Maximum (LGM; at 27.5–23.3 ka based on terrestrial stratigraphical records; Hughes and Gibbard, 2015) and the onset of the Holocene (at 11.7 ka; Cohen and Gibbard, 2019). The presence of numerous moraines within the MIS 2 maximum extents of ice caps and glaciers in the mid-elevation mountains of central Europe (e.g. Wimmenauer et al., 1990; Raab and Völkel, 2003; Krause and Margold, 2019) points to highly dynamic last deglaciation, interrupted by phases of moraine formation. The timing of its onset in the southern Black Forest remains largely unknown. Only three 10Be cosmic-ray exposure (CRE) ages for the region east-south-east of Feldberg currently back up the preliminary minimum age of this glacial phase (∼ 21 ka; Hofmann, 2023a, b). With the aid of 10Be CRE dating of moraine-boulder surfaces, Hofmann et al. (2022, 2024b) attempted to determine the onset of glacier recession from the MIS 2 maximum position north-west of Feldberg but failed, as erosion probably obliterated moraines in one studied valley. The lack of appropriate boulders on moraines at this position in a neighbouring valley prevented 10Be CRE dating of these landforms (Hofmann et al., 2024b). During the last deglaciation, the ice caps of the southern Black Forest decayed into valley glaciers with independent accumulation areas (Liehl, 1982; Metz and Saurer, 2012; Hofmann et al., 2020, 2022, 2024b). In the region north-west of Feldberg, valley glaciers withdrew stepwise from moraines at multiple ice-marginal positions no later than 17–16 ka (Hofmann et al., 2022, 2024b). After the subsequent period of cirque glaciation (no later than 15–14 ka; Hofmann, 2023a; Hofmann et al., 2022, 2024b), the final glacier retreat occurred in most of the cirques, including the Feldsee cirque east-south-east of Feldberg where final glacier decay had begun by 15 ka at the latest (Fig. 2; Hofmann et al., 2024a). In the upper reach of Zastler Valley north of Feldberg, formerly covered by the westernmost branch of the Seebach glacier (Fig. 2), a small cirque glacier may have receded from one of the presumably youngest moraines no later than 13 ka (Fig. 2; Hofmann et al., 2024b). Apart from the areas formerly covered by the Brugga glacier and the westernmost branch of the Seebach glacier as well as the Feldsee cirque, the chronology of Late Pleistocene glacier fluctuations in the southern Black Forest remains largely unknown.
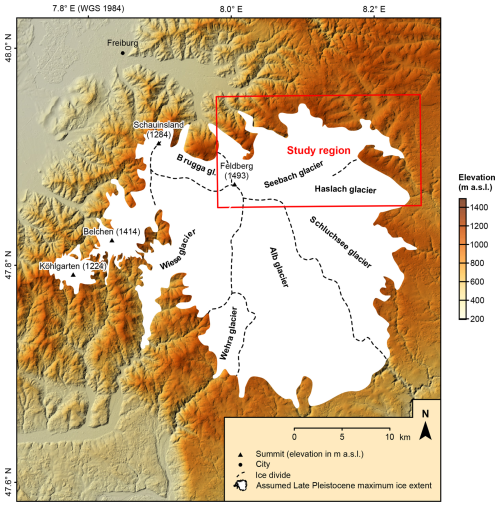
Figure 2Topographical map of the southern Black Forest showing the extent of the Late Pleistocene ice cap (Hemmerle et al., 2016) and its outlet glaciers following the nomenclature of Hofmann et al. (2020). Ice divides according to Hemmerle et al. (2016). ©DLR 2023 for the digital elevation model (TanDEM-X 30 m edited DEM; González et al., 2020) in the background.
Investigating the extent of glaciation in the southern Black Forest and refining the chronology of glacier fluctuations could provide valuable information on regional climate during the last glacial termination. In spite of numerous studies during the last decades (see Heiri et al., 2014, for a comprehensive summary of previous work), climatic variations during the last glacial termination in the region north of the Alps still remain insufficiently understood. Whilst previous work has successfully reconstructed the evolution of temperatures at multiple sites, particularly through the study of chironomid assemblages (e.g. Heiri and Millet, 2005; Lotter et al., 2012; Bolland et al., 2020), precipitation estimates are only available for a few localities (Guiot et al., 1989; Magny et al., 2001, 2006; Peyron et al., 2005). The studies of Rea et al. (2020) and Hofmann et al. (2024b) demonstrate that glacier-based precipitation reconstruction in the mid-elevation mountains of central Europe, involving glacier and equilibrium line altitude (ELA) reconstruction, adjusting independent temperature data to ELAs, and using temperature–precipitation relationships at the ELAs of modern glaciers, might be a promising avenue to fill this gap. At around 16.4±0.7 ka, annual precipitation at the ELA (1142±66 m) of a former valley glacier in Zastler Valley (southern Black Forest), once covered by the westernmost branch of the Seebach glacier (Fig. 2), amounted to 920–2420 mm (Hofmann et al., 2024b). For the subsequent period of cirque glaciation in one of its tributary valleys (no later than 14.6±0.7 ka), these authors reconstructed annual precipitation of 1330–2860 mm at the ELA (1194±66 m). Due to the strong influence of non-climatic factors on the mass balance of this cirque glacier, i.e. snow blow, avalanching, and shading, Hofmann et al. (2024b) concluded that the lower bound of the latter estimate (1330 mm a−1) probably matches the actual precipitation best. Considering the large impact of topoclimatic factors on small glaciers, moraines related to larger ice masses might be more suitable targets for precipitation reconstruction. Due to the high abundance of ice-marginal moraines north-east of Feldberg and the limited topographic control on former ice masses in this region, particularly on the Seebach outlet glacier (Liehl, 1982; Metz and Saurer, 2012; Hemmerle et al., 2016), this region appears particularly suitable for complementing the ELA-based precipitation reconstruction for the Black Forest.
Detailed studies on the extent and the chronology of glaciation in this region must, however, precede any attempt to reconstruct past climate. With regard to the extent of glaciation, the region north-east of Feldberg undoubtedly is one of the best documented areas in the entire southern Black Forest (see Meinig, 1966, 1980; Liehl, 1982; Metz and Saurer, 2012; and Hemmerle et al., 2016, and references therein). However, existing glacio-geomorphological studies of this region (e.g. Meinig, 1966) adopted a rather traditional approach, involving the interpretation of topographical maps and extensive field surveys. The standard of documentation in previous studies is very high (e.g. Meinig, 1966). The adopted mapping approach does, however, not comply with present-day standards in glacio-geomorphological mapping (see Chandler et al., 2018). Today, the identification of glacial landforms almost always involves the interpretation of high-resolution remote sensing data (e.g. Di Costanzo and Hofmann, 2016; Chandler and Lukas, 2017; Krause and Margold, 2019; Tielidze et al., 2023) for three reasons: first, the interpretation of remote sensing data prior to field surveys allows workers to directly target potential glacial landforms, resulting in considerable time savings during mapping. Second, the use of these data permits researchers to detect small-scale landforms that are not obvious during fieldwork due to vegetation cover. Third, sophisticated visualisation techniques, such as red-relief image maps (RRIMs; Chiba et al., 2008), facilitate the mapping process (e.g. Köse et al., 2021, 2022; Altınay et al., 2022).
Recent glacio-geomorphological studies of the region north-west of Feldberg and the Feldsee cirque revealed that several previously described ice-marginal moraines must be rejected, whilst multiple moraines have been mapped for the first time (Hofmann and Konold, 2023; Hofmann et al., 2020, 2022). We hypothesise that the re-examination of the glacial record north-east of Feldberg will lead to similar results. Therefore, this study aims to revisit the glacial record in this region in the light of high-resolution remote sensing data and new field evidence. This study paves the way for future dating studies, which will then enable glacier surface, ELA, and precipitation reconstruction. This paper also reviews the only data currently available on the chronology of glaciation north-east of Feldberg, i.e. minimum ages of moraines, inferred from the study of lake sediments in stratigraphically younger positions (Lang et al., 1984; Merkt, 1985; Lang, 2005), and recently published CRE ages (Hofmann et al., 2024a). Since previous workers proposed a subdivision of the last glaciation of the study region based on minimum deglaciation ages (Lang et al., 1984; Merkt, 1985; Lang, 2005) and geomorphological evidence, we first review this framework in detail. We then present the results of glacio-geomorphological mapping. Note that we will not comment on the temporal assignment of moraines, as this assessment would require reliable geochronological data.
2.1 Study region
The study region is situated in the southern Black Forest, south-west Germany, close to the borders with France and Switzerland (Fig. 1). It encompasses the region formerly covered by the north-eastern outlet glacier of the ice cap on Feldberg, the Seebach glacier (Fig. 3a). The westernmost branch of this glacier temporarily covered Zastler Valley north of Feldberg. Ice-marginal moraines in this valley have already been mapped and dated (Hofmann et al., 2020, 2024b). These landforms are therefore beyond the scope of this study. The study region belongs to the drainage basins of two tributaries of the Rhine, the Wutach and the Dreisam. The western part of this region pertains to the Dreisam catchment, whilst its eastern portion drains into the headstreams of the Wutach. One of those, the Seebach, rises in the area east-south-east of Feldberg. Note that this stream is referred to as the Gutach downstream of Titisee. The Gutach eventually merges with the Haslach and the Wutach downstream of the town of Neustadt (Fig. 3a).
A large portion of the study region belongs to the crystalline part of the Black Forest, representing the basement of the Variscan orogeny (at 380–290 Ma). With the denudation of the orogen, various sediments were deposited on top of the basement under terrestrial and marine conditions during the Permian, Triassic, and Jurassic (see Geyer et al., 2011). Due to tectonic activity associated with the formation of the Upper Rhine Graben (starting at around 50 Ma; Ziegler, 1992), the southern Black Forest was uplifted. Deeply incised and narrow valleys, the Rhenanian valleys, formed on its the westerly exposed side (see Metz and Saurer, 2012). One prime example of such a valley is Höllen Valley (Höllental in German; Fig. 3a). Due to the pronounced difference in elevation, Mesozoic sedimentary rock in the basement was stripped off and has only been preserved in the easternmost part of the study region (LGRB, 2013). At the onset of the formation of the graben, a large portion of the southern Black Forest drained into the Danube towards the east, but due to stronger erosion on the westerly exposed side of the southern Black Forest, the western limit of the drainage basin progressively shifted eastwards (Metz and Saurer, 2012). The last significant change in the watershed occurred when the Wutach was redirected from an easterly to a south-westerly direction towards the Rhine (presumably during the Late Pleistocene; see Hebestreit et al., 1993). Due to generally less erosion in the Danube basin, valleys occupied by current and former tributaries of the Danube feature the Danubian relief type, i.e. gently sloping valley walls and shallow longitudinal profiles (Metz and Saurer, 2012).
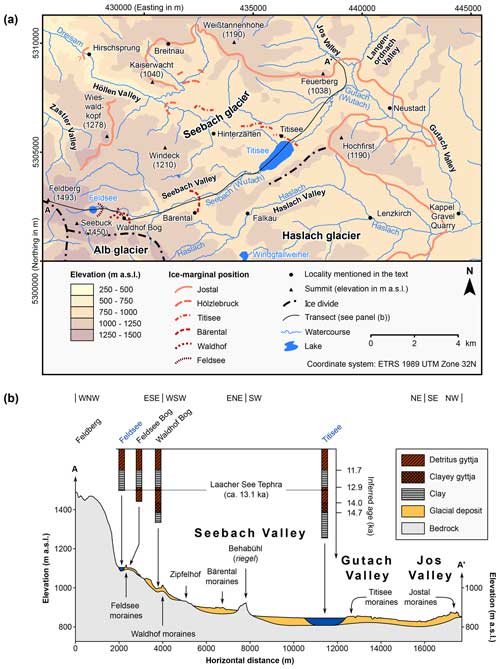
Figure 3(a) Topographical map of the study region showing ice-marginal positions in the region north-east of Feldberg: the Jostal (compiled from Schreiner, 1986; Wimmenauer et al., 1990; Bangert et al., 1992; Schreiner and Wimmenauer, 1999), Hölzlebruck (Meinig, 1980; Schreiner and Wimmenauer, 1999), Titisee (Wimmenauer et al., 1990; Schreiner and Wimmenauer, 1999), Bärental (Wimmenauer et al., 1990), Waldhof (Wimmenauer et al., 1990), and Feldsee (Wimmenauer et al., 1990) positions. Ice divides are from Hemmerle et al. (2016). Watercourses and lakes are according to LUBW (2022a) and LUBW (2022b), respectively. The filled contours in the background were derived from the high-resolution DEM of the study region, utilised for geomorphological mapping (LGL, 2015). (b) Transect from Feldberg to the entrance of Jos Valley and sedimentary successions in lakes and bogs inside moraines. See Lang (2005) for a detailed description of the sediment cores from bogs and lakes. The ages on the y axis are based on the supposed presence of the Laacher See Tephra (reported age of 13 006±9 cal a BP; Reinig et al., 2021) in the cores as well as on the succession of lithostratigraphical units. Note that the sediments have never been radiocarbon dated. Therefore, the inferred ages should be considered with greatest caution (see Hofmann et al., 2020). See panel (a) for the location. Modified and extended from Schreiner (1990).
The widespread glacial landforms in the study region, i.e. cirques, ice-marginal moraines, and glacially scoured basins (Wimmenauer et al., 1990; Schreiner and Wimmenauer, 1999), bear witness to Quaternary glaciation. In general, glacial landforms have generally been better preserved in the Danubian valleys than in the Rhenanian valleys where the Late Pleistocene maximum ice extent can often not be reconstructed with certainty (Liehl, 1982; Hofmann et al., 2022).
2.2 Ice-marginal positions north-east of Feldberg
Previous work concluded that the penultimate glaciation (assumed age of ≥MIS 6 (191–130 ka; Lisiecki and Raymo, 2005)) surpassed the last (Late Pleistocene) glaciation in ice extent (Pfannenstiel and Rahm, 1963; Liehl, 1980; Schreiner, 1986; Hemmerle et al., 2016, their Fig. 2). Since only glacial deposits (e.g. Schreiner, 1986) and erratic boulders (e.g. Pfannenstiel and Rahm, 1963) but no ice-marginal landforms have been mapped beyond the assumed Late Pleistocene maximum ice extent, the maximum extent of the penultimate glaciation remains largely elusive (see Hemmerle et al., 2016).
The study region is not only the birthplace of the theory of glaciations of the Black Forest. In a letter to Louis Agassiz (see Agassiz, 1837), the naturalist Karl Friedrich Schimper mentioned erratic boulders that he had observed in 1826 CE in Höllen Valley (Fig. 3a). The study region is also the birthplace of the commonly accepted subdivision of the Late Pleistocene glaciation. Steinmann (1896, 1902) first systematically mapped moraines in the region around Feldberg and assigned these landforms to three distinct ice-marginal positions. He named these positions after the moraine complex in Jos Valley (Jostal in German), moraines at Titisee, and well-preserved moraines at Feldsee, respectively (Fig. 3a). According to this framework, the Jostal position represents the last glaciation maximum position, whilst the moraines at the Titisee and Feldsee positions formed during the last deglaciation. Several authors, including Bangert (1957), Pfannenstiel and Rahm (1963), and Schreiner (1986), observed glacially transported boulders and till beyond the Jostal position. These observations challenged the implicit assumption of Steinmann (1902) that all glacial landforms north-east of Feldberg formed during the last glaciation (see Schrepfer, 1925). At the same time, however, previous research revealed that glacial deposits beyond the assumed last glaciation maximum position featured considerably higher proportions of weathered clasts (Schreiner, 1986). The tripartite subdivision of Steinmann (1902) became a widely accepted model during the 20th century (Liehl, 1980), and, despite later modifications, Steinmann's model still serves as the base for the current seven-fold subdivision of the last glaciation. This framework comprises the Jostal, Hölzlebruck, Titisee, Bärental, Waldhof, Feldsee, and Seebuck-Nischenkar positions (Table 1 and Fig. 3a; see Hofmann et al., 2020). As no ice-marginal landform formed at the presumably youngest position in the study region, the Seebuck-Nischenkar position (Zienert, 1973), we do not discuss this position any further.
Previous workers ascribed moraines outside the study region to positions north-east of Feldberg based on the morphostratigraphical position, the moraines' morphology (i.e. double-crested or not), and the ELA during moraine formation, calculated with rather crude methods (e.g. Liehl, 1982). In the following, commonly accepted ice-marginal positions north-east of Feldberg are presented, starting with the oldest securely identified position, the Jostal position.
Table 1Ice-marginal positions in the region north-east of Feldberg, characteristics of glaciation, tentative ages inferred from basal ages of lake sediments inside moraines (Tables 12 and 13 in Lang, 2005), and 10Be CRE ages (Hofmann et al., 2024a). Modified from Hofmann et al. (2020).
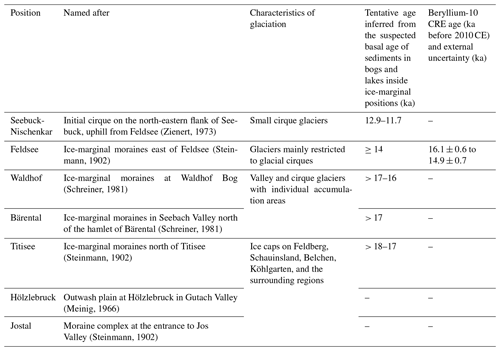
2.2.1 Jostal position
During moraine emplacement at this position, the Seebach glacier covered most of the study region. Only Windeck (Fig. 3a) and a summit further west, Langacker, remained ice-free, as glacially transported boulders do not occur in the areas around these summits (Meinig, 1966). Zastler Valley hosted the westernmost branch of the Seebach glacier at that time. The bulk of the ice from the summit area of Feldberg moved, however, in a northerly and north-easterly direction towards Höllen Valley (Höllental) and Gutach Valley (Gutachtal; Fig. 4a). In the area north-east of Wieswaldkopf, two prominent ice-marginal moraines document the Jostal position (Fig. 3a; Meinig, 1977; Schreiner and Wimmenauer, 1999). The ice extent in narrow and deeply incised Höllen Valley remains largely unknown, as no moraine has been preserved at the assumed last glaciation maximum position. The glacier front was probably situated in the area around the hamlet of Hirschsprung (Fig. 3a), where Platz (1893) observed boulders consisting of reddish granite from the area east of Feldberg. It is unclear whether the two hanging glaciers originating from two initial cirques on the southern valley wall fed the glacier in the main valley (Meinig, 1980; Liehl, 1982).
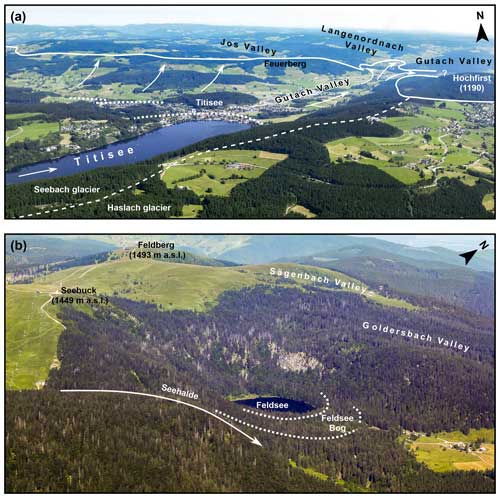
Figure 4(a) Oblique aerial photograph of the region around Titisee (taken from a glider above the village of Falkau). The Jostal position is marked with a solid line. The arrows indicate the ice flow direction. The location of the ice divide on the mountain ridge south-west of Hochfirst is marked with a dashed line. The dotted lines indicate the location of the moraines at the Titisee position. (b) Oblique aerial photograph of the Feldsee cirque and the surrounding region (taken from a glider). The prominent and well-preserved moraines at the Feldsee position are marked with dotted lines. The arrow indicates the direction of ice flow of an ice stream that advanced from the south into Seebach Valley. All photos by Mathias Geyer.
Kaiserwacht (Fig. 3a), a summit north of this valley, probably remained ice-free, as Meinig (1966) did not observe erratic boulders on its summit area. Ice from a southerly and south-easterly direction overran its eastern flank and extended to the tongue basin south-west of the village of Breitnau (Meinig, 1966; Liehl, 1982; Schreiner and Wimmenauer, 1999). A double-crested ice-marginal moraine in this basin has been assigned to the Jostal position (Schreiner and Wimmenauer, 1999).
As till and glaciofluvial deposits occur north-west of the moraine (Liehl, 1970), the ice must have advanced further prior to moraine formation. Since the moraine partly consists of obliquely stratified delta sediments (Meinig, 1966), and lacustrine sediments occur in the tongue basin further north-west (Schreiner, 1999b; Schreiner and Wimmenauer, 1999), the basin must have hosted an ice-dammed lake (Meinig, 1966, 1980; Schreiner, 1999a). The Jostal position in the valley east of Breitnau is marked by a morphologically prominent terrace with an uneven surface. This landform is either a kame terrace (Meinig, 1980), an ice-marginal moraine (Liehl, 1970), or a delta deposited in a former ice-dammed lake (Schreiner, 1999a). Varved sediments of a small ice-dammed lake document the maximum ice extent on the southern flank of Weißtannenhöhe (Meinig, 1980).
In the area further south-east, the ice must have advanced from a southerly direction up to the east–west trending mountain range of Feuerberg (Fig. 4a), as till almost entirely covers its southern flank. Previous authors mapped two north–south trending channels dissecting this mountain ridge. As an accumulation consisting of sand and gravel lies north of one of them, previous studies have interpreted the channels and the sediment accumulation as meltwater channels and as a glaciofluvial deposit, respectively (Neuschel, 1987; Schreiner, 1999a).
A conspicuous multi-ridged landform composed of delta sediments, glaciofluvial deposits, and till occupies the lowermost part of Jos Valley further east (Steinmann, 1902; Meinig, 1980; Schreiner, 1986). Steinmann (1902) interpreted this landform as a moraine complex and named the Jostal position after this multi-ridged feature. During the last glaciation, a branch of the Seebach glacier advanced from a south-easterly direction in an upstream direction into Jos Valley and neighbouring Langenordnach Valley (Langenordnachtal; Fig. 4a), whilst the bulk of the ice flowed in an easterly direction towards the maximum position in Gutach Valley.
The last glaciation maximum ice-marginal position in Gutach Valley remains largely unknown. It has repeatedly been suggested that the glacier margin was situated east of the town of Neustadt (Schreiner, 1986; Liehl, 1982; Schreiner, 1999a), as displayed in Fig. 3a. However, two distinct units of till were exposed in the former Kappel gravel quarry (Kiesgrube Kappel) several kilometres further downstream, i.e. north-west of the Haslach–Gutach confluence (Fig. 3a). The Seebach glacier deposited the uppermost till during either the penultimate (Schreiner, 1986) or the last glaciation (Schiedek and Pfaffenberger, 1993). Hence, the maximum position of the Seebach glacier might have been in the surroundings of the gravel quarry.
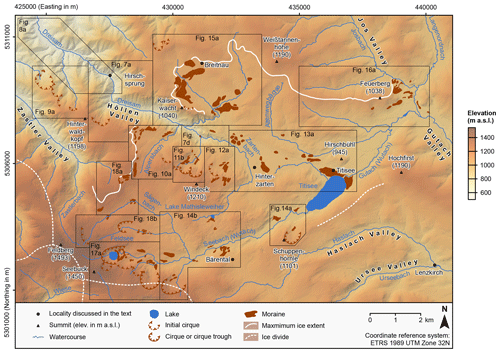
Figure 5Topographic map showing confirmed and newly mapped initial cirques, cirques, the cirque troughs in upper Goldersbach Valley, and ice-marginal moraines in the region formerly occupied by the Seebach glacier. Ice divides are according to Hemmerle et al. (2016). For watercourses and lakes, see LUBW (2022a) and LUBW (2022b), respectively. See LGL (2015) for information on the DEM in the background.
2.2.2 Hölzlebruck position
Moraines at this position are located a few hundreds of metres to 1–2 km inside the Jostal position, south-east of Breitnau (Fig. 3a; Meinig, 1966; Liehl, 1970; Schreiner and Wimmenauer, 1999). These landforms are related to periods of ice-marginal stability or re-advances that interrupted glacier recession from the Jostal position (Meinig, 1966, 1980; Schreiner, 1999a). Schreiner and Wimmenauer (1999) assigned a total of three moraines to this position. According to Meinig (1980), a moraine in the upper reach of Höllen Valley probably documents the Hölzlebruck position in the western portion of the study region (Fig. 3a).
2.2.3 Titisee position
The first unequivocal re-advance of the Seebach glacier that terminated glacier recession from the Hölzlebruck position led to moraine formation at the Titisee position. According to Schreiner and Wimmenauer (1999), the moraines at this position document three individual positions, termed Ti1, Ti2, and Ti3. Moraines at these positions occur north-west of the village of Hinterzarten, in the area between Hinterzarten and the village of Titisee, and in the area north of Titisee (Fig. 3a; Meinig, 1980; Schreiner and Wimmenauer 1999). Lang (2005) inferred from the sedimentary succession in the lake and the stratigraphical position of the apparent Laacher See Tephra (reported age of 13 006±9 cal a BP; Reinig et al., 2021) that the moraines have a minimum age of 14.7 ka (Fig. 3b). He speculated that moraine formation occurred instead at around 18–17 ka.
2.2.4 Bärental position
Wimmenauer et al. (1981) first mapped a group of seven ice-marginal moraines on the southern valley wall of Seebach Valley (Seebachtal), north of the hamlet of Bärental (Fig. 3). Geomorphological mapping revealed that ice-marginal channels separate the landforms (Schreiner, 1990). Schreiner (1981) added the Bärental position (named after the nearby hamlet of Bärental) to the subdivision of the last glaciation, since the ice-marginal moraines point to repeated phases of ice-marginal stability after glacier recession from the Titisee position. Based on the assumed age of the Titisee position, Lang (2005) speculated that moraine formation likely occurred before 17 ka (Table 1).
2.2.5 Waldhof position
Schreiner (1981) named this position after the previously described prominent double-crested ice-marginal moraines that enclose Waldhof Bog (Waldhofmoor or Waldhofwiese; Ramsay, 1862; Erb, 1948; Liehl, 1975). This bog occupies the tongue basin on the floor of the morphologically prominent Waldhof cirque on the southern valley wall (Fig. 3a). Schreiner (1981) introduced the Waldhof position, as sediment cores from both Waldhof Bog and Feldsee Bog (Feldseemoor) near Feldsee further up-valley in Seebach Valley revealed an earlier onset of sedimentation at the former site (Fig. 3b; see Lang, 2005). Liehl (1975, 1982) argued that a small glacier in the Waldhof cirque would have been insufficient to create the morphologically distinct moraines at Waldhof Bog. He suggested that an ice stream advanced from the south over the depression on the cirque's headwall into the cirque. Figure 3a reveals that another ice-marginal moraine simultaneously formed further north-west on the right-hand side of the small valley glacier from the area around Feldsee (Wimmenauer et al., 1990).
2.2.6 Feldsee position
Steinmann (1902) named this position after two prominent ice-marginal moraines east of Feldsee (Figs. 3a, b, and 4b). Moraines at three ice-marginal positions occur at this locality (Hofmann and Konold, 2023). The oldest moraine, exposure dated to 16.1±0.6 ka (Hofmann et al., 2024a), encloses the eastern end of Feldsee Bog (Feldseemoor) east of the lake. Note that this bog evolved from a proglacial lake (Lang, 2005; Hofmann et al., 2024a). The next-youngest moraine subdivides the bog in two distinct basins and has partly been buried by lake sediments and peat (Lang, 2005; Hofmann et al., 2024a). Age–depth modelling based on infrared stimulated luminescence and radiocarbon ages resulted in a modelled basal age of 16 470–15 230 cal a BP, interpreted as a minimum deglaciation and moraine age (Hofmann et al., 2024a). The youngest moraine in the Feldsee cirque dams up the lake. This landform has been exposure dated to 14.9±0.7 ka (Hofmann et al., 2024a). As the Laacher See Tephra also occurs in the sediments of Feldsee (Fig. 3b), Lang (2005) deduced that the cirque basin must have been largely ice-free no later than 13 ka.
3.1 Mapping of ice-marginal landforms
Apart from the Feldsee cirque and its immediate surroundings (Hofmann and Konold, 2023), this study first systematically utilises a high-resolution digital elevation model (DEM) (x–y resolution of 1 m; vertical accuracy of ±0.2 m), derived from light detection and ranging (lidar) data of the Baden-Württemberg State Agency for Spatial Information and Rural Development (LGL, 2015) for the mapping of ice-marginal landforms. Glacio-geomorphological mapping followed well-established standard procedures (e.g. Di Costanzo and Hofmann, 2016; Hofmann et al., 2019, 2020), involving both the analysis of the high-resolution DEM and its derivatives (contour lines, slope, aspect, and hillshades) and extensive field surveys.
As the interpretation of red-relief image maps (RRIMs; Chiba et al., 2008) has proven to be useful for the mapping of ice-marginal landforms (Köse et al., 2021, 2022; Altınay et al., 2022), RRIMs were established for selected key sites. The first step included the calculation of positive (Op) and negative openness (On; Yokoyama et al., 2002) with Relief Visualization Toolbox (RVT; Kokalj and Somrak, 2019) for the QGIS software (3.34.3 Prizren version; QGIS Development Team, 2023) available at https://rvt-py.readthedocs.io/en/latest/rvtfor_qgis.html (last access: 27 February 2024). Note that openness is a measure of the degree of dominance or enclosure of a location on an irregular terrain surface (Yokoyama et al., 2002).
The subsequent calculation of differential openness (Od; Chiba et al., 2008) was based on the following equation:
Raster files representing the topographic slope and Od were finally blended using the multiply option in QGIS. Candidates for glacial landforms were detected in ESRI®ArcGIS (version 10.8.1; Environmental Systems Research Institute, 2020), and their morphometry was assessed using the 3D analyst tool in ESRI®ArcMap. Potential glacial landforms were then directly targeted during extensive field surveys and marked on print-out versions of the shaded relief with superimposed contour lines (1:5000 scale). If available, exposures on ice-marginal landforms were inspected. The geomorphological field maps were finally scanned, imported into ArcMap, georeferenced, and digitised.
3.2 Investigation of glaciofluvial terraces
As discussed above, the maximum position of the Seebach glacier in Höllen Valley is not documented by an ice-marginal landform. Previous workers inferred the location of the maximum position in this valley from the surface profile of morphologically prominent glaciofluvial terraces in its lower reach (Steinmann, 1896; Wimmenauer and Hüttner, 1968; Schreiner and Wimmenauer, 1999). For this study, these landforms have been mapped with derivatives of the high-resolution DEM (LGL, 2015). In ArcMap, the elevation at interval nodes along two parallel transects on both the terrace and the valley bottom was retrieved from the DEM. Subsequently, the elevation at interval nodes on the terraces and the valley bottom, respectively, was plotted against the distance. Different models, including linear and exponential models, were fitted to mathematically describe the terrace surface and the valley bottom best. Identifying the intersection of these models allowed for the reconstruction of the approximate location of the ice margin during glaciofluvial aggradation in the lower reach of Höllen Valley. This approach is based on the observation that several levels of glaciofluvial terraces in the Wutach drainage basin can be linked to ice-marginal moraines (see Hebestreit et al., 1993).
Figure 5 displays confirmed and newly mapped ice-marginal moraines in the region formerly occupied by the Seebach glacier. We hereinafter discuss previous studies, starting with those in the Dreisam drainage basin and subsequently those in the Wutach catchment. Note that the German names of the localities are given in parentheses to allow the readers to easily identify the localities discussed on topographic maps.
4.1 Dreisam drainage basin
Ice-marginal landforms in the Dreisam catchment are hereinafter discussed, starting with those in Höllen Valley (Fig. 6). We subsequently focus on those in its tributary valleys.
4.1.1 Höllen Valley
The upper reach of this valley is referred to as Löffel Valley (Löffeltal; Fig. 7d), the valley of the Zartenbach (LUBW, 2022a). This narrow and steep valley widens at an elevation of about 750 m a.s.l. and transitions into Höllen Valley (Höllental in German; the literal translation is Hell's Valley). This valley narrows downstream of the hamlet of Hirschsprung, widens again upstream of the hamlet of Falkensteig, and eventually leads into Zarten Basin (Zartener Becken; Fig. 6). Höllen Valley pertains to the drainage basin of the Dreisam, a tributary of the Rhine (LUBW, 2022a).
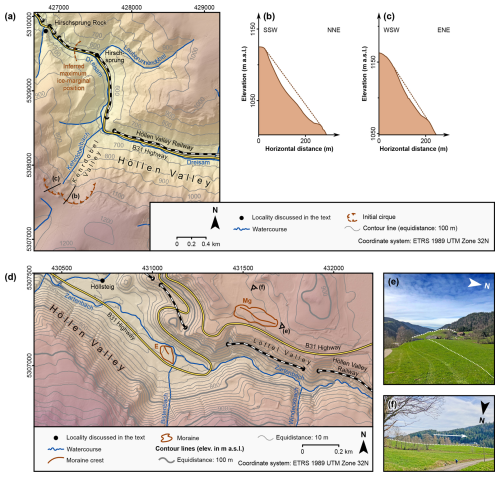
Figure 7(a) Glacio-geomorphological map of Höllen Valley, upstream of Hirschsprung Rock. (b) Transect from Kehrdobel Valley to the plateau above the niche in the south-eastern part of the upper reach of the valley. (c) Transect from Kehrdobel Valley to the plateau above the niche in the south-western part of the upper reach of the valley (three-fold vertical exaggeration). (d) Glacio-geomorphological map of Höllen Valley, upstream of the hamlet of Höllsteig (three-fold vertical exaggeration). E – Erb (1948). Mg – Meinig (1980). (e) View over the ice-marginal moraine (dotted line) at 850–870 m a.s.l. looking towards Höllen Valley. The whaleback in the middle ground is marked with a dashed line. (f) View over the ice-distal side of the ice-marginal moraine at 850–870 m a.s.l. looking towards Löffel Valley. The arrow denotes the direction of ice flow. For watercourses and the DEM in panels (a) and (d), see LUBW (2022a) and LGL (2015), respectively. All photos by FMH.
Field surveys and the interpretation of remote sensing data confirmed the previously mapped glaciofluvial terraces in the surroundings of the village of Falkensteig (Figs. 6 and 8; Steinmann, 1896; Wimmenauer and Hüttner, 1968; Schreiner and Wimmenauer, 1999). Fitting exponential models to the terraces' surface and to the valley bottom yielded the highest coefficient of determination. Figure 8c reveals that these models intersect at an elevation of ca. 540 m a.s.l. We thus confirm the previous hypothesis that the terrace's sediments were laid down by proglacial streams when the margin of the Seebach glacier was situated between Hirschsprung Rock (Hirschsprungfelsen) and the hamlet of Hirschsprung (Fig. 8a and Table 2; see Steinmann, 1896; Liehl, 1982; Leser and Metz, 1988; Metz and Saurer, 2012). Since Platz (1893) did not provide a detailed description of their location, we could not confirm the accumulation of reddish-granite boulders near Hirschsprung. Note that this granite only occurs in the region east and south-east of Feldberg (see Wimmenauer et al., 1990), and, thus, glacial transport was likely involved in the relocation of the boulders. Even if we had confirmed the observation of Platz (1893), their presence would not have permitted us to consider them evidence for glaciation around Hirschsprung. Although these boulders likely underwent glacial or glaciofluvial transport (see Schreiner 1999a), they might have been relocated later by fluvial activity. The distinct change in valley morphology at this locality, first described by Steinmann (1896), more convincingly supports the ice-marginal position near Hirschsprung. Whilst multiple deeply incised tributary valleys dissect the steep valley walls downstream of this locality, the valley widens upstream of Hirschsprung, features the typical cross-section of a trough valley, and has almost no tributary valleys (Figs. 7a and 8a). The distinct change in valley morphology points to intense glacial erosion upstream of Hirschsprung.
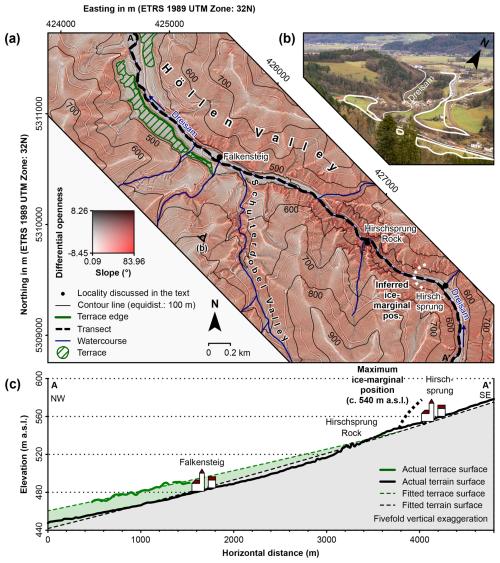
Figure 8(a) RRIM of the lower reach of Höllen Valley before it leads into Zarten Basin. Rivers and streams according to LUBW (2022a). The RRIM was established with the aid of the high-resolution DEM of the study region (LGL, 2015) utilised for geomorphological mapping. The photo location of panel (b) is marked with the eye symbol. (b) View from the southern valley wall over Falkensteig towards Zarten Basin. The terraces are marked with solid lines. See panel (a) for the photo location. Photo by FMH. (c) Transect from the area downstream of Falkensteig to the area upstream of Hirschsprung, showing the actual terrain and terrace surfaces, fitted terrace and terrain surfaces, and the maximum ice-marginal position. See panel (a) for the location.
Figure 7a reveals that we mapped the moraine upstream of the hamlet of Höllsteig, i.e. west of the Zartenbach–Bistenbach confluence, in good faith (Fig. 7d). Indeed, construction work for the widening in of the B31 highway in the 1970s led to the almost complete removal of this landform (Meinig, 1980), composed of silty and boulder-rich till (Erb, 1948). Field mapping and the interpretation of remote sensing data confirmed the ice-marginal moraine at an elevation of 850–870 m a.s.l., north of Löffel Valley, deposited on the right-hand side of the former outlet glacier in Höllen Valley (Table 2 and Fig. 7b; Meinig, 1980).
We confirmed and newly mapped several glacial landforms in tributary valleys. The analysis of the morphology of two niches in the upper reach of Kehrdobel Valley (Fig. 7a, b, c) supports the previous initial cirque interpretation (Schreiner and Wimmenauer, 1999). These landforms point towards the temporary existence of small glaciers, which might have fed the branch of the Seebach glacier in Höllen Valley. Geomorphological mapping in the valley of the Schulterdobelbach (Schulterdobel) confirmed the previously mapped initial cirque in its upper reach (Fig. 9a, b; Schreiner and Wimmenauer, 1999). The longitudinal profile of the upper reach of the valley, i.e. the flattening in its upper reach and the steepening further up-valley, speaks to an initial cirque (Fig. 9b). The same is true for upper reaches of the valleys occupied by the Rotheckbach, the Hogenbächle, and the Weilersbach (Fig. 9c, d, e). The interpretation of the RRIM (Fig. 9a) revealed the presence of a group of three ridges downstream of the Holzeck area (Holzeck; Fig. 9a). The eastern sediment ridge is situated at an elevation of 650–690 m a.s.l. on the eastern valley wall, whilst the western ridges are located at an elevation of 660–690 m a.s.l. on the western valley wall. As the ridges together form an arch, we have tentatively mapped them as ice-marginal moraines. We also identified a sediment ridge at an elevation of 700–740 m a.s.l. on the western valley wall (Fig. 9a). Since this ridge is oriented subparallel to the valley floor, we interpret the ridge as a moraine.
Overall, the study of the glacial landform record provides valuable insights into Pleistocene environmental change in Höllen Valley. The advance of the Seebach glacier to the ice-marginal position near Hirschsprung, the subsequent phase of ice-marginal stability, and the initial phase of glacier retreat led to glaciofluvial aggradation in the lowermost portion of the valley. The streams in tributary valleys with their small glaciers probably carried sediments towards the outwash plain. Due to glacier decay and the cessation of glacial input, the proglacial river abandoned the outwash plain at Falkensteig. Erosional downcutting finally led to the formation of the prominent terraces on the valley floor.
4.1.2 Alpersbach Valley
The Alpersbach, a tributary of the Zartenbach (LUBW, 2022a), drains this tributary valley of Höllen Valley. From the source of the Alpersbach, Alpersbach Valley (Alpersbachtal) extends in a northerly to north-easterly direction (Fig. 10a).
The mountain range that extends from Wieswaldkopf in a northerly direction towards Höllen Valley bounds the valley to the west, whilst the mountain range between Lochrütte Pass (Lochrütte), Langacker (1197 m a.s.l.), and Fürsatz Pass (Fürsatz) delimits the Alpersbach drainage basin to the south. As displayed in Fig. 10a, a step about 200 m high separates the valley from Höllen Valley.
This research confirms the ice-marginal moraines near the Hansele farm (Hanselehof; Fig. 10) first described by Meinig (1966). Their orientation is consistent with the previous interpretation (see Meinig, 1977) that a branch of the Seebach glacier once advanced over Lochrütte Pass towards Höllen Valley. The orientation of the moraine crests suggests moraine emplacement at the western ice margin of the transfluent Seebach glacier. We thus affirm the conclusion of Meinig (1977) that these landforms are not related to an independent glacier in Alpersbach Valley.
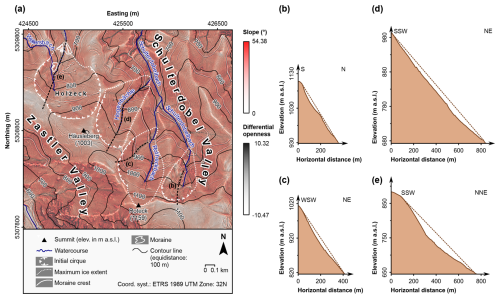
Figure 9(a) Glacio-geomorphological map of Schulterdobel Valley, of its tributary valleys, and of the valley occupied by the Weilersbach, superimposed on an RRIM. Watercourses are according to LUBW (2022a). The RRIM was derived from the high-resolution DEM of the study region (LGL, 2015). (b) Transect from the upper reach of Schulterdobel Valley to the main ridge south of the valley (three-fold vertical exaggeration). (c) Transect from the valley of the Rotheckbach to the north-western flank of Roteck (three-fold vertical exaggeration). (d) Transect from the valley of the Hogenbächle to the north-western flank of Roteck (three-fold vertical exaggeration). (e) Transect from the upper reach of the valley north of Häusleberg to the north-western flank of this mountain (three-fold vertical exaggeration).
We mapped several sediment ridges in the area above the southern valley wall of Höllen Valley for the first time (Fig. 10a). Morphologically distinct depressions separate these landforms. We interpret the ridges and the depressions as ice-marginal moraines and meltwater channels, respectively (Fig. 10a). The large number of moraines at this locality point towards a progressive thinning of the Seebach glacier. We newly mapped two relatively short sediment ridges on the eastern flank of Wieswaldkopf and interpret them as ice-marginal moraines (Fig. 10a). Note that Meinig (1966) has already described an approximately 400 m long north–south trending step on the eastern flank of this mountain. The lack of outcropping bedrock and the undulating surface of this feature do not corroborate the trimline interpretation of Meinig (1966). We suggest instead that this landform is an ice-marginal moraine (Fig. 10a). We newly mapped an ice-marginal moraine north-east of the Sägenbach–Alpersbach confluence (Fig. 10a). This study does not confirm the moraines described by Steinmann (1902), which are located south-east of the Alpersbach (Steinmann, 1902). Indeed, this study corroborates the view of Meinig (1977) that Steinmann (1902) erroneously mapped bedrock ridges as ice-marginal moraines (Fig. 10a).
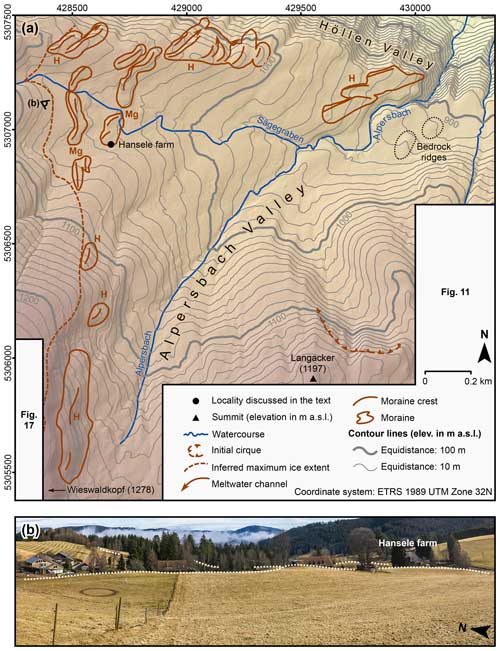
Figure 10(a) Glacio-geomorphological map of Alpersbach Valley. H – Hofmann (this study). Mg – Meinig (1977). Watercourses are according to LUBW (2022a). See LGL (2015) for information on the DEM in the background. The eye symbol indicates the photo location of panel (b). (b) Panorama of the ice-distal sides of the moraines in the surroundings of the Hansele farm. Moraine crests are marked with dotted lines. Photo by FMH.
4.1.3 Bisten Valley
This tributary valley of Höllen Valley is situated east of Alpersbach Valley (Fig. 5) and drains to the Bistenbach, a tributary of the Zartenbach (LUBW, 2022a). Bistenkopf (1115 m a.s.l.) bounds Bisten Valley (Bistental) to the west. The roughly east–west trending mountain range from Fürsatz Pass to Windeck (1210 m a.s.l.) delimits the valley to the south (Fig. 11b). A step about 200 m high separates the valley from Höllen Valley, and the valley thus is a hanging valley.
This study confirms the view of Meinig (1977) that the upper reach of the valley is a cirque complex (Bistenkar; Fig. 11a), consisting of two cirques and an initial cirque further west (Fig. 11b). Geomorphological mapping corroborated the moraines on the western valley wall and those on the valley floor, mapped by Meinig (1977), and revealed the presence of a moraine 150 m north-east of the prominent ice-marginal moraine on the western valley wall, i.e. west of the Bisten farm (Bistenhof). We confirm the moraine 150 m south-west of the Bisten farm. Figure 11b reveals the presence of two faint ice-marginal moraines on the valley floor, which are located north of those mapped by Meinig (1977). Due to their indistinct morphology, meltwater probably overprinted these landforms after their formation.
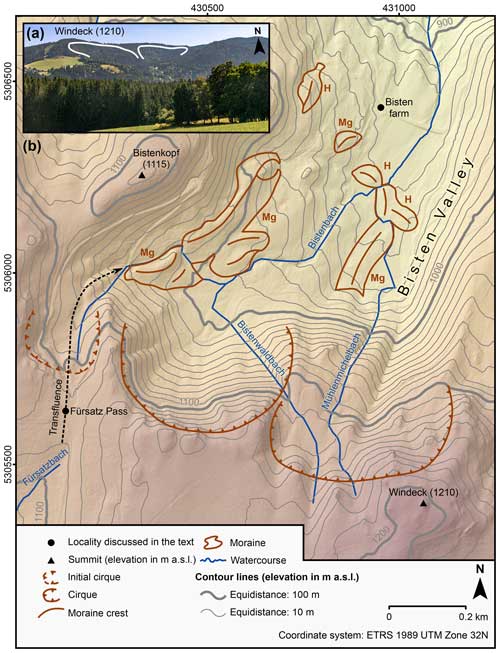
Figure 11(a) Photo of the cirque complex in the upper reach of Bisten Valley, taken from the Föhrwald area (photo by FMH). See Fig. 15a for the photo location. Cirques are marked with solid lines. (b) Glacio-geomorphological map of Bisten Valley. H – Hofmann (this study). Mg – Meinig (1977). Watercourses are according to LUBW (2022a). See LGL (2015) for information on the DEM in the background.
4.1.4 North-eastern and eastern flank of Windeck
The roughly east–west trending mountain range east of Windeck (1210 m a.s.l.) bounds the area to the south (Fig. 12a). A morphologically distinct basin is situated north of this mountain range. The north-eastern and eastern flank of Windeck largely drains to the Windeckbach and the Ospelehofbach. These comparably small streams flow in a northerly direction, tumble down the steep southern valley wall of Löffel Valley, and finally lead into the Zartenbach (LUBW, 2022a).
We mapped two sediment ridges west of the Säbelthoma farm. Figure 12a reveals that a morphologically distinct north-north-east–south-south-west trending depression separates these ridges. We interpret the sediment ridges and the depression as ice-marginal moraines and as a meltwater channel, respectively. It is possible that the westernmost moraine marks the maximum position of the transfluent ice stream that advanced over the main ridge east of Windeck (Fig. 12a). This interpretation is consistent with the observation of Meinig (1966) that glacially transported boulders do not occur on the summit area of Windeck further west. This mountain might thus have been a nunatak during Pleistocene glaciations. Geomorphological mapping in the area east of the Säbelthoma farm revealed the presence of multiple sediment ridges, separated by morphologically distinct depressions (Fig. 12a, c). We interpret these landforms as ice-marginal moraines and meltwater channels, respectively. Note that Liehl (1970, 1982) has already mentioned an ice-marginal moraine at this locality.
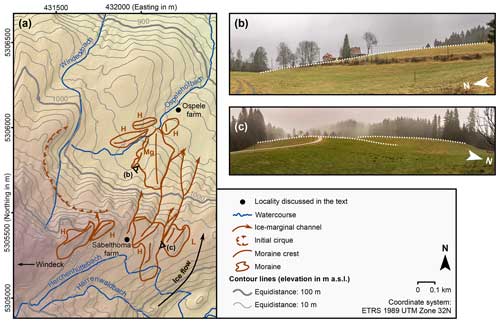
Figure 12(a) Glacio-geomorphological map of the north-eastern and eastern flanks of Windeck (1210 m a.s.l.). H – Hofmann (this study). L – Liehl (1970). Mg – Meinig (1977). Eye symbols indicate the photo locations of panels (b) and (c). Watercourses are according to LUBW (2022a). See LGL (2015) for information on the DEM in the background. (b) View from the floor of the initial cirque south-west of the Ospele farm looking towards the morphologically prominent medial moraine further south-east. The moraine crest is marked with a dotted line. (c) Ice-marginal moraines east of the Säbelthoma farm. See the farm in the background. Moraine crests are marked with dotted lines. All photos by FMH.
The basin south-west of the Ospele farm, with its morphologically distinct semi-circular headwall, has been mapped as an initial cirque (Figs. 12a and 13b). Figure 12a reveals that multiple sediment ridges occur on the cirque floor. The largest and arguably most prominent ridge with a north–south trending crest lies south-south-west of the Ospele farm (Figs. 12b and 13b). Meinig (1977) has already mapped this ridge as a medial moraine but did not clarify whether this landform developed between two transfluent ice streams or between a glacier in the initial cirque and the transfluent Seebach glacier (presumptive ice flow denoted with an arrow in Fig. 12a). Three lines of evidence support the idea that this landform is indeed a medial moraine: first, we deem it unlikely that the former glacier in the initial cirque has left a moraine with such a large volume. Second, the landform lies in the elongation of an ice-marginal moraine east of the Säbelthoma farm. Third, the medial moraine constitutes an arch together with a significantly smaller moraine further west. These arguments support the idea that the moraine is indeed a medial moraine that formed between a transfluent ice stream and the glacier in the initial cirque. We mapped a significantly smaller sediment ridge further north-west. As this ridge constitutes an arch together with the medial moraine, we interpret the sediment ridge as an ice-marginal moraine of the small glacier in the initial cirque. We mapped two sediment ridges north of the medial moraine. Due to their comparably small volumes, the former small glacier from the initial cirque left these landforms. The smooth morphology of the eastern ridge leads to the conclusion that the transfluent Seebach glacier might have overprinted this landform.
4.1.5 Hinterzarten
The village occupies the basin east of Löffel Valley, extending from Hinterzarten towards the village of Titisee and Gutach Valley (Fig. 13a). During the Pleistocene, the formerly existing basin evolved into a glacially scoured basin (Meinig, 1966). With a surface of almost 1 km2 (LUBW, 2006b), Hinterzarten Bog (Hinterzartener Moor) occupies the largest part of the basin. The western part of the basin of Hinterzarten drains into the Zartenbach and the Moosbächle (LUBW, 2022a). The centre of the village, i.e. the townhall, lies on a roughly north-north-west–east-south-east trending ridge. The valley of the Zartenbach narrows upstream of the village of Hinterzarten and extends to the south before it turns in a south-westerly direction. The upper reach of the valley of the Zartenbach is a basin, occupied by both Lake Mathisleweiher (LUBW, 2022b) and Eschengrund Bog (Eschengrundmoos) further south-west (Fig. 14b; LUBW, 2006a).
This study confirms the morphological distinct, 10–25 m high Birklehof moraines north-west of the village of Hinterzarten (marked with “St” in Fig. 13a; Steinmann, 1902), named after the nearby hamlet of Birklehof north of the upper reach of Löffel Valley. Geomorphological mapping also corroborated the presence of two relatively small ice-marginal moraines north-east and north of Hinterzarten Bog (marked with “Mg” in Fig. 13a). Revisiting the area east of the centre of Hinterzarten tentatively supports the ice-marginal moraine mapped by Meinig (1966). Unfortunately, the construction of houses has led to significant changes in the morphology of the terrain surface (Fig. 13b). During field mapping, we did not observe any exposures that would have allowed us to rule out the ridge being composed of bedrock. This study confirms the ice-marginal moraine further east that progresses from a southerly direction into Hinterzarten Bog (marked with “P” in Fig. 13a; Platz, 1893; Meinig, 1966; Schreiner and Wimmenauer, 1999). The analysis of remote sensing data and field mapping confirmed the moraines in the area around Lake Mathisleweiher (Fig. 14b; Metz, 1985), which are situated in the upper reach of the valley of the Zartenbach.
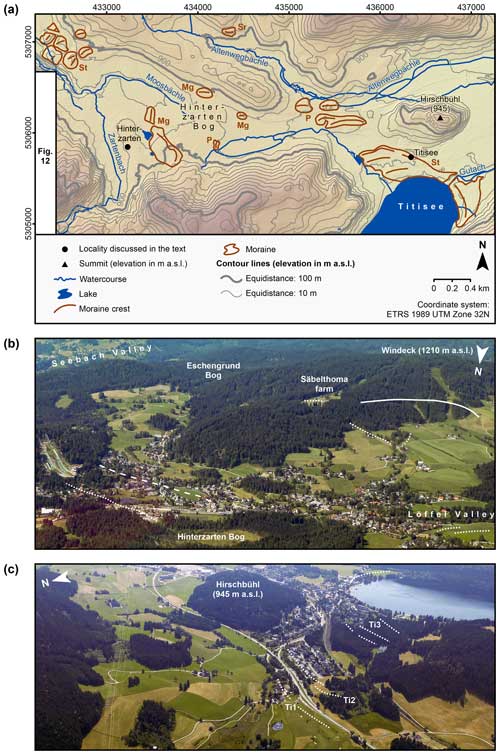
Figure 13(a) Glacio-geomorphological map of the area around the villages of Hinterzarten and Titisee. Mg – Meinig (1966). P – Platz (1893). Sr – Schreiner (Schreiner and Wimmenauer, 1999). St – Steinmann (1902). Watercourses and lakes are according to LUBW (2022a) and LUBW (2022b), respectively. See LGL (2015) for information on the DEM in the background (b) Oblique aerial photograph of the area around Hinterzarten. Ice-marginal moraines are marked with dotted lines. Note the prominent Birklehof moraines close to Löffel Valley. The dashed line indicates the bedrock ridge in the centre of Hinterzarten. The newly mapped initial cirque north-east of Windeck is marked with a solid line. The valley of the Zartenbach in the middle ground leads to Eschengrund Bog and Lake Mathisleweiher. (c) Oblique aerial photograph of the surroundings of Titisee. The ice-marginal moraines at the Titisee position (positions Ti1, Ti2, and Ti3; Schreiner and Wimmenauer, 1999) are marked with dotted lines. All photos by Matthias Geyer.
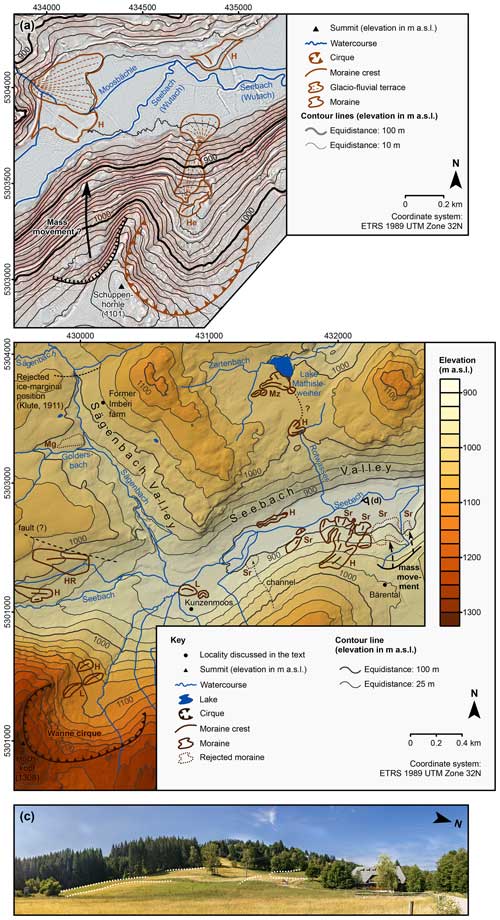
Figure 14(a) RRIM of Seebach Valley, upstream of Titisee, and the Schuppenhörnle cirque. H – Hofmann (this study). He – Haase (1968). (b) Glacio-geomorphological map of Seebach Valley, the lower reach of Sägenbach Valley, and the area around Lake Mathisleweiher. H – Hofmann (this study). HR – Hantke and Rahm (1976). L – Liehl (1975). Mg – Meinig (1980). Mz – Metz (1985). Watercourses and lakes are according to LUBW (2022a) and LUBW (2022b), respectively. See LGL (2015) for information on the DEM in the background. The eye symbol indicates the photo location of panel (c). (c) Panorama of the Bärental moraines on the southern valley wall (photo by FMH).
4.1.6 Breitnau
The village of Breitnau is situated on a mound on the southern flank of Rossberg (1120 m a.s.l.; Fig. 15a). This mountain and Wirbstein (1028 m a.s.l.) bound the basin of Breitnau to the north-east, whilst Hohwart (1120 m a.s.l.) delimits the basin to the north-west.
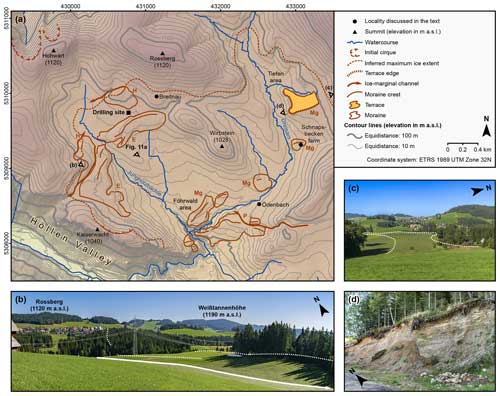
Figure 15(a) Glacio-geomorphological map of the area around the village of Breitnau. Watercourses are according to LUBW (2022a). See LGL (2015) for information on the DEM in the background. The photo locations of panels (b), (c), and (d) and Fig. 11a are shown as eye symbols. E – Erb (1948). Mg – Meinig (1966). H – Hofmann (this study). P – Platz (1893). (b) Photo of the basin of Breitnau, taken from the area north of Kaiserwacht. The crests of the large double-crested ice-marginal moraine in the basin are marked with dotted lines. The solid line with the arrow indicates the ice-marginal channel at the western end of the moraine that extends from the basin to Höllen Valley. (c) View from the south-western flank of Weißtannenhöhe over the terrace in the Tiefen area looking towards the village of Breitnau. The terrace edge is marked with a dashed line. (d) Exposure at the western end of the terrace in the Tiefen area. All photos by FMH.
The mountain range of Kaiserwacht (1040 m a.s.l.) separates the basin from Höllen Valley. It drains to the Jungholzbächle, a tributary of the Dreisam (LUBW, 2022a). The uppermost reach of the valley of the Dreisam, also referred to as Ödenbach (see Meinig, 1966), extends from the Dreisam–Jungholzbächle confluence in a north-easterly direction. The uppermost reach of the Dreisam drainage basin is bounded to the north-east by Weißtannenhöhe (1190 m a.s.l.; Fig. 15b).
The presumably oldest ice-marginal landforms in the surroundings of Breitnau are an ice-marginal moraine south-west of the village (marked with “H” in Fig. 15a), a mound west of the village (Fig. 15a), and a morphologically prominent terrace in the Tiefen area east of Breitnau (Fig. 15a, c). A channel that extends from the valley of the Jungholzbächle towards Höllen Valley bounds the ice-marginal moraine south-west of the village to the east (Fig. 15b). The presence of the channel supports the hypothesis of Erb (1948) and Schreiner (1999a) that meltwater drained from the basin south-west of the village towards Höllen Valley and tumbled down the V-shaped tributary valley west of Kaiserwacht (Fig. 15a). The morphology of the mound west of Breitnau is consistent with the hypothesis of Meinig (1966, 1980) that a branch of the Seebach glacier advanced from the south-east and that a glaciofluvial fan formed at the ice margin. Due to bulldozing induced by the advancing Seebach glacier, the fan evolved into a mound. Since we deem the hypothesis of Meinig (1966, 1980) plausible, we tentatively mapped the landform as an ice-marginal moraine. Unfortunately, we did not observe any road cuts or exposures that would have allowed for the inspection of the landform's sediments. Geomorphological mapping confirmed the terrace in the Tiefen area, situated approximately 2 km east of the village (Fig. 15c). Without detailed investigations according to today's standards in glacial sedimentology, it is impossible to decide whether the landform is a kame terrace (Meinig, 1980), an ice-marginal moraine (Liehl, 1970), or a delta in a former ice-dammed lake (Schreiner, 1999a). Thanks to a relatively large exposure at the western end of the landform (Fig. 15d), future sedimentological studies would offer the potential to unveil its genesis and verify the sedimentological study of Meinig (1962).
The morphostratigraphical positions of the large double-crested moraine south-west of Breitnau (marked with “E” in Fig. 15a) and the moraine south of the terrace in the Tiefen area (marked with “Mg” in Fig. 15a; Meinig, 1980) suggest a similar age of these landforms. Geomorphological mapping confirmed that the arcuate, up to 400 m wide, and 1.5 km long ice-marginal moraine extends from the area south of the village towards the northern flank of Kaiserwacht (marked with “E” in Fig. 15a; Erb, 1948; Meinig, 1966; Schreiner and Wimmenauer, 1999). Sedimentological investigations of an exposure on the ice-distal side of the moraine revealed the presence of obliquely stratified delta sediments (sand and gravel) dipping towards the north-west (Meinig, 1980). This observation reinforces the idea that the landform is an ice-marginal moraine. Sediment cores from a site north-west of the moraine (Fig. 15a) revealed the presence of lacustrine sediments (Schreiner, 1999b). The presence of lake sediments north-west of the moraine suggests that the phase of ice-marginal stability and moraine formation led to the formation of an ice-dammed and/or moraine-dammed lake (Meinig, 1966, 1980; Schreiner, 1999a).
The analysis of remote sensing data and field campaigns confirmed the presumably younger moraine north of the hamlet of Ödenbach (Schreiner and Wimmenauer, 1999) and those in the Föhrwald area, i.e. north of the Dreisam (Ödenbach)–Jungholzbächle confluence (Meinig, 1966, 1980). This is also true for the prominent ice-marginal moraine south of the hamlet of Ödenbach (Liehl, 1970, 1982; Schreiner and Wimmenauer, 1999) and the counterparts west of the Jungholzbächle (Fig. 15a).
The use of derivatives of the high-resolution DEM allowed us to identify a niche on the north-eastern flank of Kaiserwacht (Fig. 15a). We speculate that this landform is an initial cirque, temporarily hosting a firn field, or perhaps a small cirque glacier.
Overall, the mapping results are consistent with the previous hypothesis that the Seebach glacier once advanced from a southerly to south-easterly direction in an up-valley direction to the area around Breitnau (Meinig, 1966, 1980; Schreiner, 1999a). The mountain range of Kaiserwacht (1040 m a.s.l.) probably remained ice-free, as Meinig (1966) did not observe erratic boulders in this locality. This is in line with our own field observations. Compared to earlier investigations (Meinig, 1966, 1980; Schreiner and Wimmenauer, 1999), the mapping results imply a slightly larger maximum ice extent west of Breitnau.
4.2 Wutach drainage basin
The Seebach, the headstream of the Wutach, rises in a basin between the summits of Seebuck and Feldberg; tumbles down the steep western portion of the headwall of the Feldsee cirque; and flows through Feldsee. This stream traverses Feldsee Bog east of the lake and flows in an easterly and then in a north-easterly direction towards Titisee (LUBW, 2022a). Note that the valley of the Seebach (Seebachtal) between Feldsee and Titisee is sometimes referred to as Bären Valley (Bärental; see Meinig, 1980). The headstream of the Wutach is termed Gutach downstream of Titisee. Relatively flat Gutach Valley extends from the lake in a north-easterly direction. At the Josbach–Gutach confluence, a change in valley orientation occurs to the south-west. At an elevation of about 720 m a.s.l., the Gutach and the Haslach merge into the Wutach senso stricto (Fig. 5; LUBW, 2022a).
4.2.1 Gutach Valley
The lack of ice-marginal moraines in the lower reach of Gutach Valley posed major difficulties for the reconstruction of the extent of glaciation. Whilst Liehl (1982) and Schreiner (1999a) suggested that the Late Pleistocene maximum position of the Seebach glacier was situated somewhere downstream of the town of Neustadt (as displayed in Fig. 3a), Schiedek and Pfaffenberger (1993) concluded that the Seebach glacier advanced to the ancient Kappel gravel quarry (Kiesgrube Kappel) north-west of the Gutach–Haslach confluence (Fig. 3a) during the Late Pleistocene. From the bottom to the top, Schiedek and Pfaffenberger (1993) observed the following lithostratigraphical units: gravel, till (lower moraine sensu Schiedek and Pfaffenberger), gravel, silty till (upper moraine sensu Schiedek and Pfaffenberger), lacustrine silt, and silty to sandy periglacial deposits. Schiedek and Pfaffenberger (1993) described the upper moraine as a trapezoidal silty diamict up to 3.5 m thick. Silt overlay the wedge-shaped upper end, whilst gravel covered the wedge-shaped lower end. One of the clasts in the matrix-supported diamict showed clear striations. These observations led to the conclusion that the upper moraine is likely the remnant of a terminal moraine or a former till plain. Since silt covers the wedge-shaped upper end, the moraine or till plain must have dammed up a small lake (Schiedek and Pfaffenberger, 1993).
Although Schiedek and Pfaffenberger (1993) did not date the deposit, they concluded that the Seebach glacier likely emplaced the upper moraine during the Late Pleistocene. For this reason, Schiedek and Pfaffenberger (1993) demanded that the commonly accepted ice-marginal position near Neustadt (Fig. 3a) be reconsidered and introduced into the Kappel position. Revisiting the Kappel gravel quarry revealed that the exposures studied by Schiedek and Pfaffenberger (1993) do not exist anymore. Therefore, we were unable to confirm the presence of the previously suggested ice-marginal moraine or till plain.
The first ice-marginal moraines upstream of the gravel quarry were described by Platz (1893) and later mapped by Schalch (1903). These landforms are two sediment ridges located north-east of the Gutach–Josbach confluence (marked with “P” in Fig. 16a). The analysis of both remote sensing data and field mapping confirmed the moraines.
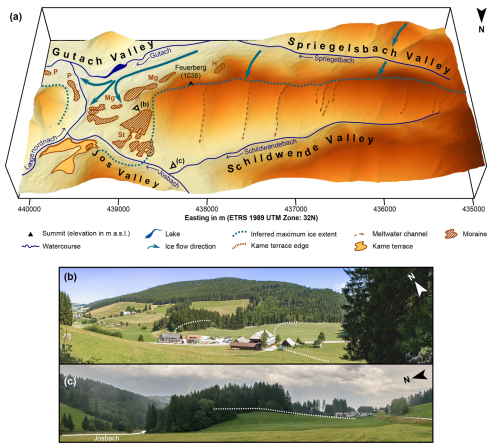
Figure 16(a) Perspective plot of the lower reach of Jos Valley and the surroundings showing moraines, meltwater channels, former ice flow directions, and kame terraces. Watercourses and lakes are according to LUBW (2022a) and LUBW (2022b), respectively. Note that this plot is based on the high-resolution DEM of the State Agency for Spatial Information and Rural Development Baden-Württemberg (LGL, 2015). H – Hofmann (this study). P – Platz (1893). St – Steinmann (1902). See LGL (2015) for information on the DEM in the background. The photo locations of panels (b) and (c) are shown as eye icons. (b) View from the north-eastern flank of Feuerberg looking over the multi-ridged moraine complex at the entrance to Jos Valley. Moraine crests are marked with dotted lines. (c) The north-western end of the moraine complex. The outermost crest of the moraine complex is marked with a dotted line. All photos by FMH.
This study also confirms the previously mapped ice-marginal moraines near the entrance to Jostal and those on the southern flank of Feuerberg (marked with “Mg” in Fig. 16a; Meinig, 1966, 1980). We newly mapped a sediment ridge in a niche on the southern flank of Feuerberg (marked with “H” in Fig. 16a). Field surveys revealed the presence of numerous rounded boulders on the entire southern flank of Feuerberg, which we interpret as glacially transported boulders. Rounded boulders were largely missing on the northern flank of Feuerberg. Two north–south trending channels dissect the mountain ridge west of Feuerberg sensu stricto (Fig. 16a; Neuschel, 1987; Schreiner, 1999a). As a sandy to gravelly deposit lies north of one of them, Neuschel (1987) and Schreiner (1999a) interpreted the channels as meltwater channels. The sandy to gravelly sediments are probably glaciofluvial sediments (Neuschel, 1987; Schreiner, 1999a). The interpretation of high-resolution remote sensing data revealed a total of seven channels on the main ridge and on the northern flank of Feuerberg (Fig. 16a). The presence of glacially transported boulders on the southern flank of Feuerberg supports the idea that the Seebach glacier once covered its whole southern flank and advanced up to the main ridge (Schreiner and Wimmenauer, 1999).
A presumably younger group of ice-marginal moraines is situated north-east, north, and north-west of Titisee. Platz (1893) first described the presumably oldest ice-marginal moraine (ice-marginal positions Ti1 and Ti2 according to Schreiner, 1999a) west of Hirschbühl (Fig. 13a, c). The presumptively younger moraines at ice-marginal position Ti3 (Schreiner, 1999a) surround the northernmost portion of Titisee (Fig. 13a, c; Steinmann, 1896). Figure 13a reveals that moraines indicative of six ice-marginal positions have been mapped during this study.
4.2.2 Seebach Valley
Wimmenauer et al. (1990) noted the presence of two morphologically distinct terraces at an elevation of about 850 m a.s.l. upstream of Titisee (Fig. 14a). The almost flat terraces abruptly slope down to the floodplain of the Seebach. Schreiner (1990) interpreted these landforms as deltas. The Moosbächle from the north-west apparently transported the sediments towards the delta when the lake level was situated at a 10 m higher elevation (Fig. 14a; Schreiner, 1990). This study confirms the two flat-topped terraces on the valley floor upstream of Titisee (Fig. 14a). In contrast to Wimmenauer et al. (1990), we interpret the features as remnants of ice-marginal moraines, mainly due to the arcuate shape of the presumably younger terrace.
A presumably younger double-crested moraine is situated on the saddle that separates Seebach Valley from the basin around Lake Mathisleweiher (Fig. 14b). This landform might be as old as the ice-marginal moraines upstream of the lake, first mapped by Metz (1985).
This study confirms most of the ice-marginal moraines at the Bärental position (Schreiner, 1981), situated at an elevation of about 900 m a.s.l. on the southern valley wall. Note that the two easternmost moraines mapped by Wimmenauer et al. (1990) must be rejected (Fig. 14b). Revisiting this area revealed the presence of two mounds with undulating surfaces, separated by a small stream. We identified a morphologically distinct escarpment further uphill. We thus infer that a rock avalanche likely led to the emplacement of the mounds. We newly mapped a sediment ridge with three crests north of the Seebach. Since this landform constitutes an arch together with a sediment ridge south of the Seebach, we mapped the sediment north of the Seebach as an ice-marginal moraine (Fig. 14b). It should be noted that the presumably youngest moraine mapped by Wimmenauer et al. (1990) must be rejected. In fact, this study revealed the presence of an alluvial fan connected to a channel on the southern valley wall (Fig. 14b).
However, geomorphological mapping in the area around the hamlet of Kunzenmoos further upstream confirmed the presence of the east–west trending ice-marginal moraines north and north-west of the hamlet (Fig. 14b; Liehl, 1975). The east–west orientation of the moraines' crests supports the scenario that the glacier from the Wanne cirque (Wannekar) further south-west laid down these landforms when the Seebach glacier had already withdrawn from this portion of Seebach Valley (see Liehl, 1975).
Hantke and Rahm (1976) mapped an ice-marginal moraine north-west of the Seebach–Sägenbach confluence (Fig. 14b). Field mapping revealed the presence of a ridge at this locality. A morphologically distinct channel separates this landform from the northern valley wall. Note that the ridge's crest is subparallel to the channel. The channel matches a suspected fault on a geological map almost perfectly (see Wimmenauer et al., 1990). We therefore deduce that the presence of bedrock with relatively low erodibility favoured the channel's formation. Since the ridge's crest and the channel have different orientations (east–west and west-north-west–east-south-east, respectively; Fig. 14b), it is unlikely that the ridge is a pseudo-ridge resulting from the removal of rock and sediment in the area around the fault. We thus confirm the moraine interpretation of Hantke and Rahm (1976).
Geomorphological mapping in the area further south-west revealed the presence of a double-crested sediment ridge with east–west oriented crests. As these landforms resemble the ice-marginal moraine further north-west in terms of morphology, we mapped the double-crested ridge as an ice-marginal moraine (Fig. 14b).
Ramsay (1862) first described the morphologically prominent moraines at Waldhof Bog (Waldhofmoor or Waldhofwiese) further upstream (Fig. 17c). Figure 17a reveals these semi-circular landforms. Note that Waldhof Bog evolved from a lake in the cirque basin (Lang, 2005). This study confirms both the prominent partly double-crested moraines at the fringe of the bog and the presumably older moraine further uphill (Wimmenauer et al., 1990). Metz and Saurer (2012) speculated that the moraines at the fringe of Waldhof Bog owe their existence to a former small cirque glacier that was additionally fed by a transfluent ice stream from Menzenschwander Alb Valley (Fig. 17a). The presence of north–south oriented ice-marginal channels east of the Caritas Therapy Centre (Caritas-Haus; Fig. 17a) supports the view that a transfluent ice stream once overrode the saddle at the therapy centre (Liehl, 1982). We argue that the ice-marginal channels are not proof that the transfluence still existed during moraine formation in the Waldhof cirque (Waldhofkar). Indeed, moraine emplacement might have occurred when the saddle was already ice-free.
Wimmenauer et al. (1981) first mapped an ice-marginal moraine west-north-west of the Waldhof cirque, i.e. on the southern valley wall (Fig. 17a). This study confirms the moraine interpretation by Wimmenauer et al. (1990). Geomorphological mapping revealed the presence of a sediment ridge further north-west. Since this landform constitutes an arch together with the aforementioned moraine, we classify the ridge as an ice-marginal moraine. Note that the moraine north of the Seebach (marked with “HR” in Fig. 17a; Hantke and Rahm, 1976) is not the counterpart of the moraines south of the Seebach. This moraine is situated at a higher elevation than those south of the Seebach. We thus deduce that moraine formation required the presence of a thicker glacier at this locality. This interpretation is consistent with the fact that the southern valley wall receives less incoming solar radiation than the east-south-east-exposed northern valley wall.
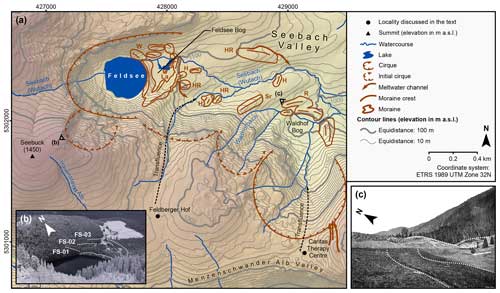
Figure 17(a) Glacio-geomorphological map of the uppermost portion of Seebach Valley and its tributary valleys. H – Hofmann (this study; Hofmann and Konold, 2023). HR – Hantke and Rahm (1976). La – Lang et al. (1984). R – Ramsay (1862). Sr – Schreiner (Wimmenauer et al., 1981). W – Walchner (1846). Watercourses and lakes are according to LUBW (2022a) and LUBW (2022b), respectively. See LGL (2015) for information on the DEM in the background. (b) Oblique aerial photograph of the Feldsee cirque (photo by F. Jesse; published under the CC0 1.0 DEED license). The moraines at ice-marginal positions of the Feldsee cirque glacier (according to the nomenclature of Hofmann and Konold, 2023, and Hofmann et al., 2024a) are marked with dotted lines. (c) Photograph of the moraines at Waldhof Bog taken in 1888 CE (Platz, 1893, 864). The ice-marginal moraines at Waldhof position are marked with dotted lines.
Geomorphological mapping confirmed the previous observation (Hantke and Rahm, 1976) that an ice-marginal moraine emerges at an elevation of 1060 m a.s.l. on the southern valley wall (marked with “HR” in Fig. 17a). A double-crested moraine is situated on the gently sloping valley floor further west (marked with “H” in Fig. 17a; Hofmann and Konold, 2023; Hofmann et al., 2024a). Figure 17a reveals that a group of three subdued moraines occurs on the plateau 0.2 km further north-west, i.e. at the entrance to the Feldsee cirque (marked with “HR” and “H” in Fig. 17a; Hantke and Rahm, 1976; Hofmann and Konold, 2023; Hofmann et al., 2024a). Note that the westernmost of these moraines has been partly buried by a prominent semi-circular moraine at the eastern margin of Feldsee Bog (ice-marginal position FS-03; Fig. 17a, b). The orientation of the crests of the moraines on the valley floor and on the plateau suggests that these landforms likely do not pertain to the former Feldsee cirque glacier but to a transfluent ice stream from the area around the hamlet of Feldberger Hof. Note that Meinig (1980) has already ascribed one of the moraines at the entrance to the Feldsee cirque to this transfluent glacier.
The oldest moraine of the Feldsee cirque glacier (ice-marginal position FS-03; Fig. 17b) is boulder-rich and features a much less smooth crest than those of the transfluent glacier from Feldberger Hof. Since this moraine overlies an ice-marginal moraine of this transfluent glacier, we deduce that the Feldsee cirque glacier reworked the latter during a re-advance. Another semi-circular moraine is situated further west (ice-marginal position FS-02; Fig. 17b) and subdivides Feldsee Bog into two distinct basins (Lang et al., 1984; Lang, 2005; Hofmann et al., 2024a). Note that lake sediments and peat partly cover this moraine. It is therefore only visible south of the bog, in its centre, and north of the bog. The arguably most prominent moraine (ice-marginal position FS-01; Fig. 17b) is situated on the eastern shore of Feldsee. Note that this landform has already been described by Walchner (1846).
4.2.3 Jos Valley and Langenordnach Valley
A morphologically distinct terrace occupies the entrance of Langenordnach Valley (Langenordnachtal). Sedimentological investigations of exposures in a gravel quarry on the terrace revealed a complex sedimentary succession composed of delta sediments, till, again delta sediments, and till (Meinig, 1980). This study confirms the presence of the terrace (Fig. 16a) and the kame terrace interpretation by Meinig (1980).
Steinmann (1902) first described the morphologically prominent moraine complex (Fig. 16) at the entrance to neighbouring Jos Valley (Jostal). According to Meinig (1980, his Fig. 3), this landform has three distinct crests. Steinmann (1902) confirmed the hypothesis of Platz (1893) that a branch of the Seebach glacier advanced from a south-easterly direction into the lower reach of the valley. Revisiting the landform confirmed the moraine complex. Geomorphological mapping revealed the presence of six distinct crests. According to two exposures at two newly created gravel roads, the bottom of the landform consists of deltaic foresets, pointing to the temporary existence of an ice-dammed lake north-west of the landform. Till and silty to sandy deposits occur on top of the deltaic foresets. The top of the landform consists of till. Note that this sedimentary succession is largely consistent with that in a former gravel quarry described by Meinig (1980). Future work should aim to study the sedimentary succession in the exposures in more detail to unveil the depositional history of the moraine complex. According to field observations, the moraine's sediments contain conspicuous reddish granite, which outcrops in the region east and south-east of Feldberg (see Wimmenauer et al., 1990). Its presence reinforces the previous hypothesis that the moraine complex pertains to the Seebach glacier and not to a glacier from the upper reach of Jos Valley (Steinmann, 1902; Meinig, 1966, 1980, Liehl, 1982).
4.2.4 Altenweg Valley
The main stream in this tributary valley (Altenwegtal) of Gutach Valley, the Altenwegbächle, rises in a basin on the southern flank of Weisstannenhöhe and flows in a southerly direction towards the area between Hinterzarten and Titisee (LUBW, 2022a). At an elevation of about 900 m a.s.l., a change in valley orientation occurs to the east-south-east (Fig. 13a). Geomorphological mapping confirmed the presence of a moraine at 910 m a.s.l., north of the Altenwegbächle (Fig. 13a; Schreiner and Wimmenauer, 1999).
4.2.5 Schuppenhörnle cirque
A roughly north-north-east–south-south-west trending tributary valley of Seebach Valley is situated north-east of Schuppenhörnle (Fig. 14a). Geomorphological mapping confirmed the view of Haase (1968) that this landform is a cirque. We therefore informally refer to this landform as Schuppenhörnle cirque (Schuppenhörnlekar). The interpretation of remote sensing data confirms the sediment ridge at the northern end of the cirque floor, connected to a cone-shaped accumulation of sediments and a fan further down-valley (Fig. 14a). Unfortunately, exposures or road cuts were not available to inspect the ridge's sediments. Due to the orientation of the ridge and the landform association, we tentatively confirm the moraine interpretation by Haase (1968) and Wimmenauer et al. (1990). The cone-shaped landform and the fan further downstream likely owe their existence to glaciofluvial aggradation down-valley from the moraine (Fig. 14a).
4.2.6 Wanne cirque
The Wannebach, a tributary of the Seebach, drains this small tributary valley of Seebach Valley (LUBW, 2022a). The upper reach of the valley of the Wannebach is a well-developed cirque (Wannekar) with a conspicuously flat floor (Fig. 14b; Liehl, 1975; Wimmenauer et al., 1990). Glacio-geomorphological mapping confirmed the moraine at the northern end of the cirque floor (marked with “L” in Fig. 14b). In addition, we newly mapped two ice-marginal moraines in its immediate surroundings (Fig. 14b).
4.2.7 Sägenbach Valley
This tributary valley of Seebach Valley, the Sägenbachtal, drains to the Sägenbach, the largest tributary of the Seebach (LUBW, 2022a). Figure 18b reveals that Baldenweger Buck bounds the upper reach of the valley to the west. Rinken Pass further north-east separates this valley from Zastler Valley (Fig. 18b). The mountain range that extends from Schweizerwald (1305 m a.s.l.) to Wieswaldkopf (1278 m a.s.l.) bounds this valley to the north-west. At elevations of 1100 and 1000 m a.s.l., changes in valley orientation occur to the south-east and south, respectively (Figs. 14b and 18a). A confluence step separates Sägenbach Valley from Seebach Valley. Fluvial erosion has, however, largely obliterated this landform (Metz and Saurer, 2012).
Previous reconstructions conclude that the Seebach glacier temporarily entirely covered Sägenbach Valley during the last (Late Pleistocene) glaciation (Liehl, 1982; Metz and Saurer, 2012; Hemmerle et al., 2016). The geological map of the State Geological Survey of Baden-Württemberg at the 1:25 000 scale (Wimmenauer et al., 1990) does not display any ice-marginal moraines in this valley. However, extensive literature mining revealed the potential presence of moraines at multiple localities (Table 3).
Both Platz (1893) and Levy (1912) mentioned ice-marginal moraines at an elevation of 1020 m a.s.l. near the ruins of the Imberi farm (Imberihof; Fig. 14b). Geomorphological mapping did not confirm the presence of moraines at this locality. Likewise, the previously mapped moraine at an elevation of about 1000 m a.s.l. further north-west (Klute, 1911) must be rejected. A field survey revealed the presence of bedrock ridges at this locality (Fig. 14b), covered by till. Liehl (1982, his Table 3) mentioned a presumably younger moraine at an elevation of 1081 m a.s.l. This study reveals the absence of a sediment ridge at this locality (Fig. 18a). Wimmenauer et al. (1990) mapped glaciofluvial deposits at an elevation of 1100 m a.s.l. on the valley floor. We assume that Wimmenauer et al. (1990) probably observed these deposits in a former gravel quarry on a sediment ridge at around 1100 m a.s.l., situated north of the Sägenbach (Fig. 18a). Since this ridge constitutes an arch together with two small sediment ridges south of the Sägenbach (Fig. 18a), we interpret the sediment ridge as an ice-marginal moraine. Note that the former gravel quarry is nowadays completely overgrown with shrubs and trees. Therefore, investigating the sediments in the gravel quarry during fieldwork proved to be impossible.
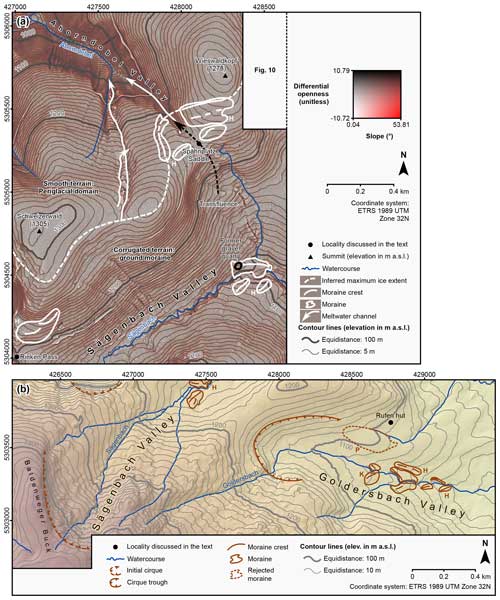
Figure 18(a) RRIM of Sägenbach Valley and the area around Spähnplatz Saddle. H – Hofmann (this study). Watercourses are according to LUBW (2022a). (b) Glacio-geomorphological map of the upper reach of Sägenbach Valley and Goldersbach Valley. H – Hofmann (this study). K – Klute (1911). P – Platz (1893). Watercourses are according to LUBW (2022a). See LGL (2015) for information on the DEM in the background.
We mapped for the first time a group of three sediment ridges at an elevation of 1140–1160 m a.s.l. further upstream. Figure 18b reveals that the northernmost ridge has led to a southward shift in the Sägenbach. Since these ridges constitute an arch, we interpreted them as ice-marginal moraines (Fig. 18b). These landforms indicate that a temporary period of ice-marginal stability punctuated the glacier retreat to the initial cirque in the uppermost reach of the valley.
With the aid of the RRIM and superimposed contour lines (Fig. 18a), we detected only faint sediment ridges a few metres high around Spähnplatz Saddle between Wieswaldkopf and Schweizerwald. Since other processes can be ruled out as possible explanations for the existence of the lobate ridges, we mapped them as ice-marginal moraines. The moraines probably formed at the margin of a former lobe of the Seebach glacier that once advanced over the saddle into the uppermost reach of Ahorndobel Valley. We hypothesise that the availability of meltwater favoured erosional downcutting north-west of Spähnplatz Saddle.
In accordance with the geological map of Wimmenauer et al. (1990), we interpreted the corrugated terrain on the south-western flank of Schweizerwald as ground moraine. During the interpretation of the RRIM, we noted that the slope angle markedly decreases at an elevation of about 1280–1290 m a.s.l. We interpret this step as a glacial trimline. The presence of two channels on the eastern flank of Schweizerwald (first mapped by Metz, 1985) supports the trimline interpretation. Figure 18a reveals that these morphologically prominent landforms extend from a saddle east of Schweizerwald towards Ahorndobel Valley. The preferred interpretation is that the margin of the Seebach glacier was once situated on the saddle. Meltwater flowed from the ice margin in a northerly direction towards Ahorndobel Valley and led to erosional downcutting.
Revisiting the area north-east of Rinken Pass resulted in the identification of a double-crested sediment ridge (Fig. 18a). We interpret this landform as an ice-marginal moraine that formed in a lateral position at the Seebach glacier.
4.2.8 Goldersbach Valley
This valley (Goldersbachtal) drains to the Goldersbach, the largest tributary of the Sägenbach. The Goldersbach rises on the north-eastern flank of Feldberg, flows through the V-shaped upper reach of Goldersbach Valley in an east-north-easterly direction, and tumbles down a step that separates the upper and lower reaches of this valley. Starting from an elevation of 1120 m a.s.l., the Goldersbach flows in an east-south-easterly direction. A relatively flat valley floor and gently sloping valley walls characterise the lower part of the valley (Fig. 18b). The Goldersbach eventually flows into the Sägenbach at an elevation of 970 m a.s.l. (Fig. 18b; LUBW, 2022a).
According to the geological map at the 1:25 000 scale of the State Geological Survey of Baden-Württemberg (Wimmenauer et al., 1990), this valley is devoid of moraines. The apparent lack of moraines conflicts with previous work (Platz, 1893; Klute, 1911; Meinig, 1980; Liehl, 1982) and with this study, indicating the presence of multiple ice-marginal moraines (Table 4). Meinig (1980) mapped a moraine north-west of the Sägenbach–Goldersbach confluence (Fig. 14b). Geomorphological mapping unveiled a terrace-like landform at this locality. We hypothesise that this landform is the remnant of a former outwash plain, connected to an ice-marginal position further up-valley. We mapped two sediment ridges at an elevation of 1030 m a.s.l. on the valley floor (marked with “H” in Fig. 18b). These ridges are subparallel to the Goldersbach and constitute an arch. Another sediment ridge lies 100 m further west. We observed a group of three sediment ridges further up-valley. The northernmost ridge is situated on the northern valley wall (marked with “H” in Fig. 18b), whilst the sediment ridges on the valley floor further south constitute an arch together with a sediment ridge south of the Goldersbach (Fig. 18b). Inspecting the sediment at a road cut on the southernmost ridge during field mapping revealed that this landform consists of a clast-rich diamict. The arcuate sediment ridges enclose a basin on the valley floor and have led to a southward displacement of the Goldersbach (Fig. 18b). Based on these observations, we conclude that these ridges are ice-marginal moraines. Revisiting the area further upstream where Klute (1911) mapped an ice-marginal moraine unveiled the presence of a prominent sediment ridge south of the Goldersbach and of a significantly smaller ridge north of this stream (marked with “K” in Fig. 18b). As these ridges resemble those further down-valley, we mapped them as ice-marginal moraines.
Platz (1893) described a moraine about 8 m high south of the Rufen hut (Rufenhütte). This study does not confirm the moraine interpretation by Platz (1893). Although we observed the west-north-west–east-south-east trending sediment ridge south of the hut (marked with “P” in Fig. 18b), we do not interpret this landform as an ice-marginal moraine. In fact, the smooth surface and its relatively large dimensions speak to streamlined subglacial till. Figure 18b shows that an unnamed stream rises north of this feature and flows along its northern end. This stream then flows towards the Sägenbach in a north-easterly direction. It appears plausible that the upper reach of this stream evolved from a subglacial channel, which bounds the ridge-like landform to the north.
Liehl (1982, his Table 3) mentioned an ice-marginal moraine at an elevation of 1143 m a.s.l. Unfortunately, he described neither the exact location of the moraine nor the landform itself. We could not identify a moraine at this elevation. Figure 18b shows that a semi-circular steep slope surrounds the upper end of the lower reach of the valley. In accordance with the cirque classification of Benn and Evans (2010, 311), we mapped this feature as a cirque trough.
To conclude, the moraines in the Goldersbach document four ice-marginal positions (Table 4) of a small glacier from the cirque trough, which was additionally fed by ice from the upper reach of the valley.
This holistic study allowed for the determination of the extent of the glaciation north-east of Feldberg in unprecedented detail. However, glacier-based climate reconstruction requires information on both the spatial and temporal evolution of glaciation. The establishment of a stratigraphy is “key to understanding the environmental record in sedimentary sequences” (Hughes et al., 2005, 85). Future work should, in the first instance, establish a stratigraphical model for the study region, i.e. assign ice-marginal landforms to stratigraphical units, with the ultimate goal of placing them in time.
Hughes et al. (2005) advocated the use of a morpho-lithostratigraphical framework for the ordering of landforms in formerly glaciated mountain ranges. This approach is based on the idea that landforms are assigned to lithostratigraphical units based on their morphostratigraphical position and lithological properties. However, it is foreseeable that these criteria will be insufficient for the correlation of ice-marginal landforms of isolated cirque glaciers, such as those in Bisten Valley or the Schuppenhörnle cirque. Geochronology must therefore come into play, calling for an extensive dating campaign. Prime targets for future dating studies are the terrace in the Tiefen area east of Breitnau, the kame terrace at the entrance to Langenordnach Valley, the glaciofluvial terraces in the lower reach of Höllen Valley, and the moraine complex at the entrance to Jos Valley. As the landforms' sediments contain sediments in the silt and sand fraction, luminescence methods could allow for the ascertaining of the timing of landform emplacement. Since quartz from the southern Black Forest has proven to be problematic for optically stimulated luminescence dating (Hofmann et al., 2021, 2024a), future studies should select potassium feldspar as a dosimeter. Beryllium-10 CRE dating of large boulders on moraines in the Wanne cirque, the Waldhof cirque, in the upper reach of Seebach Valley, and in the basin south-west of Breitnau is currently in progress. The resulting age datasets will enable us to evaluate the hypothesis that all mapped landforms formed during the Late Pleistocene glaciation, as was already assumed by Steinmann (1902), and to test whether the three-fold morpho-lithostratigraphy for the region north-west of Feldberg (see Hofmann, 2023b) is valid for the study region.
Obtaining age datasets will certainly greatly facilitate the construction of a stratigraphical model. Nevertheless, it is evident that due to the high costs associated with luminescence and CRE dating, future dating studies will only be able to determine the age of key landforms. In addition, multiple moraines, such as those in the surroundings of Hinterzarten, Titisee, and Bärental, lack large boulders for CRE dating. The landforms' sediments probably do not contain any suitable material for radiocarbon dating. Due to the absence of exposures, we could not assess whether these landforms contain sediments appropriate for luminescence dating. If successfully tested, reconstructing infrared-stimulated luminescence depth profiles in large clasts from these landforms could potentially allow us to ascertain their age (Rades et al., 2018, 2024). Given the foreseeable difficulties in determining the age of these landforms, the combination of different stratigraphic approaches is best-suited to order the mapped glacial record. This holistic approach will involve criteria such as the morphostratigraphical position or landform associations.
Lithological criteria will, most likely, not permit us to do so. Indeed, the sediments of the mapped landforms likely exhibit only minor lithological differences or may even be indistinguishable. This is in line with the lithostratigraphy of the State Geological Survey of Baden-Württemberg, which assigned all glacial deposits in the study region to the lithostratigraphical group of the Younger Black Forest Glacial Sediments (Jüngere Schwarzwald-Glazialsedimente; LGRB, 2022a). Unlike the Older Black Forest Glacial Sediments (Ältere Schwarzwald-Glazialsedimente), which are likely of Middle Pleistocene age (LGRB, 2022b), the younger Black Forest glacial sediments feature unweathered to slightly weathered clasts (LGRB, 2022a). Although the analysis of weathering rinds of clasts in glacial deposits might represent an easy means to differentiate between glacial deposits of Middle Pleistocene and Late Pleistocene age (Schreiner, 1986; LRGB, 2022a, b), this method will probably not work for deposits of Late Pleistocene age.
Once a sufficiently large body of age datasets is available, an integrated (holostratigraphical) model will be established, which will combine practices in morphostratigraphy, lithostratigraphy, and chronostratigraphy. In other words, this stratigraphy will consider the morphostratigraphical position of the mapped landforms, landform associations, the elevation, their age, and, if applicable, lithological properties. As recommended by Hughes et al. (2005), this model will be anchored to a continuous regional terrestrial record. As soon as the regional stratigraphy is established, glacier and ELA reconstruction will be undertaken. Adjusting independent summer temperature data to the ELAs will finally enable precipitation reconstruction based on the workflow of Hofmann et al. (2024a).
Due to the presence of numerous ice-marginal landforms, this research reinforces the idea of dynamic glaciation in the region north-east of Feldberg. Numerous mapped ice-marginal positions imply that the glaciers in the study region likely reacted very sensitively to changes in mass balance. Apart from the former cirque glaciers north-east of Feldberg, non-climatic factors probably exerted only a minor influence on ice masses, particularly on the coalescing Seebach glacier. We therefore affirm the conclusion of Klute (1911) that climatically induced stabilisation and re-advance phases were significantly involved in the genesis of the mapped landforms. Due to the presumably close relationship between climate and the glaciers' mass balance, the ice-marginal landforms north-east of Feldberg warrant further research for climate reconstruction. To achieve this goal, future work must, in the first instance, ascertain the age of the mapped landforms to construct a regional stratigraphy.
Five general implications for glacio-geomorphological studies emerge from this research:
-
Extensive literature mining prior to glacio-geomorphological mapping is essential for apprehending the glacial record. The cases of Sägenbach Valley and Goldersbach Valley illustrate that we would have certainly missed several ice-marginal landforms without a comprehensive review of previous work. If mapping or descriptions of glacial landforms are available for a region of interest, glacial geomorphologists should perform extensive literature mining, which ensures the greatest-possible accuracy during mapping.
-
Employing RRIMs for the mapping process greatly facilitates the detection of ice-marginal landforms and thus improves the quality of mapping. As already discussed by Chiba et al. (2008), RRIMs have the advantages of being independent of the direction of incident light and of displaying fine structures on relatively flat terrain, which are not easily recognisable in hillshades. Tools for geographic information systems such as Relief Visualization Toolbox by Kokalj and Somrak (2019), which is freely available, provide an easy means for the establishment of RRIMs.
-
The interpretation of high-resolution remote sensing data prior to field surveys allows for the direct targeting of candidates for ice-marginal landforms in the field, resulting in considerable time savings during mapping. Without the selection of potential targets for geomorphological mapping prior to field surveys, it would have certainly been impossible to efficiently map the relatively large study region.
-
Detailed glacio-geomorphological mapping allows for the resolution of discrepancies in the literature. Addressing these issues is key for dating campaigns. Without a clear understanding of the landforms being sampled, the interpretation of age datasets may pose major difficulties (see Winkler, 2018). This study largely confirmed previous mapping in the region north-east of Feldberg, and only a few ice-marginal landforms must be rejected. Hence, this study underlines the conclusion of Davies (2021, 228) that “dating campaigns should be strongly grounded in geomorphological mapping”.
-
Remote-sensing-data-based mapping of ice-marginal moraines should not and will not replace careful field mapping but will merely simplify it since field surveys and the inspection of outcrops are the only way to reliably distinguish moraines from wall-shaped erosional landforms. Without thorough field mapping, we would probably not have rejected the moraine at 1000 m a.s.l. mapped by Klute (1911) in the Sägenbach valley.
For rivers and lakes in Fig. 1, see https://www.eea.europa.eu/data-and-maps/data/wise-viewer-data-2007/wise-large-rivers-and-lakes/wise-large-rivers-and-lakes/at_download/file (European Environment Agency, 2008). See https://download.geoservice.dlr.de/TDM30_EDEM (DLR, 2023) for the DEM in Figs. 1 and 2. For watercourses and lakes in the study region, see http://www2.lubw.baden-wuerttemberg.de/public/abt2/dokablage/oac_77/moore01/412.htm (LUBW, 2022a) and http://www2.lubw.baden-wuerttemberg.de/public/abt2/dokablage/oac_77/moore01/450.htm (LUBW, 2022b), respectively. The DEM utilised for glacio-geomorphological mapping (LGL, 2015) is freely available in the Open GeoData Portal of the state of Baden-Württemberg (https://opengeodata.lgl-bw.de, last access: 10 January 2025). See Hofmann and Preusser (2024) for shapefiles of cirques, initial cirques, glaciofluvial terraces or fans and for the maximum ice extent, meltwater channels, moraine crests, moraines, and terraces.
FP and FMH designed the study and acquired funding. FMH undertook the fieldwork. FMH and FP interpreted the data. FMH drafted the manuscript and the figures. FMH and FP contributed to the final version of the paper.
The contact author has declared that neither of the authors has any competing interests.
Publisher’s note: Copernicus Publications remains neutral with regard to jurisdictional claims made in the text, published maps, institutional affiliations, or any other geographical representation in this paper. While Copernicus Publications makes every effort to include appropriate place names, the final responsibility lies with the authors.
The authors thank Claus Pfaffenberger and Thomas Schiedek for fruitful discussions during a joint visit to the former Kappel gravel quarry. Thanks are due to Onur Altinay for introducing RRIM to the mapping of moraines in the southern Black Forest. The forestry department of the Breisgau-Hochschwarzwald district kindly provided a forest access permit. Thanks are due to Matthias Geyer (Geotourist Freiburg) for providing oblique aerial photographs of the study region. The authors cordially thank the State Geological Survey of Baden-Württemberg (LRGB), particularly Ulrike Wieland-Schuster, for providing access to the lidar-based DEMs of the Baden-Württemberg State Agency for Spatial Information and Rural Development (LGL). Thanks are due to the reviewers (Levan Tielidze and an anonymous second reviewer), whose critical but constructive comments resulted in considerable improvements to the paper. This article is dedicated to Roland Meinig, who significantly advanced research on glaciation north-east of Feldberg.
This research was undertaken while Felix Martin Hofmann was in receipt of a PhD fellowship from Studienstiftung des Deutschen Volkes. This study was financially supported by the German Research Foundation (DFG) through the “Geometry, chronology, and dynamics of the last Pleistocene glaciation of the Black Forest” (https://www.vergletscherung-schwarzwald.de/, last access: 9 April 2024) and “Chronology of the glaciation of the southern Black Forest after the Late Pleistocene glaciation maximum” projects granted to Frank Preusser (project numbers 426333515 and 516126018, respectively).
This open-access publication was funded by the University of Freiburg.
This paper was edited by Hans von Suchodoletz and reviewed by Levan Tielidze and one anonymous referee.
Agassiz, L.: Auszug aus dem Briefe des Herrn Dr. Schimper Ueber die Eiszeit, an Pr. Louis Agassiz, Präsident der Gesellschaft, Verhandlungen der Allgemeinen Schweizerischen Gesellschaft für die Gesammten Naturwissenschaften, 22, 38, https://doi.org/10.5169/seals-89706, 1837.
Altınay, O., Sarıkaya, M. A., Çiner, A., Žebre, M., Stepišnik, U., Yıldırım, C., Yetemen, Ö., and Wilcken, K. M.: Cosmogenic 36Cl surface exposure dating of glacial landforms on Mt. Barla (SW Turkey), Geomorphology, 416, 108424, https://doi.org/10.1016/j.geomorph.2022.108424, 2022.
Bangert, V.: Zum Pleistozän des Blattes Lenzkirch (Nr. 8115), Südschwarzwald, Jh. Geol. Landesamt Baden-Württemberg, 2, 209–218, 1957.
Bangert, V., Schreiner, A., and Etzold, A.: Geologische Karte von Baden-Württemberg 1:25000. Blatt 8115 Lenzkirch, Landesvermessungsamt Baden-Württemberg, Stuttgart, 132 pp., 1992.
Batchelor, C. L., Margold, M., Krapp, M., Della Murton, K., Dalton, A. S., Gibbard, P. L., Stokes, C. R., Murton, J. B., and Manica, A.: The configuration of Northern Hemisphere ice sheets through the Quaternary, Nat. Commun., 10, 3713, https://doi.org/10.1038/s41467-019-11601-2, 2019.
Benn, D. I. and Evans, D. J. A.: Glaciers & Glaciation, 2nd ed., Routledge, London, New York, 802 pp., https://doi.org/10.4324/9780203785010, 2010.
Bolland, A., Rey, F., Gobet, E., Tinner, W., and Heiri, O.: Summer temperature development 18,000–14,000 cal. BP recorded by a new chironomid record from Burgäschisee, Swiss Plateau, Quaternary Sci. Rev., 243, 106484, https://doi.org/10.1016/j.quascirev.2020.106484, 2020.
Chandler, B. M., Lovell, H., Boston, C. M., Lukas, S., Barr, I. D., Benediktsson, Í. Ö., Benn, D. I., Clark, C. D., Darvill, C. M., Evans, D. J., Ewertowski, M. W., Loibl, D., Margold, M., Otto, J.-C., Roberts, D. H., Stokes, C. R., Storrar, R. D., and Stroeven, A. P.: Glacial geomorphological mapping: A review of approaches and frameworks for best practice, Earth-Sci. Rev., 185, 806–846, https://doi.org/10.1016/j.earscirev.2018.07.015, 2018.
Chandler, B. M. P. and Lukas, S.: Reconstruction of Loch Lomond Stadial (Younger Dryas) glaciers on Ben More Coigach, north-west Scotland, and implications for reconstructing palaeoclimate using small ice masses, J. Quaternary Sci., 32, 475–492, https://doi.org/10.1002/jqs.2941, 2017.
Chiba, T., Kaneta, S., and Suzuki, Y.: Red Relief Image Map: New Visualization Method for Three Dimensional Data, in: XXIst ISPRS Congress. Proceedings. Volume XXXVIII, Part B2, Commission II., Beijing, 1071–1076, https://www.isprs.org/proceedings/XXXVII/congress/2_pdf/11_ThS-6/08.pdf (last access: 10 January 2025), 2008.
Clark, C. D., Ely, J. C., Hindmarsh, R. C. A., Bradley, S., Ignéczi, A., Fabel, D., Ó Cofaigh, C., Chiverrell, R. C., Scourse, J., Benetti, S., Bradwell, T., Evans, D. J. A., Roberts, D. H., Burke, M., Callard, S. L., Medialdea, A., Saher, M., Small, D., Smedley, R. K., Gasson, E., Gregoire, L., Gandy, N., Hughes, A. L. C., Ballantyne, C., Bateman, M. D., Bigg, G. R., Doole, J., Dove, D., Duller, G. A. T., Jenkins, G. T. H., Livingstone, S. L., McCarron, S., Moreton, S., Pollard, D., Praeg, D., Sejrup, H. P., van Landeghem, K. J. J., and Wilson, P.: Growth and retreat of the last British–Irish Ice Sheet, 31 000 to 15 000 years ago: the BRITICE-CHRONO reconstruction, Boreas, 51, 699–758, https://doi.org/10.1111/bor.12594, 2022.
Cohen, K. M. and Gibbard, P. L.: Global chronostratigraphical correlation table for the last 2.7 million years, version 2019 QI-500, Quaternary Int., 500, 20–31, https://doi.org/10.1016/j.quaint.2019.03.009, 2019.
Davies, B.: Dating Glacial Landforms I: Archival, incremental, relative dating techniques and age-equivalent stratigraphic makers, in: Cryospheric Geomorphology, edited by: Haritashya, U., Elsevier, 225–248, https://doi.org/10.1016/B978-0-12-818234-5.00042-0, 2021.
Di Costanzo, H. and Hofmann, F. M.: Le retrait du glacier du Drac Blanc (massif des Écrins, Alpes françaises), Mediterranée, Paléo-environnements, géoarchéologie, géohistoire, 2016, https://doi.org/10.4000/mediterranee.7796, 2016.
DLR: TanDEM-X 30 m Edited DEM, DLR [data set], https://download.geoservice.dlr.de/TDM30_EDEM (last access: 12 December 2023), 2023.
Ehlers, J., Gibbard, P. L., and Hughes, P. D. (Eds.): Quaternary Glaciations – Extent and Chronology.: A Closer Look, Developments in Quaternary Sciences, 15, Elsevier, Amsterdam, Oxford, ISBN 978-0-444-53447-7, 2011.
Engel, Z., Traczyk, A., Braucher, R., Woronko, B., and Křížek, M.: Use of 10Be exposure ages and Schmidt hammer data for correlation of moraines in the Krkonoše Mountains, Poland/Czech Republic, Z. Geomorphol., 55, 175–196, https://doi.org/10.1127/0372-8854/2011/0055-0036, 2011.
Engel, Z., Braucher, R., Traczyk, A., Léanni, L., and ASTER Team: 10Be exposure age chronology of the last glaciation in the Krkonoše Mountains, Central Europe, Geomorphology, 206, 107–121, https://doi.org/10.1016/j.geomorph.2013.10.003, 2014.
Environmental Systems Research Institute: ArcGIS Desktop [software], Environmental Systems Research Institute, https://www.esri.com/de-de/arcgis/products/arcgis-desktop/overview (last access: 10 January 2025), 2020.
Erb, L.: Die Geologie des Feldbergs, in: Der Feldberg im Schwarzwald, edited by: Müller, K., L., Bielefelds Verlag KG, Freiburg i. Br., 22–96, 1948.
European Environment Agency: WISE Large rivers and lakes, European Environment Agency [data set], https://www.eea.europa.eu/data-and-maps/data/wise-viewer-data-2007/wise-large-rivers-and-lakes/wise-large-rivers-and-lakes/at_download/file (last access: 12 December 2023), 2008.
Geyer, M., Nitsch, E., and Simon, T.: O. F. Geyer/M. P. Gwinner: Geologie von Baden-Württemberg, 5nd Ed., Schweizerbart, Stuttgart, 627 pp., ISBN 978-3-510-65267-9, 2011.
Giermann, G.: Die würmeiszeitliche Vergletscherung des Schauinsland-Trubelsmattkopf-Knöpflesbrunnen-Massivs (südlicher Schwarzwald), Ber. Naturf. Ges. Freiburg i. Br., 54, 197–207, https://www.zobodat.at/pdf/Berichte-naturf-Ges-Freiburg-Br_54_0197-0207.pdf (last access: 13 January 2025), 1964.
González, C., Bachmann, M., Bueso-Bello, J.-L., Rizzoli, P., and Zink, M.: A Fully Automatic Algorithm for Editing the TanDEM-X Global DEM, Remote Sens.-Basel, 12, 3961, https://doi.org/10.3390/rs12233961, 2020.
Graf, A., Akçar, N., Ivy-Ochs, S., Strasky, S., Kubik, P. W., Christl, M., Burkhard, M., Wieler, R., and Schlüchter, C.: Multiple advances of Alpine glaciers into the Jura Mountains in the Northwestern Switzerland, Swiss J. Geosci., 108, 225–238, https://doi.org/10.1007/s00015-015-0195-y, 2015.
Guiot, J., Pons, A., Beaulieu, J. L. de, and Reille, M.: A 140,000-year continental climate reconstruction from two European pollen records, Nature, 338, 309–313, https://doi.org/10.1038/338309a0, 1989.
Haase, E.: Der “Falkaustand” - ein Sonderfall oder eine gesetzmäßige Erscheinung im Bild der Südschwarzwälder Vergletscherung? Ber. Naturf. Ges. Freiburg i. Br., 58, 135–158, https://www.zobodat.at/pdf/Berichte-naturf-Ges-Freiburg-Br_58_0135-0158.pdf (last access: 13 January 2025), 1968.
Hantke, R. and Rahm, G.: Das frühe Spätglazial in den Quellästen der Alb (Südlicher Schwarzwald), Vierteljahresschrift der Naturforschenden Gesellschaft in Zürich, 121, 293–299, 1976.
Hebestreit, C., Schiedek, T., Bauer, M., and Pfaffenberger, C.: Zeitmarken der Wutacheintiefung – Terrassenkorrelation, Terrassenstratigraphie und Kalktuffe, Jber. Mitt. Oberrhein. Geol. Ver., N.F., 75, 291–312, https://doi.org/10.1127/jmogv/75/1993/291, 1993.
Heiri, O. and Millet, L.: Reconstruction of Late Glacial summer temperatures from chironomid assemblages in Lac Lautrey (Jura, France), J. Quaternary Sci., 20, 33–44, https://doi.org/10.1002/jqs.895, 2005.
Heiri, O., Koinig, K. A., Spötl, C., Barrett, S., Brauer, A., Drescher-Schneider, R., Gaar, D., Ivy-Ochs, S., Kerschner, H., Luetscher, M., Moran, A., Nicolussi, K., Preusser, F., Schmidt, R., Schoeneich, P., Schwörer, C., Sprafke, T., Terhorst, B., and Tinner, W.: Palaeoclimate records 60–8 ka in the Austrian and Swiss Alps and their forelands, Quaternary Sci. Rev., 106, 186–205, https://doi.org/10.1016/j.quascirev.2014.05.021, 2014.
Hemmerle, H., May, J.-H., and Preusser, F.: Übersicht über die pleistozänen Vergletscherungen des Schwarzwaldes, Ber. Naturf. Ges. Freiburg i. Br., 106, 31–67, https://www.zobodat.at/pdf/Berichte-naturf-Ges-Freiburg-Br_106_0031-0067.pdf (last access: 13 January 2025), 2016.
Hofmann, F. M.: Geometry, chronology and dynamics of the last Pleistocene glaciation of the Black Forest, E&G Quaternary Sci. J., 72, 235–237, https://doi.org/10.5194/egqsj-72-235-2023, 2023a.
Hofmann, F. M.: Geometry, Chronology and Dynamics of the last Pleistocene glaciation of the Black Forest, Doctoral thesis, Faculty of Environment and Natural Resources, University of Freiburg, Germany, https://doi.org/10.6094/UNIFR/241069, 2023b.
Hofmann, F. M. and Konold, W.: Landschaftsgeschichte des oberen Seebachtals, Südschwarzwald (Exkursion D am 14. September 2023), Jber. Mitt. Oberrhein. Geol. Ver., N.F., 105, 63–89, https://doi.org/10.1127/jmogv/105/0004, 2023.
Hofmann, F. M. and Preusser, F.: Glacial landforms NE of Feldberg (Black Forest, SW Germany), FreiDok plus, [data set], https://doi.org/10.6094/UNIFR/256998, 2024.
Hofmann, F. M., Rambeau, C., Gegg, L., Schulz, M., Steiner, M., Fülling, A., Léanni, L., Preusser, F., and ASTER Team: Regional beryllium-10 production rate for the mid-elevation mountainous regions in central Europe, deduced from a multi-method study of moraines and lake sediments in the Black Forest, Geochronology, 6, 147–174, https://doi.org/10.5194/gchron-6-147-2024, 2024a.
Hofmann, F. M., Steiner, M., Hergarten, S., ASTER Team, and Preusser, F.: Limitations of precipitation reconstructions using equilibrium line altitudes exemplified for former glaciers in the Southern Black Forest, Central Europe, Quaternary Res., 117, 135–159, https://doi.org/10.1017/qua.2023.53, 2024b.
Hofmann, F. M., Preusser, F., Schimmelpfennig, I., Léanni, L., and ASTER Team: Late Pleistocene glaciation history of the southern Black Forest, Germany: 10Be cosmic-ray exposure dating and equilibrium line altitude reconstructions in Sankt Wilhelmer Tal, J. Quaternary Sci., 37, 688–706, https://doi.org/10.1002/jqs.3407, 2022.
Hofmann, F. M., Fülling, A., and Preusser, F.: Challenges in luminescence dating of the last glaciation maximum in the southern Black Forest, Germany, Ancient TL, 39, P.5-7, https://doi.org/10.6094/UNIFR/242353, 2021.
Hofmann, F. M., Rauscher, F., McCreary, W., Bischoff, J.-P., and Preusser, F.: Revisiting Late Pleistocene glacier dynamics north-west of the Feldberg, southern Black Forest, Germany, E&G Quaternary Sci. J., 69, 61–87, https://doi.org/10.5194/egqsj-69-61-2020, 2020.
Hofmann, F. M., Alexanderson, H., Schoeneich, P., Mertes, J. R., Léanni, L., and ASTER Team: Post-Last Glacial Maximum glacier fluctuations in the southern Écrins massif (westernmost Alps): insights from 10Be cosmic ray exposure dating, Boreas, 48, 1019–1041, https://doi.org/10.1111/bor.12405, 2019.
Hughes, A. L. C., Gyllencreutz, R., Lohne, Ø. S., Mangerud, J., and Svendsen, J. I.: The last Eurasian ice sheets – a chronological database and time-slice reconstruction, DATED-1, Boreas, 45, 1–45, https://doi.org/10.1111/bor.12142, 2016.
Hughes, P. D. and Gibbard, P. L.: A stratigraphical basis for the Last Glacial Maximum (LGM), Quaternary Intern., 383, 174–185, https://doi.org/10.1016/j.quaint.2014.06.006, 2015.
Hughes, P. D., Gibbard, P. L., and Woodward, J. C.: Quaternary glacial records in mountain regions: A formal stratigraphical approach, Episodes, 28, 85–92, https://doi.org/10.18814/epiiugs/2005/v28i2/002, 2005.
Ivy-Ochs, S.: Glacier variations in the European Alps at the end of the last glaciation, Cuadernos de Investigación Geográfica, 41, 295–315, https://doi.org/10.18172/cig.2750, 2015.
Ivy-Ochs, S., Kerschner, H., Reuther, A., Preusser, F., Heine, K., Maisch, M., Kubik, P. W., and Schlüchter, C.: Chronology of the last glacial cycle in the European Alps, J. Quaternary Sci., 23, 559–573, https://doi.org/10.1002/jqs.1202, 2008.
Klute, F.: Die Schneereste des Schwarzwaldes im Frühsommer und die Beziehungen ihrer Lage zu den Stellen ehemaliger Vergletscherung, Ber. Naturf. Ges. Freiburg i. Br., 19, 61–116, https://www.zobodat.at/pdf/Berichte-naturf-Ges-Freiburg-Br_19_0061-0116.pdf (last access: 13 January 2025), 1911.
Kokalj, Ž. and Somrak, M.: Why Not a Single Image? Combining Visualizations to Facilitate Fieldwork and On-Screen Mapping, Remote Sens.-Basel, 11, 747, https://doi.org/10.3390/rs11070747, 2019.
Köse, O., Sarıkaya, M. A., Çiner, A., and Yıldırım, C.: Glacial geomorphology of the Aladağlar, central Taurus Mountains, Turkey, J. Maps, 17, 101–113, https://doi.org/10.1080/17445647.2021.1883137, 2021.
Köse, O., Sarıkaya, M. A., Çiner, A., Candaş, A., Yıldırım, C., and Wilcken, K. M.: Reconstruction of Last Glacial Maximum glaciers and palaeoclimate in the central Taurus Range, Mt. Karanfil, of the Eastern Mediterranean, Quaternary Sci. Rev., 291, 107656, https://doi.org/10.1016/j.quascirev.2022.107656, 2022.
Krause, D. and Margold, M.: Glacial geomorphology of the Šumava / Bayerischer Wald mountains, Central Europe, J. Maps, 15, 719–725, https://doi.org/10.1080/17445647.2019.1661881, 2019.
Lang, G.: Seen und Moore des Schwarzwaldes als Zeugen spätglazialen und holozänen Vegetationswandels. Stratigraphische, pollenanalytische und großrestanalytische Untersuchungen, Andrias, 16, Karlsruhe, 160 pp., https://www.zobodat.at/pdf/Andrias_16_0003-0160.pdf (last access: 10 January 2025), 2005.
Lang, G., Merkt, J., and Streif, H.: Spätglazialer Gletscherrückzug und See- und Moorentwicklung im Südschwarzwald, Südwestdeutschland, Dissertationes Botanicae, 72 (Festschrift Welten), 213–234, 1984.
Leser, H. and Metz, B.: Vergletscherungen im Hochschwarzwald, Berliner Geogr. Abh., 47, 155–175, 1988.
Levy, F.: Das System des Feldberggletschers im hohen Schwarzwald, Mitteilungen der Geographischen Gesellschaft in München, 7, 133–137, 1912.
LGL: DGM aus ALS_1, LGL, https://opengeodata.lgl-bw.de/#/(sidenav:product/3) (last access: 10 January 2025), 2015.
LGL: Open GeoData Portal, LGL, https://opengeodata.lgl-bw.de/#/ (last access: 10 January 2025), 2024.
LGRB (Landesamt für Geologie, Rohstoffe und Bergbau Baden-Württemberg): Geologische Karte von Baden-Württemberg 1 50 000 (GeoLa), LGRB, Freiburg, https://maps.lgrb-bw.de/?view=lgrb_geola_geo (last access: 10 January 2025), 2013.
LGRB: Geologische Karte von Baden-Württemberg (GK-BW). Geologische Generallegendeneinheiten. Jüngere Schwarzwald-Glazialsedimente (qpSj), https://media.lgrb-bw.de/link/geo/geo_gle_548.pdf (last access: 14 July 2023), 2022a.
LGRB: Geologische Karte von Baden-Württemberg (GK-BW). Geologische Generallegendeneinheiten. Ältere Schwarzwald-Glazialsedimente (qpSa), https://media.lgrb-bw.de/link/geo/geo_gle_555.pdf (last access: 15 July 2023), 2022b.
Liehl, E.: Exkursion ins Höllentalgebiet, Breitnau und Hinterzarten, Mitteilungen der Geographischen Fachschaft der Universität Freiburg i. Br., 2, 134–142, 1970.
Liehl, E.: Geländeformen des Feldberggebiets, in: Landformen im Kartenbild. Topographisch-Geomorphologische Kartenproben 1: 25 000. Gruppe II: Mittelgebirge. Gefalteter Unterbau, stellenweise überlagert. Kartenprobe 6: Kristalliner Schwarzwald mit Karen und Moränen, Feldberg, edited by: Hofmann, W. and Louis, H., Westermann, Braunschweig, 8–16, 1975.
Liehl, E.: Der Schwarzwald in der letzten Eiszeit. Ein wissenschaftsgeschichtlicher Rückblick, in: Der Schwarzwald. Beiträge zur Landeskunde, edited by: Liehl, E. and Sick, W.-D., Konkordia, Bühl, 9–35 pp., 1980.
Liehl, E.: Landschaftsgeschichte des Feldberggebietes, in: Der Feldberg im Schwarzwald: subalpine Insel im Mittelgebirge, edited by: Landesanstalt für Umweltschutz Baden-Württemberg and Institut für Ökologie und Naturschutz, Karlsruhe, 13–147, 1982.
Lisiecki, L. E. and Raymo, M. E.: A Pliocene-Pleistocene stack of 57 globally distributed benthic δ18O records, Paleoceanography, 20, PA1003, https://doi.org/10.1029/2004PA001071, 2005.
Lotter, A. F., Heiri, O., Brooks, S., van Leeuwen, J. F., Eicher, U., and Ammann, B.: Rapid summer temperature changes during Termination 1a: high-resolution multi-proxy climate reconstructions from Gerzensee (Switzerland), Quaternary Sci. Rev., 36, 103–113, https://doi.org/10.1016/j.quascirev.2010.06.022, 2012.
LUBW: Eschengrundmoos, LUBW, http://www2.lubw.baden-wuerttemberg.de/public/abt2/dokablage/oac_77/moore01/412.htm (last access: 16 January 2024), 2006a.
LUBW: Hinterzartener Moor, LUBW, http://www2.lubw.baden-wuerttemberg.de/public/abt2/dokablage/oac_77/moore01/450.htm (last access: 16 January 2024), 2006b.
LUBW: Fließgewässer (AWGN), LUBW [data set], https://rips-datenlink.lubw.de/UDO_download/Fliessgewaessernetz.zip (last access: 8 September 2023), 2022a.
LUBW: Stehendes Gewässer (AWGN), LUBW [data set], https://rips-datenlink.lubw.de/UDO_download/StehendeGewaesser.zip (last access: 8 September 2023), 2022b.
Magny, M., Aalbersberg, G., Bégeot, C., Benoit-Ruffaldi, P., Bossuet, G., Disnar, J.-R., Heiri, O., Laggoun-Defarge, F., Mazier, F., Millet, L., Peyron, O., Vannière, B., and Walter-Simmonet, V.: Environmental and climatic changes in the Jura mountains (eastern France) during the Lateglacial–Holocene transition: a multi-proxy record from Lake Lautrey, Quaternary Sci. Rev., 25, 414–445, https://doi.org/10.1016/j.quascirev.2005.02.005, 2006.
Magny, M., Guiot, J., and Schoellammer, P.: Quantitative Reconstruction of Younger Dryas to Mid-Holocene Paleoclimates at Le Locle, Swiss Jura, Using Pollen and Lake-Level Data, Quaternary Res., 56, 170–180, https://doi.org/10.1006/qres.2001.2257, 2001.
Meinig, R.: Untersuchung der glazialgeologischen Verhältnisse im Raum Breitnau – Steig – Löffeltal (Südschwarzwald), Unpublished diploma thesis, Institute of Geology and Palaeontology, University of Freiburg, Freiburg, 78 pp., 1962.
Meinig, R.: Die würmeiszeitliche Vergletscherung im Gebiet Breitnau-Hinterzarten-Neustadt (Schwarzwald), Dissertation, Institute of Geology and Palaeontology, University of Freiburg, Freiburg, 166 pp., 1966.
Meinig, R.: Die würmeiszeitlichen Moränen in Alpersbach und im Bistental westlich von Hinterzarten/Südschwarzwald, Ber. Naturf. Ges. Freiburg i. Br., 67, 189–201, https://www.zobodat.at/pdf/Berichte-naturf-Ges-Freiburg-Br_67_0189-0201.pdf (last access: 10 January 2025), 1977.
Meinig, R.: Halte und Eisrandbildungen des würmzeitlichen Bärental-Gletschers, Südschwarzwald, in: Spätglaziale und Postglaziale Gletscherschwankungen: Glazial- und Periglazialformen, Trier, 1980, 257–282, 1980.
Mentlík, P., Engel, Z., Braucher, R., and Léanni, L.: Chronology of the Late Weichselian glaciation in the Bohemian Forest in Central Europe, Quaternary Sci. Rev., 65, 120–128, https://doi.org/10.1016/j.quascirev.2013.01.020, 2013.
Mercier, J.-L., Bourlès, D. L., Kalvoda, J., Braucher, R., and Paschen, A.: Deglaciation of the Vosges dated using 10Be, Acta Universitatis Carolinae Geographica, 2, 139–155, 1999.
Mercier, J.-L., Kalvoda, J., Bourlès, D. L., Braucher, R., and Engel, Z.: Preliminary results of 10Be dating of glacial landscape in the Giant Mountains, Acta Universitatis Carolinae Geographica, Supplementum, 35, 157–170, 2000.
Merkt, J.: Exkursionspunkt: “Feldsee”. Bohrung Feldsee-Mitte, in: Deutsche Quartärvereinigung. 22. wissenschaftliche Tagung in Freiburg i. Br.: Exkursionsführer I Südschwarzwald, Oberschwaben/Bodensee, südliches Oberrheingebiet, edited by: Schreiner, A. and Metz, B., Deutsche Quartärvereinigung, Hannover, 39–41, 1985.
Metz, B.: Geomorphologische Karte 1:25 000 der Bundesrepublik Deutschland. GMK 25 Blatt 21. 8114 Feldberg, Geo Center, Stuttgart, 1985.
Metz, B. and Saurer, H.: Geomorphologie und Landschaftsentwicklung, in: Der Feldberg: subalpine Insel im Schwarzwald, edited by: Regierungspräsidium Freiburg, Jan Thorbecke Verlag der Schwabenverlag AG, Ostfildern, 14–62, 2012.
Neuschel, P.: Geologische Untersuchung des Spriegelsbachtales und der Umgebung (westlich Neustadt/ Schwarzwald), Unpublished diploma thesis, University of Freiburg, 65 pp., https://doi.org/10.1016/B978-0-323-91899-2.00002-4, 1987.
Palacios, D., Hughes, P. D., Sánchez-Goñi, M. F., García Ruiz, J. M., and Andrés, N.: The terminations of the glacial cycles, in: European Glacial Landscapes. The last deglaciation, edited by: Palacios, D., Hughes, P. D., García Ruiz, J. M., and Andrés, N., Elsevier, Amsterdam, Oxford, Cambridge, 11–24, https://www.zobodat.at/pdf/Berichte-naturf-Ges-Freiburg-Br_53_0005-0061.pdf (last access: 10 January 2025), 2023.
Peyron, O., Bégeot, C., Brewer, S., Heiri, O., Magny, M., Millet, L., Ruffaldi, P., van Campo, E., and Yu, G.: Late-Glacial climatic changes in Eastern France (Lake Lautrey) from pollen, lake-levels, and chironomids, Quaternary Res., 64, 197–211, https://doi.org/10.1016/j.yqres.2005.01.006, 2005.
Pfannenstiel, M. and Rahm, G.: Die Vergletscherung des Wutachtales während der Rißeiszeit, Ber. Naturf. Ges. Freiburg i. Br., 53, 5–61, QGIS Development Team, https://www.zobodat.at/pdf/Berichte-naturf-Ges-Freiburg-Br_53_0005-0061.pdf (last access: 13 January 2025), 1963.
Platz, P.: Die Glazialbildungen des Schwarzwaldes, Mitt. d. Bad. Geol. Landesanst., 2, 837–924, 1893.
Preusser, F., Graf, H. R., Keller, O., Krayss, E., and Schlüchter, C.: Quaternary glaciation history of northern Switzerland, E&G Quaternary Sci. J., 60, 21, https://doi.org/10.3285/eg.60.2-3.06, 2011.
QGIS Development Team: QGIS Geographic Information System, [software], https://www.qgis.org/ (last access: 10 January 2025), 2023.
Raab, T. and Völkel, J.: Late Pleistocene glaciation of the Kleiner Arbersee area in the Bavarian Forest, south Germany, Quaternary Sci. Rev., 22, 581–593, https://doi.org/10.1016/S0277-3791(02)00090-2, 2003.
Rades, E. F., Sohbati, R., Lüthgens, C., Jain, M., and Murray, A. S.: First luminescence-depth profiles from boulders from moraine deposits: Insights into glaciation chronology and transport dynamics in Malta valley, Austria, Radiat. Meas., 120, 281–289, https://doi.org/10.1016/j.radmeas.2018.08.011, 2018.
Rades, E. F., Sohbati, R., Alexanderson, H., Jain, M., and Murray, A. S.: Exploring the potential of rock surface luminescence from glacial sediments: dating and transport history, Boreas, https://doi.org/10.1111/bor.12648, 2024.
Rahm, G.: Die Vergletscherung des Belchengebietes (Südschwarzwald) zur Würmeiszeit, E&G Quaternary Sci. J., 37, 31–40, https://doi.org/10.3285/eg.37.1.03, 1987.
Ramsay, A. C.: On the Glacial Origin of certain Lakes in Switzerland, the Black Forest, Great Britain, Sweden, North America, and elsewhere, Q. J. Geol. Soc. London, 18, 185–204, 1862.
Rea, B. R., Pellitero, R., Spagnolo, M., Hughes, P., Ivy-Ochs, S., Renssen, H., Ribolini, A., Bakke, J., Lukas, S., and Braithwaite, R. J.: Atmospheric circulation over Europe during the Younger Dryas, Sci. Adv., 6, eaba4844, https://doi.org/10.1126/sciadv.aba4844, 2020.
Reinig, F., Wacker, L., Jöris, O., Oppenheimer, C., Guidobaldi, G., Nievergelt, D., Adolphi, F., Cherubini, P., Engels, S., Esper, J., Land, A., Lane, C., Pfanz, H., Remmele, S., Sigl, M., Sookdeo, A., and Büntgen, U.: Precise date for the Laacher See eruption synchronizes the Younger Dryas, Nature, 595, 66–69, https://doi.org/10.1038/s41586-021-03608-x, 2021.
Reuther, A. U.: Surface exposure dating of glacial deposits from the last glacial cycle. Evidence from the Eastern Alps, the Bavarian Forest, the Southern Carpathians and the Altai Mountains, Relief, Boden, Paläoklima, 21, Borntraeger, Berlin, Stuttgart, 213 pp., ISBN 978-3-443-09021-0, 2007.
Sawatzki, G.: Geologische Karte 1:25 000 von Baden-Württemberg. Erläuterungen zu Blatt 8214 St. Blasien, Landesvermessungsamt Baden-Württemberg, Stuttgart, 1992.
Schalch, F.: Geologische Specialkarte des Grossherzogtums Baden. Blatt 119 Neustadt., Großherzogliche Badische Geologische Landesanstalt, Heidelberg, 1903.
Schiedek, T. and Pfaffenberger, C.: Akkumulations- und Erosionsphasen im Niederterrassen-Kieskörper des Gutachtales, in: Eintiefungsgeschichte und Stoffaustrag im Wutachgebiet (SW-Deutschland), edited by: Einsele, G. and Ricken, W., Institut für Geowissenschaften der Universität Tübingen, Tübingen, 58–65, 1993.
Schreiner, A.: Quartär, in: Geologische Karte von Baden-Württemberg 1:25 000. Erläuterungen zu Blatt 8114 Feldberg, edited by: Geologisches Landesamt Baden-Württemberg, Landesvermessungsamt Baden-Württemberg, Stuttgart, 67–95, 1981.
Schreiner, A.: Neuere Untersuchungen zur Rißeiszeit im Wutachgebiet (Südostschwarzwald), Jh. Geol. Landesamt Baden-Württemberg, 28, 221–244, 1986.
Schreiner, A.: Quartär, in: Geologische Karte 1:25 000 von Baden-Württemberg. Erläuterungen zu Blatt 8114 Feldberg, 2nd ed., edited by: Geologisches Landesamt Baden-Württemberg, Landesvermessungsamt Baden-Württemberg, Stuttgart, 67–95, 1990.
Schreiner, A.: Quartär, in: Geologische Karte von Baden-Württemberg 1:25 000. Erläuterungen zum Blatt 8014 Hinterzarten, edited by: Wimmenauer, W. and Schreiner, A., Landesvermessungsamt Baden-Württemberg, Stuttgart, 97–141, https://doi.org/10.1016/S0277-3791(02)00090-2, 1999a.
Schreiner, A.: Schichtenverzeichnisse, in: Geologische Karte von Baden-Württemberg 1:25 000. Erläuterungen zum Blatt 8014 Hinterzarten, edited by: Wimmenauer, W. and Schreiner, A., Landesvermessungsamt Baden-Württemberg, Stuttgart, 172–177, 1999b.
Schreiner, A. and Wimmenauer, W.: Geologische Karte von Baden-Württemberg 1:25 000. Blatt 8014 Hinterzarten, Landesamt für Geologie, Rohstoffe und Bergbau, Freiburg im Breisgau, 189 pp., 1999.
Schrepfer, H.: Zur Kenntnis der Eiszeit im Wutachgebiet, Mitteilungen des Badischen Landesvereins für Naturkunde und Naturschutz in Freiburg i. Br., 1, 469–473, https://www.zobodat.at/pdf/Mitt-Bad-Landesver-Natkde-Natschutz-Freiburg_NF_1_0469-0473.pdf (last access: 10 January 2025), 1925.
Steinmann, G.: Die Spuren der letzten Eiszeit im hohen Schwarzwalde, in: Festprogramm seiner Königlichen Hoheit Großherzog Friedrich zur Feier des siebzigsten Geburtstages, edited by: Albrecht-Ludwigs-Universität zu Freiburg, J. C. B. Mohr (Paul Siebeck), Freiburg, Leipzig, 187–226, https://doi.org/10.11588/diglit.49386{#}0201, 1896.
Steinmann, G.: Die Bildungen der letzten Eiszeit im Bereiche des alten Wutachgebiets, Jber. Mitt. oberrhein. geol. Ver., 35, 16–23, 1902.
Tielidze, L. G., Charton, J., Jomelli, V., and Solomina, O. N.: Glacial geomorphology of the Notsarula and Chanchakhi river valleys, Georgian Caucasus. J. Maps, 19, 2261490, https://doi.org/10.1080/17445647.2023.2261490, 2023.
Valenti, C., Rinterknecht, V., Koehler, H., Griselin, S., Audouard, L., Gehres, B., Couette, P.-O., and Équipe ASTER: Déglaciation des Vosges gréseuses et reconstructions paléoclimatiques à partir de datations par le béryllium-10 produit in situ, Quaternaire, 35, https://doi.org/10.4000/quaternaire.19147, 2024.
Walchner, F. A.: Handbuch der Geognosie zum Gebrauche bei seinen Vorlesungen und zum Selbststudium mit besonderer Berücksichtigung der geognostischen Verhältnisse des Grossherzogtums Baden, Christian Theodor Groos, Karlsruhe, 1120 pp., 1846.
Wimmenauer, W. and Hüttner, R.: Geologische Karte von Baden-Württemberg 1:25000. Blatt 8013 Freiburg., Geologisches Landesamt in Baden-Württemberg, Stuttgart, 159 pp., 1968.
Wimmenauer, W., Liehl, E., and Schreiner, A.: Geologische Karte von Baden-Württemberg 1:25 000. Blatt 8114 Feldberg, Landesvermessungsamt Baden-Württemberg, Stuttgart, 1981.
Wimmenauer, W., Liehl, E., and Schreiner, A.: Geologische Karte von Baden-Württemberg 1:25 000. Blatt 8114 Feldberg, Landesvermessungsamt Baden-Württemberg, Stuttgart, 1990.
Winkler, S.: Investigating Holocene mountain glaciations: a plea for the supremacy of glacial geomorphology when reconstructing glacier chronologies, Erdkunde, 72, 215–234, https://doi.org/10.3112/erdkunde.2018.03.04, 2018.
Yokoyama, R., Shirasawa, M., and Pike, R. J.: Visualizing topography by openness: A new application of image processing to digital elevation models, Photogramm. Eng. Rem. S., 68, 257–266, 2002.
Ziegler, P. A.: European Cenozoic rift system, Tectonophysics, 208, 91–111, https://doi.org/10.1016/0040-1951(92)90338-7, 1992.
Zienert, A.: Die Würm-Vereisung und ihre Rückzugsstadien im Westteil des Hochschwarzwaldes, Z. Geomorphol., 17, 359–366, https://doi.org/10.1127/zfg/17/1973/359, 1973.
- How to cite
- Abstract
- Kurzfassung
- Introduction
- Regional setting and previous work
- Methods
- Results and discussion
- Towards an integrated stratigraphical framework and climate reconstruction
- Conclusions
- Data availability
- Author contributions
- Competing interests
- Disclaimer
- Acknowledgements
- Financial support
- Review statement
- References
- How to cite
- Abstract
- Kurzfassung
- Introduction
- Regional setting and previous work
- Methods
- Results and discussion
- Towards an integrated stratigraphical framework and climate reconstruction
- Conclusions
- Data availability
- Author contributions
- Competing interests
- Disclaimer
- Acknowledgements
- Financial support
- Review statement
- References