the Creative Commons Attribution 4.0 License.
the Creative Commons Attribution 4.0 License.
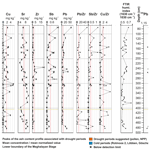
Anthropogenic and climate signals in late-Holocene peat layers of an ombrotrophic bog in the Styrian Enns valley (Austrian Alps)
Wolfgang Knierzinger
Ruth Drescher-Schneider
Klaus-Holger Knorr
Simon Drollinger
Andreas Limbeck
Lukas Brunnbauer
Felix Horak
Daniela Festi
Michael Wagreich
Using peat bogs as palaeoenvironmental archives is a well-established practice for reconstructing changing climate and anthropogenic activity in the past. In this paper, we present multi-proxy analyses (element geochemistry, pollen, non-pollen palynomorphs, stable Pb isotopes, humification, ash content) of a 500 cm long, 14C-dated peat core covering the past ∼5000 years from the ombrotrophic Pürgschachen Moor in the Styrian Enns valley (Austrian Alps). Early indications of low settlement and agricultural activity date to ∼2900 cal BCE. An early enrichment of Cu was found in peat layers corresponding to the late Copper Age (∼2500 cal BCE). These enrichments are attributed to Cu mining activities in the Eisenerz Alps. More pronounced increases in cultural indicators (cultivated plants, shrubs, herbs, charcoal) in the pollen record and enrichments of trace metals suggest significant human impact in the vicinity of Pürgschachen Moor in the middle Bronze Age (∼1450–1250 cal BCE), in the late Bronze Age (∼1050–800 cal BCE) and in the period of the late La Tène culture (∼300 cal BCE–1 cal CE). The greater part of the Iron Age and the Roman imperial period are each characterized by a general decline in anthropogenic indicators compared to previous periods. Distinct enrichments of Pb and Sb in the sample that corresponds to ∼900 cal CE are attributed to medieval siderite mining activity in the immediate vicinity of Pürgschachen Moor. The results of this interdisciplinary study provide evidence that strong, climate-controlled interrelations exist between the pollen record, the humification degree and the ash content in an ombrotrophic environment. Human activity, in contrast, is mainly reflected in the pollen record and by enrichments of heavy metals. The study indicates a dry period in the region of the bog around ∼1950 cal BCE.
Hochmoore eignen sich zur Rekonstruktion des Paläoklimas und anthropogener Aktivität in der Vergangenheit. In der vorliegenden Arbeit werden Multiproxy-Analysen (Elementchemie, Pollen, Mikrofossilien, stabile Pb-Isotope, Humifizierung, Aschegehalt) eines 500 cm langen und mittels der C-14-Methode datierten Torfkerns aus dem ombrotrophen Pürgschachener Moor im steirischen Ennstal (Österreichische Alpen) vorgelegt und diskutiert. Der Bohrkern umfasst einen Zeitraum von ∼5000 Jahren. Frühe Hinweise auf Besiedelung und landwirtschaftliche Nutzung datieren auf ∼2900 cal BCE. Erste markante Cu-Anreicherungen, die auf Cu-Bergbau in den angrenzenden Eisenerzer Alpen zurückgeführt werden, zeigen sich in Torfschichten aus der späten Kupfersteinzeit (∼2500 cal BCE). Deutlichere Zunahmen von Kulturindikatoren (Kulturpflanzen, Sträucher, Kräuter, Holzkohle) im Pollendiagram und Anreicherungen von Spurenmetallen lassen auf signifikanten menschlichen Einfluss in der Umgebung des Pürgschachener Moores in der Mittelbronzezeit (∼1450–1250 cal BCE), in der Spätbronzezeit und (∼1050–800 cal BCE) sowie in der Periode der späten Latènezeit (∼300 cal BCE–1 cal CE) schließen. Der größte Teil der Eisenzeit sowie die römische Kaiserzeit sind durch einen allgemeinen Rückgang von anthropogenen Indikatoren im Vergleich zu früheren Perioden gekennzeichnet. Anreicherungen von Pb und Sb in einer Torfprobe aus dem Mittelalter (∼900 cal CE) können mit Sideritabbau in unmittelbarer Nähe des Pürgschachener Moores in Verbindung gebracht werden. Die Ergebnisse dieser interdisziplinären Studie belegen starke klimagesteuerte Wechselbeziehungen zwischen Pollen, dem Humifizierungsgrad und dem Aschegehalt. Menschliche Aktivität in der Umgebung des Moores zeigt eine geringe Korrelation mit Humifizierung und Aschegehalt, findet allerdings stärkeren Niederschlag in den Pollen- und Schwermetalldaten. Die vorliegende Studie weist auf eine ausgedehnte Trockenperiode in der Umgebung des Pürgschachener Moors um ∼1950 cal BCE hin.
- Article
(14240 KB) - Full-text XML
-
Supplement
(1025 KB) - BibTeX
- EndNote
1.1 Peat bogs as geochemical and palaeoenvironmental archives
In recent years, several multi-proxy studies of bogs, involving elemental, isotopic, and palynological and/or plant macrofossil analyses, have gained in significance, enabling relatively accurate interpretations and quantifications of human impact in the past (e.g. Bindler, 2003; De Vleeschouwer et al., 2007; van der Knaap et al., 2011; Segnana et al., 2019; von Scheffer et al., 2019). Ombrotrophic (rain-fed) peat bogs are solely dependent on precipitation and isolated from the direct influence of rivers, springs or groundwater. Consequently, such bogs provide excellent archives for reconstructing past atmospheric fluxes and environmental conditions (Martínez-Cortizas et al., 1999; De Vleeschouwer, 2010; Drexler et al., 2016). As the world faces future global climate change, a profound understanding of the interrelations of palaeoenvironmental and past human signals is of crucial importance for obtaining a more comprehensive picture of changes in the biosphere throughout the past. With respect to the Alpine region during the Holocene, palaeoenvironmental studies have mainly concentrated on the reconstruction of environmental conditions and past human impact in the Western Alps and western parts of the Eastern Alps (e.g. Büntgen et al., 2005; Nicolussi et al., 2005; Festi et al., 2014; Segnana et al., 2019). Only few data exist regarding areas in the eastern foothills of the Alpine main ridge in eastern Austria (e.g. Drescher-Schneider, 2003; Schmidt et al., 2006; Boch et al., 2009; Huber et al., 2010).
This present study seeks to close this research gap by combining (bio)geochemical, isotopic and palynological analyses of peat deposits of Pürgschachen Moor in the Austrian Alps. In particular, we provide further insights into palaeoenvironmental changes and the advent and chronology of prehistoric societies (from the Copper Age up to Roman times) and prehistoric copper mining in the Liezen area and the more eastern Eisenerz Alps. In addition, by also consulting the ash content and the humification degree, we aim to enhance the general knowledge regarding the interrelations between humification, mineralization, climate change and human impact in wetlands.
Pollen analysis is considered a reliable tool for inferring palaeoenvironmental changes and associated vegetational shifts (e.g. Finsinger et al., 2006; Herzschuh, 2007). Anthropogenic indicators in pollen records, such as crop plants and cereals, were first formalized by Behre (1981, 1988) and are nowadays commonly used to reconstruct agricultural and other human activities in the past (e.g. Bunting et al., 2001; Zhang et al., 2010; Rösch and Lechterbeck, 2016). The accuracy of assessments of past human activity by using geochemical methods depends, among other factors, on the mobility of relevant trace elements (e.g. Pb, Sb, Cu) in the peat environment.
Even though emissions of copper smelting processes are detectable in regular soils across tens of kilometres (Ettler, 2016) and the potential of Cu as an anthropogenic tracer in peat deposits has been highlighted by different authors (e.g. Ukonmaanaho et al., 2002; Rausch et al., 2005; Mighall et al., 2009; Novak et al., 2011; Mariet et al., 2016), other studies have questioned the reliability of Cu as a marker of past human impact in peat environments, due to its dependence on pH value, mineralogy (Rausch et al., 2005), vegetation (Shotyk et al., 2002; Ukonmaanaho et al., 2004) and hydrology (Tipping et al., 2003; Bobrov et al., 2011).
Therefore, we also measured Pb and Sb, which have successfully been used to reconstruct anthropogenic activity in various studies as they show low mobility in peat environments (e.g. Cloy et al., 2009; De Vleeschouwer et al., 2009; Shotyk et al., 2017). However, post-depositional redistribution processes of Pb and Sb have also been demonstrated occasionally (Olid et al., 2010; Rothwell et al., 2010). Mobilization of heavy metals in peat, in general, has been attributed to long-term water table fluctuations (Tipping et al., 2003; Rothwell et al., 2010).
Stable Pb isotope measurements were conducted to improve the contextualization of the elemental results, attempting to differentiate between local, regional and non-regional anthropogenic and geogenic sources (e.g. Allan et al., 2018). Organic compounds, especially humic acids that are considered the main adsorption agents in peat, provide adsorption sites that enhance trace element accumulation (Yang et al., 2019). To improve the quality of the interpretation of past anthropogenic activity, heavy metal concentrations were contrasted with the humification degree, provided by Fourier-transformed infrared (FTIR) spectroscopy (see also Biester et al., 2012). FTIR spectroscopy can be used to identify changes in major peat chemical properties, such as changes in relative contributions of carbohydrates or aromatics (Niemeyer et al., 1992; Kalbitz et al., 1999). Due to preferential degradation of carbohydrates over aromatics, relative absorption indicative of these moieties can be used as an indicator of the degree of peat decomposition and/or humification (Artz et al., 2008; Broder et al., 2012; Biester et al., 2014).
Since the extent of decomposition of peat is mainly dependent on plant species (Drollinger et al., 2020), nutrient fluxes and hydrological conditions, a connection between climate and decomposition can be established (van der Linden and van Geel, 2006). An assessment of the humification degree can therefore also help to reconstruct climate changes in the past.
The Sr concentration in bogs, to a large extent controlled by groundwater inflow, is regularly used as an additional proxy for the trophic status of the bog (Shotyk et al., 2017). As humification is closely interlinked with mineralization (Tolonen, 1984), an interpretation of ash content variations contributes to the reconstruction of past climate.
1.2 Regional setting and historical context
Pürgschachen Moor is the largest intact valley peat bog in Austria with an areal extent of ∼62 ha (Drollinger et al., 2019). Because of the peatland`s proximity to prehistoric mining sites and a large vertical extension of the peat body, it was chosen as the study site. The peat bog is located in the Styrian Enns valley, a large fault line that separates the Lower Tauern to the south from the Palaeozoic Greywacke zone and the Northern Calcareous Alps in the north (Keil and Neubauer, 2009). The mean annual temperature in the adjacent town of Admont is ∼7.3 ∘C. Annual precipitation, referring to the time between 1990 and 2019, is ∼1250 mm (Drollinger et al., 2019). The Greywacke zone, comprising the most significant copper ore resources of the Eastern Alps, lies between the Northern Calcareous Alps and the Central Eastern Alps (Kucha and Raith, 2009). The Greywacke zone in the study area contains Ordovician- to Devonian-age metamorphosed sedimentary rocks (turbidites, greywackes and limestones) and Ordovician-age mafic volcanic rocks (Gasser et al., 2009; Lutz and Pernicka, 2013; see Fig. 1).
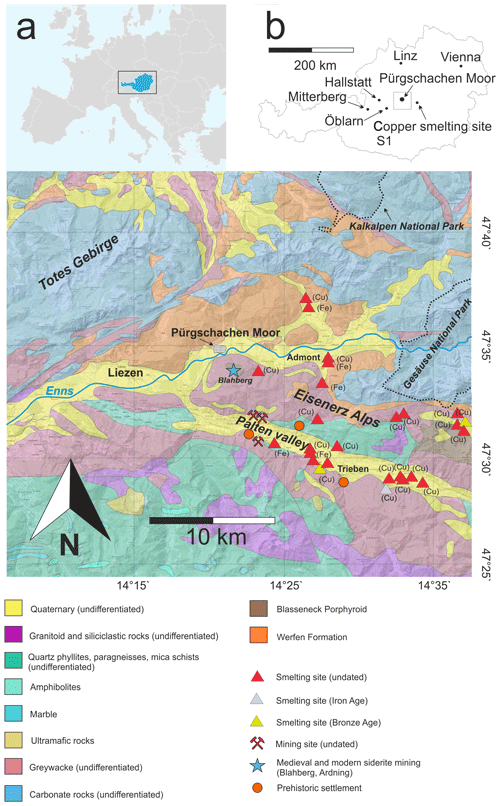
Figure 1Overview map with the geographical location of Pürgschachen Moor in the Enns valley and known prehistoric smelting sites and settlements (Klemm, 2003), embedded in the regional geology (Geologische Bundesanstalt, 2019). Europe (a), the prehistoric mining areas Mitterberg and Hallstatt, Öblarn, the copper smelting site S1, Linz and Vienna (b) are given as geographic references.
Copper ores of the eastern Greywacke zone are typically characterized by mineral phases of the tennantite–tetrahedrite solid-solution series (Cu12As4S13–Cu12Sb4S13) and chalcopyrite (CuFeS2)−pyrite (FeS2) mineralizations (Hanning, 2012; Haubner et al., 2015; Pernicka et al., 2016).
Palaeoenvironmental studies indicate a marked increase in metal pollution throughout Europe during the Bronze Age (e.g. Bränvall et al., 2001; Monna et al., 2004; Mighall et al., 2009; Longman et al., 2018; Wagreich and Draganits, 2018). This also applies to the Austrian Alps, where a gradual intensification of human activity is assumed for the middle Bronze Age and the early part of the late Bronze Age in different areas in Tyrol, Salzburg, Styria and Lower Austria (e.g. Röpke and Krause, 2013; Breitenlechner et al., 2014; Haubner et al., 2015; O'Brien, 2015; Pichler et al., 2018). Several studies have aimed to reconstruct the prehistoric human impact in the Mitterberg region in Salzburg, which was possibly the largest copper producer in Europe in the middle of the second millennium BCE (e.g. Stöllner, 2011; Breitenlechner et al., 2014; Pernicka et al., 2016). In contrast, relatively few detailed palaeoenvironmental studies exist regarding prehistoric settlement development and early mining in the Eisenerz Alps and the Gesäuse area. The earliest indications of large-scale settlement activity in the Palten valley, which forms the southernmost border of the Eisenerz region, date back to the late Neolithic period in the fourth millennium BCE (Drescher-Schneider, 2003; Preßlinger et al., 2009). Taking into account archaeological findings in the region, it is probable that a large number of non-specified prehistoric copper mining and smelting sites in the region can be attributed to the middle and/or late Bronze Age (Klemm, 2003; Preßlinger et al., 2009). The largest excavated bronze smelting site in the Eisenerz Alps, S1 in the Supplement (Fig. 1), has also been radiocarbon dated to the middle Bronze Age (1600–1350 cal BCE; Kraus, 2014).
Given the lack of archaeological evidence assignable to the Iron Age, a decrease in anthropogenic activity during the following centuries is suggested (Klemm, 2003). More intense human impact during the Roman period is indicated by different archaeological finds and evidence for Roman trade routes in the region (Herbert, 2015). A decline in archaeological findings corresponding to the Migration Period points towards a decrease in human activity during this interval (Klemm, 2003). Siderite mining (FeCO3) at Blahberg (Fig. 1) and in other parts of the area is documented, with interruptions, from the 11th century up to the year 1895 (Preßlinger and Köstler, 2002).
Even though no detailed palaeoclimate studies regarding the region of Eisenerz Alps have been conducted so far, we expect that the regional palaeoclimate variations show consistencies with palaeoclimate models of other parts of the Alps (e.g. Heiri, 2003; Nicolussi et al., 2005) and other climate records in the Northern Hemisphere (Vinther et al., 2006). Accordingly, we assume a general decrease in the average temperatures around 3000 cal BCE and an impact of recurring cold cyclical oscillations (Rotmoos 2, Löbben, Göschenen 1, Göschenen 2) in the late Holocene for this region (Ivy-Ochs et al., 2009; Kutschera et al., 2017).
2.1 Sample location
The sample location is in the centre of Pürgschachen Moor (47∘34 N, 14∘20 E), approximately 1.5 km southwest of Ardning (district of Liezen) at an altitude of 632 m (see Fig. 2).
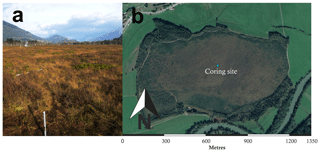
Figure 2Location of the coring site in the centre of the ombrotrophic Pürgschachen Moor (a; eastern view from the coring site; photo: W. Knierzinger), Ardning (Austria). Aerial view of Pürgschachen Moor (b) with the location of the coring site (modified from ©Google Maps, last access: 15 December 2019) and scale.
The peat body at the central treeless peatland area of Pürgschachen Moor reaches depths of 7–8 m; the transition from sedge to Sphagnum peat has been identified at a depth of 5 to 6 m (Birker, 1979). The bog is one of the last remains of a former string of peatlands along the Enns river valley (Drollinger et al., 2019). The vascular vegetation of the peat bog is comprised of mosaic patterns across the peat bog area, consisting of the most abundant shrub species Pinus mugo, Calluna vulgaris, and the graminoids Eriophorum vaginatum and Rhynchospora alba. The most abundant Sphagnum mosses are Sphagnum capillifolium, Sphagnum magellanicum and Sphagnum tenellum (Drollinger et al., 2019).
2.2 Coring and preparation
A ∼500 cm long peat core (diameter of 80 and 50 mm) was extracted by means of a Russian peat corer of 8 cm diameter in late 2017. At a depth of ∼500 cm, coring had to be aborted due to impenetrable layers associated with the more frequent presence of roots and other wooden material. The core was stored below 6 ∘C. Prior to analysis, the sample material was dried overnight and then pulverized. In total, 45 samples (∼200 mg) were used for elemental analysis, 34 samples (∼200 mg) for Pb isotopic analysis and 41 (∼40 mg) for FTIR analyses. For the analyses of ash contents 100 samples (∼1 g), taken at intervals of 5 cm, were used. The labelling of the samples denotes information on the sampling depth (i.e. P308 corresponds to 308 cm below surface). Due to a variety of analytical techniques, analyses were not stringently performed at regular measuring intervals.
2.3 Radiocarbon dating
Six samples, comprised of various Sphagnum plant fragments and one bark fragment of Pinus sylvestris, were radiocarbon dated at the Poznan Radiocarbon Laboratory in Poland. To remove unwanted components and to isolate the dateable fraction, the acid–alkali–acid procedure was applied (for further details see Czernik and Goslar, 2001). The top of the extracted peat core was assigned to the year 2012. A Bayesian radiocarbon model was established using Bacon (version 2.2; see Blaauw and Christen, 2011), which is based on the IntCal13 calibration curve (Reimer et al., 2013).
2.4 Pollen analysis
A total of 65 pollen samples at sub-sampling intervals varying between 2.5 and 10 cm were analysed. In order to obtain pollen concentration data, Lycopodium tablets were added to each sample (1 cm3 of material; see Stockmarr, 1971). Peat samples were chemically treated using acetolysis. Pollen counting was performed using a Leitz Biomed optical microscope with 400× and 1000× magnification with phase contrast. At least 900–1000 pollen grains per sample were counted and identified based on Beug (2004). The non-pollen palynomorphs (NPPs) were determined on the basis of numerous reference studies provided by Miola (2012). The results are presented as reduced-percentage diagrams, calculated and drawn using the software package Tilia 2.1.1 and TGView (Grimm, 2004). Pollen grains of trees, shrubs and upland herbs (arboreal pollen (AP) + non-arboreal pollen (NAP) = 100 %) are presented. Pteridophyta, mosses and water plants are not included in the pollen sum.
The pollen diagram is divided into local pollen zones (LPZ P1–P13) that reflect the vegetation development (Berglund and Ralska-Jasiewiczowa, 1986). Periods of human impact (K1–K7) are displayed separately.
2.5 Elemental analysis and ash contents
Quantitative analyses of Cu, Sr, Zr, Sb, and Pb were carried out by using an iCAP Qc ICP-MS system (Thermo Fisher Scientific, Bremen, Germany) at the Institute of Chemical Technologies and Analytics (TU Wien, Vienna). Different research groups demonstrated accurate elemental analysis of peat using quadrupole-based ICP-MS instruments (e.g. Shotyk et al., 2001; Krachler et al., 2002; Mihaljevič et al., 2006; Mighall et al., 2014). Peat aliquots of 200 mg were digested using an acid mixture of 4 ml HNO3 (65 %), 2 ml HCl (37 %) and 2 ml H2O2 (30 %) in a microwave-assisted closed-vessel system (Multiwave 3000, HF 100 vessels, Anton Paar, Graz, Austria).
Calibration standards from 1 to 100 µg mL−1 were prepared using the ICP multi-elemental standard solution VIII Certipur® (Merck Darmstadt, Germany), the ICP multi-elemental standard solution IV Certipur® (Merck Darmstadt, Germany) and a zirconium ICP standard Certipur® (Merck Darmstadt, Germany). All measurements were blank-corrected. Differences in sample introduction efficiency, due to variations in physical properties such as surface tension or viscosity, and also differences in sample atomization and excitation efficiency, due to variations in the plasma load, were minimal; concentrations after dilution of the sample digests were only in the range of micrograms per litre to milligrams per litre. 115In was used as an internal standard for correction of remaining effects and/or instrument drifts. The choice of the conservative element for normalization of metal concentrations generally depends on the given environmental, geographic and geological conditions (Boës et al., 2011; Kylander et al., 2016). In this study, Zr, indicative of geogenic siliciclastic input of adjacent relatively zircon-rich Austroalpine nappes (Stattegger, 1982) and Gosau Group sediments (Wagreich, 1988), was chosen as a conservative element for normalization purposes.
Due to the focus on the reconstruction of prehistoric anthropogenic activity, no elemental measurements were performed for the first 75 cm. Operation settings are given in the Supplement, Table S1. Due to the assumed absence of unambiguous non-anthropogenic reference sections in the core, no enrichment factors (see e.g. Weiss et al., 1999) were calculated. Ash contents were determined as the percentage of the dry weight (see Tolonen, 1984), with a precision balance after burning the samples at 550 ∘ C for 10 h in a muffle furnace.
2.6 Pb isotope analysis
Stable Pb isotope measurements were carried out by using the iCAP Qc ICP-MS system described in Sect. 2.5. The isobaric interference between 204Hg and 204Pb was corrected by monitoring the signal of 200Hg. The Pb ICP standard Certipur® (Merck, Darmstadt, Germany), traceable to NIST standard reference material SRM® 928 Pb, was used as a bracketing standard for performance verification and correction of instrumental drift. The Pb ICP standard Certipur® was characterized with the aid of the certified reference material ERM-EB 400 (Federal Institute for Materials Research and Testing, BAM). The previously prepared 50 mL liquid samples were diluted (up to 1:50) to guarantee a high degree of comparability. The raw Pb isotope ratios obtained from the measured samples were corrected for contributions of Hg, spikes and blanks. In a subsequent step, the isotope ratios were corrected for isotopic fractionation based on bracketing standard measurements. Due to complete acid digestion and high-dilution factors, matrix effects were considered to be negligible, and hence no additional reference material with a biological matrix was analysed. Notwithstanding the fact that thermal ionization (TIMS) and multi-collector plasma mass spectrometry (MC-ICP-MS) provide higher analytical precision (e.g. Gulson et al., 2018), the Q-ICP-MS technique was deemed sufficient for establishing a temporal trend and for a general contextualization of the peat samples with regard to other European signals (Mihaljevič et al., 2006; Kylander et al., 2005; Judd and Swami, 2010; Mighall et al., 2014).
2.7 Fourier-transform infrared spectroscopy
FTIR spectra of peat samples were recorded by means of a Cary 660 FTIR spectrometer (Agilent, Santa Clara, CA, USA) at the Institute of Landscape Ecology in Münster, Germany. On the one hand, the selection of samples for FTIR analyses was based on prominent trends in the ash content curve; on the other hand it was oriented at the sample selection for ICP-MS analysis. For FTIR analyses, 2 mg of powdered peat sample was mixed with 200 mg KBr (FTIR grade, Sigma Aldrich, St. Louis, MO, USA) and pressed to a 13 mm pellet. To reduce analytical noise and to obtain comparable spectra for all samples, 32 scans per sample and subsequent baseline correction of data were conducted. The humification index was approximated by calculating the ratio between the peak intensity at 1630 cm−1 (indicative of lignin and other aromatics) and at 1030 cm−1 (indicative of polysaccharides such as cellulose; see also Holmgren and Norden, 1988; Biester et al., 2014). Exact localization of wavenumbers of specific peaks in FTIR data was accomplished by using an automated R (version 3.5.1; R Core Team, 2018) custom script that enables a baseline correction of peaks and a conversion into relative abundances (Hodgkins et al., 2018).
3.1 Peat chronology and pollen analysis
Generally, the retrieved core is dark brown to black; contains a lot of amorphous material; and appears to be moderately to highly decomposed, according to the von Post humification scale (von Post, 1924). The section ∼375–∼385 cm comprises very highly decomposed homogeneous peat. Macroscopic visual inspection revealed increased woody plant material at the base of the core (∼480–∼500 cm). Calibrated ages are given in Table 1. The Bayesian chronological model is displayed in Fig. 3 (see also the Supplement).
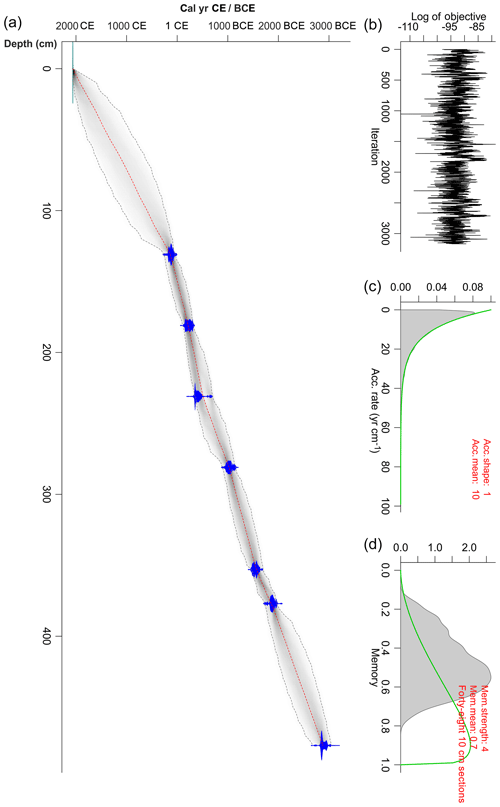
Figure 3(a) Chronological model of the Pürgschachen core based on Bayesian statistics combining the radiocarbon dates and the surface date of the core (R package Bacon; see Blaauw and Christen, 2011). The top of the extracted peat core was assigned to the year 2017. Calculated dates are represented in blue; the grey area displays the 95 % confidence interval. The number of Markov chain Monte Carlo iterations (b) used to generate the age–depth model; the prior (green) and posterior (grey) distributions of accumulation rates (c) and memory (d).
The pollen zone (PZ) P1 (484–450 cm; ∼3000 BCE–2380 cal BCE), displays a relatively heterogeneous development. In PZ P1a (484–467.5 cm), the lowest sample (484 cm) is attributed to a wood layer (most likely pine), which formed the drilling-resistant base of the core. Compared to adjacent peat sections, a rarity of Amphitrema and Neorhabdocoela is apparent. At about 470 cm, a slight increase in Cannabaceae was found. PZ P1b is characterized by dense spruce forest, mixed with beeches and firs (see Figs. 4 and 5).
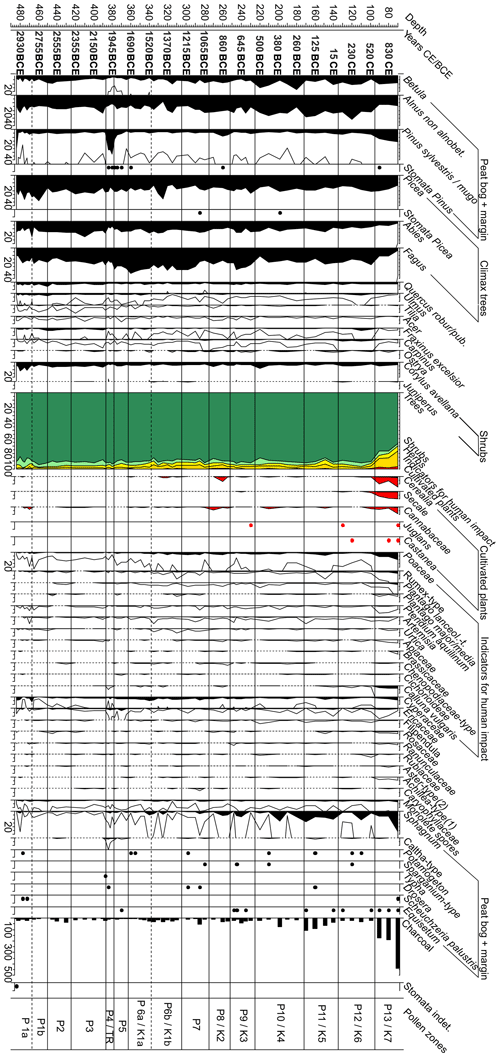
Figure 4Reduced pollen diagram of the peat core, comprising trees, shrubs, cultivated plants, other indicators of human impact and typical wetland flora. For reasons of clarity, 10× exaggeration curves (thin black lines) were integrated to highlight the abundance of infrequent components.
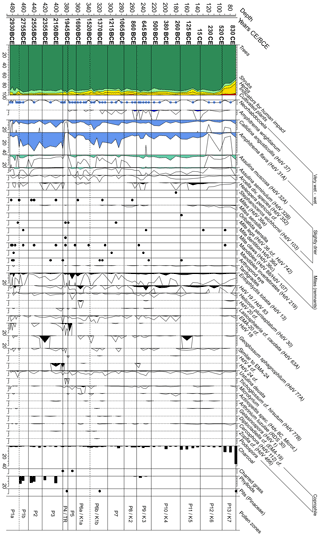
Figure 5Diagram of non-pollen palynomorphs (NPPs), showing changes in wetness and animal content. NPP types with a constant presence of less than 0.5 % are shown as dotted lines. For reasons of clarity, 10× exaggeration curves (thin black lines) were integrated to highlight the abundance of infrequent components.
In PZ P2 (450–425 cm), a fir- and beech-rich spruce forest still predominates in the vicinity of the bog. In the pollen record, a slight decrease in Fagus and increases in charcoal and Corylus avellana are apparent. Besides, high values of Amphitrema flavum were determined.
Apart from an expansion of Fagus, PZ P3 (425–387 cm) is characterized by a relatively invariable vegetation development, showing more or less consistent values of Alnus, Picea and Abies.
In PZ P4 (387–378 cm; ±2025–1913 cal BCE) a massive increase in Pinus and slightly higher values of Calluna, Ericaceae and Caltha were noted. In contrast, the values of other forest trees, Amphitrema and Callidina decrease during this period.
A continuous increase in Fagus characterizes the vegetation of PZ P5 (378–362.5 cm). PZ P6/K1 (362.5–305 cm) covers a more prolonged period (∼1720–∼1255 cal BCE), ranging from the end of the early Bronze Age to the middle Bronze Age. In the lower section (PZ P6a/K1a, 362.5–337.5 cm; ∼1720–∼1500 cal BCE) a decrease in beech and fir as well as an increase in birch, hazel and cultural indicators like Cerealia, Cannabaceae, Plantago lanceolata, Rumex type, Artemisia, Chenopodiaceae and Pteridium aquilinum was determined.
PZ P6b/K1b (1500–1255 cal BCE) is characterized by frequent finds of Cerealia and Cannabaceae and an increase in Urtica, Plantago lanceolata, Juniperus and grasses, accompanied by a strong decline in elms, lime trees, maples and oaks and an expansion in birch. Especially in the younger part of PZ P6b, a decline in fir and spruce is apparent. A significantly higher input of charcoal was noted.
PZ P7 (305–275 cm) covers the early and a part of the late Urnfield period (1250–1010 cal BCE). The pollen record of PZ P7 show lower levels of Cannabaceae and Rumex as well as a decrease in charcoal. Around 290 cm, there is an apparent decrease in beech and an increase in alder and hazel. Higher charcoal input was detected at a depth of 285 cm. PZ P8/K2 (275–252 cm) is associated with the late Urnfield period (1020–780 cal BCE). In this section, a decline in firs, spruces and – to a lesser extent – beeches was found; hazel was becoming more common. A more frequent occurrence of Cerealia was found. Elevated charcoal levels were detected in younger sections of this zone.
PZ P9/K3 (252–225 cm) is characterized by a decrease in Cannabaceae and Poaceae. Decreases in charcoal, spruce and beech and a marked increase in hazel are associated with PZ P10/K4 (225–172 cm). Amphitrema flavum shows a marked decrease at ∼235 cm.
The pollen record of PZ P11/K5 (172–135 cm) still implies a dense forest stand and displays pronounced increases in Poaceae, Rumex and Artemisia. The charcoal input between 172 and ∼150 cm is higher than ever before.
The first occurrences of rye, walnut and sweet chestnut stretch back to PZ P12/K6 (135–95 cm), accompanied by a slight decrease in beech, fir and spruce. Charcoal is barely detectable.
In PZ P13/K7 (95–70 cm) the number of most forest trees, with the exception of pines and birches, is strongly reduced. In addition to a more frequent occurrence of rye and other cereals, an increase in charcoal and walnut was found. Furthermore, the presence of heather and an increase in pine as well as a decrease in wetness indicators were determined.
3.2 Elemental and biochemical analyses
Sr concentrations are rather invariable throughout the core, ranging from 3 to 10 mg kg−1. A slight increase in Sr was noted in the basal section (450–482 cm) of the core (see Fig. 6). A relatively high degree of invariability also applies to Zr (∼0.1 to 2 mg kg−1). Only the middle section of the core (233–283 cm) is characterized by comparatively higher Zr concentrations, peaking at 236 cm with ∼19 mg kg−1. Cu∕Zr ratios show enrichments at 153 cm, at 155 cm, in the section 305–310 cm, at 335, at 415 and at 430 cm. Pb∕Zr and Sb∕Zr ratios largely run parallel to each other, showing peaks at 75, 118, 145, 175, 277, 303, 305 and 310 cm. Apart from that, Pb∕Zr ratios are elevated at 415, 450, 475 and 482 cm. A rather strong positive Pearson's correlation coefficient (r) of 0.49 between Sb and Pb was found. Sb and Cu are also weakly positively correlated (r=0.27). In comparison to the average ash content (1.07 %) of the homogeneous lower sections of the core (50–350 cm), the average ash content of the uppermost section (1–50 cm) is clearly elevated (2.19 %). Marked increases were determined for 380 cm (5.1 %) and the lowermost section ranging from 490 to 500 cm (up to 9.8 %). The FTIR humification proxy (ratio 1630 cm−1 / 1030 cm −1) displays increased values at 75, 150, 165, 175, 230, 235, 250 and 380 cm. Particularly low humification degrees were determined at 160, 272, 400, 450 and 460 cm and in the section 330–350 cm. The humification proxy shows a positive correlation with Pb (r=0.55) and Sb (r=0.76). While Cu () and Zr (r=0.29) display no or no strong particular relationships with humification, Sr () is negatively correlated with the FTIR humification proxy (see the Supplement). The colour of the peat ash varies from brownish white (e.g. middle section of the core) to greyish white (core section 375–385 cm).
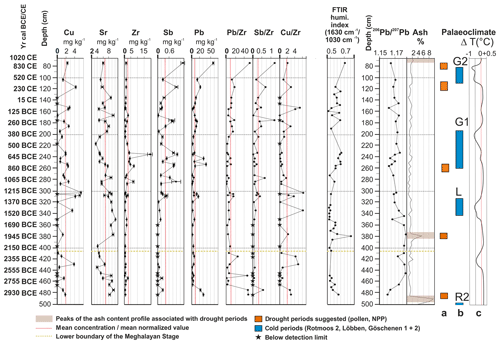
Figure 6Element concentrations, as obtained from ICP-MS analyses, of Cu, Sr, Zr, Sb and Pb (mg kg−1); Zr-normalized Pb, Sb and Cu values; FTIR humification index (1630 cm−1 / 1030 cm−1); Pb206 / Pb207; and ash content vs. depth. Marked changes in wetness proxies (based on values of Pinus, Callidina and Amphitrema) in the pollen and NPP records (a; see also Figs. 4 and 5), indicating drought periods, are largely consistent with peaks in the ash content profile. Climate oscillations (b) Rotmoos 2 (R2), Löbben (L), Göschenen 1 (G1) and Göschenen 2 (G2) (Ivy-Ochs et al., 2009) and the relative summer palaeotemperature variation in the European Alps (c; see Kutschera et al., 2017) are given as references. Error bars (3-sigma) were calculated on three replicates. Concentrations below the detection limit (black stars) are predominantly concentrated below 350 cm. The dashed yellow line corresponds to the formally defined base of the late Holocene, the Meghalayan Stage (Walker et al., 2019). The red line (see c; 0 ∘C) represents the mean temperature between 1990 and 2000 CE in the Alps.
3.3 Pb isotopes
206Pb∕207Pb ratios of peat from Pürgschachen Moor (75–482 cm) range between 1.1602 and 1.1829, showing a decreasing trend towards the uppermost section. The two lowermost samples (P482, P475) deviate significantly from this trend. A similar development is evident regarding 206Pb∕208Pb ratios. No particular temporal evolution was found for the 207Pb∕208Pb ratios (see the Supplement).
4.1 Climate-driven interrelations of peat proxies
The results of our study demonstrate climate-driven interrelations between the pollen record, the ash content and peat decomposition. Cultural activity, in contrast, is mainly reflected in the pollen record and by enrichments of trace elements (see Sect. 4.2). Varying ash contents in peat bogs have been linked to climate changes in two ways: on the one hand, the ash content is affected by a varying dust flux due to climate variations and human land-use change, which directly influence the amount of mineral matter in the bog (Pratte et al., 2017). On the other hand, the amount of mineral matter in peat is strongly interrelated with humification processes (Tolonen, 1984). Due to mass loss of organic matter in peat bogs in the course of proceeding decomposition during drier periods (see also Huang et al., 2013; Xiao et al., 2017), an enrichment of mineral matter per volume unit takes place, resulting in an increase in the ash content (Boelter, 1969). This, in turn, might improve nutrient availability and could lead to a positive feedback on microbial decomposition of peat (Kempter, 1996).
On Pürgschachen Moor, the strongest positive correlation between humification and ash content was generally found in sections with higher ash contents. Positive correlations between decomposition rates and the ash content in peat are emphasized by different authors (e.g. Tolonen, 1984; Kempter, 1996; Martínez Cortizas et al., 2007; Engel et al., 2010; Leifeld et al., 2011; Drzymulska, 2016). However, a direct linkage between higher ash contents and warmer climate conditions causing slower growth rates and higher mineralization rates (see also Sîre et al., 2008; Huang et al., 2013; Xiao et al., 2017) does not always hold up to scrutiny, as Stivrins et al. (2017) imply by underlining the capacity of peat bogs to buffer climate variations. This is further aggravated by the fact that, from a climatological perspective, no stable relationship between temperature and precipitation exists (Bell et al., 2018).
Despite that, with regard to the present study, the erratic increase in the ash content (up to ∼5 %) and the humification index (see Fig. 6) at a core depth of ∼380 cm can be linked to increased decomposition and mineralization rates of organic material during a late-Atlantic climate optimum in the time between ∼2300 and ∼1700 cal BCE (Kutschera et al., 2017), which most likely initiated a temporal drying phase of the bog around ∼1950 cal BCE. This interpretation is further corroborated by a decrease in Amphitrema and Callidina, a massive increase in Pinus, and slightly higher values of Calluna, Ericaceae and Caltha in this section.
A pronounced Zr peak and a decrease in Amphitrema flavum at 236 cm, corresponding to ∼600 cal BCE, might be related to dry climate conditions during the Göschener 1 oscillation. Distinct colour differences in ash samples between sample material with relatively higher ash contents (brighter in section 385–375 cm) and sample material with lower ash contents (e.g. darker in section 180–240 cm) also indicate a linkage between ash colour on the one hand and the humification degree and ash content on the other. In the literature, brighter colours of ash are attributed to varying plant species (Babayemi et al., 2010), higher fire severity (Hogue and Inglett, 2012) or higher ash content (enhanced mineral matter; Bodí et al., 2014). As the vegetation of the peat is relatively stable throughout the core and given the fact that the same burning conditions have been applied to all peat samples, the brighter ash colours in section 385–375 cm are likely to be attributed to the higher mineral content in this section.
Similar interrelations (high ash content, marked changes in the wetness indicators, increasing humification) are apparent in the section 80–70 cm that corresponds to the initial phase of the Medieval Warm Period (Kutschera et al., 2017) and in the section 500–490 cm, corresponding to the warm period around 3000 cal BCE, following the Rotmoos II oscillation (Ivy-Ochs et al., 2009). Strongly increased ash contents in this lowermost section are, however, at least partly linked to the more frequent presence of roots and tree trunks that precluded deeper drilling.
In general, low average Sr concentrations below 10.5 ppm and low ash contents (∼Ø1.5 %) point towards an ombrotrophic nature of the upper 500 cm of Pürgschachen Moor. The marked increase in ash contents in subsurface layers of Pürgschachen Moor is in good accordance with conditions in comparable European peat bogs (Smieja-Król and Fiałkiewicz-Kozieł, 2014).
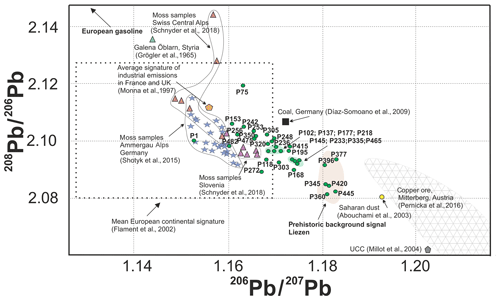
Figure 7Pb isotopic signatures (208Pb∕206Pb vs. 206Pb∕207Pb) from peat samples of Pürgschachen Moor (P sample numbers, green dots) in comparison with copper ores at Mitterberg (Salzburg, Austria; Pernicka et al., 2016), galena at Öblarn (Styria, Austria; Grögler et al., 1965), moss samples from the Ammergau Alps (Bavaria, Germany; Shotyk et al., 2015), and moss samples taken in Slovenia and in the Swiss Central Alps (Schnyder et al., 2018). Average Pb isotopic signatures of industrial emissions from France (Monna et al., 1997) and of the upper continental crust (UCC; Millot et al., 2004) as well as the Pb isotopic composition of German coal (Díaz-Somoano et al., 2009) and Saharan dust (Abouchami et al., 2003) are given as further references. The average European gasoline Pb isotopic composition is characterized by low 206Pb∕207Pb and high 208Pb∕206Pb ratios (e.g. Monna et al., 1997), indicated by an arrow (top left). The low 206Pb∕207Pb ratio of P1 is attributed to increased industrialization processes and the peak of leaded gasoline consumption in recent decades (see also Cloy et al., 2008; Forel et al., 2010; Shotyk et al., 2015; Drexler et al., 2016; Schnyder et al., 2018). Radiocarbon dates from Pürgschachen Moor (see Table 1).
4.2 Late-Holocene human activities in the Liezen area
Strong positive correlations between Pb and Sb in peat soils affected by anthropogenic activity such as mining, smelting and industrialization have been highlighted by different authors (e.g. Steinnes, 1997; Shotyk et al., 2004; Cloy et al., 2005; Galuszka et al., 2014). Yet, anthropogenic activities are not necessarily the only reason for higher concentrations of Pb and Sb in ombrotrophic peat, as a positive relationship between Pb and Sb on the one hand and the humification degree on the other is demonstrated in various studies (e.g. Pilarski et al., 1995; Ho et al., 2000; Gao and Huang, 2009; Biester et al., 2012). However, with regard to this study, humification-normalized Pb and Sb values do not change the interpretation of past anthropogenic activity in the area of Pürgschachen Moor.
Though positive correlations between Cu and humification are known from the literature (e.g. Yang and Hodson, 2018), no such correlation was determined for peat of Pürgschachen Moor. This correlation might have been overwritten by occasional input of anthropogenic copper smelting dust. The weak positive correlation between Sb and Cu might be linked to the smelting of antimony-rich copper ores.
A comparative analysis of anthropogenic proxies in the pollen record (i.e. cultivated plants, herbs shrubs, charcoal) and in the geochemical record (Pb, Sb, Cu) reveals interrelations. However, since no continuous concordance between the palynological and geochemical anthropogenic proxies were found over time or depth, it is reasonable to assume that prehistoric regional settlement activity in the Liezen area was only partly connected to mining.
Taking into account the low average Pb concentrations (∼7 mg kg−1) of the prehistoric samples of the peat core, high Pb concentrations of up to ∼30 mg kg−1 in the lowermost Copper Age section (∼3000 to 2800 cal BCE) of the core are very untypical and are not considered to be anthropogenic in origin. These peaks in the Pb profile are not correlated with Cu and are most probably caused by higher influx of galena (PbS). Galena is a relatively frequent accessory mineral in different lithologies in the Liezen area (Schumann, 2002). A higher geogenic influx of galena – possibly connected to a slightly increased groundwater influence as indicated by slightly higher Sr concentrations in the lowermost section – might also be the reason for untypically low 206Pb∕207Pb ratios at 475 and 482 cm (see the isotopic signature of galena from Öblarn in Fig. 7; see also Wagreich and Draganits, 2018). Moreover, galena tends to incorporate, among other elements, higher amounts of Sb (e.g. Laflamme and Cabri, 1986; Sharp and Buseck, 1993), and this might explain a high Sb∕Zr ratio at 475 cm. Another explanation for these lower 206Pb∕207Pb ratios may be diffuse contamination of the peat samples during core retrieval or sample preparation.
A weak forest opening combined with higher levels of cultivated plants at 470 cm, corresponding to ∼2800 cal BCE, might reflect early agricultural activity in the surroundings of the peat bog.
A slight decrease in Fagus with a concomitant increase in charcoal and Corylus avellana may indicate a weak anthropogenic disturbance in the following PZ P2. A Cu enrichment at 430 cm, corresponding to PZ P2, around 2500 cal BCE, is interpreted as the incipient indication of larger copper mining activity in the region. Despite the fact that the pollen analyses do not imply human activity in the immediate proximity of Pürgschachen Moor at that time, Neolithic settlement activity is known from the Liezen area and the Eisenerz Alps (Drescher-Schneider, 2003). The absence of cultural indicators and low trace element enrichments in the section PZ P3–PZ P5 (425–362.5 cm) suggest a decrease in settlement and mining activity in the following centuries. This time (∼2400–∼1700 cal BCE) roughly corresponds to a late-Atlantic warm period characterized by Alpine glacial recession (Kutschera et al., 2017).
Increasing human impact in the region in the middle Bronze Age (∼1450–∼1250 cal BCE) is implied by cultural indicators in the pollen record and elevated trace metal concentrations. Intense human activity in the immediate vicinity of Pürgschachen Moor seems to diminish again during the period of the early Urnfield culture (∼1250–∼1050 cal BCE). Nevertheless, enrichments of Cu and Sb indicate ongoing mining activity in the region during this time. While intense local agricultural and human activity is reflected in peat sections (more frequent occurrence of cereals and Cannabaceae) associated with the period of the late Urnfield culture (∼1050–∼800 cal BCE), the geochemical data do not imply significant metallurgical activity in the region. The following centuries of the early and middle Iron Age (∼750–∼300 cal BCE) are characterized by decreasing trace metal enrichments and slightly decreasing cultural indicators in the pollen record. This finding is consistent with archaeological evidence from the region (Klemm, 2003) and might be connected to a cooling period in the second half of the first millennium BCE (Nicolussi et al., 2005). Significantly higher charcoal influx and enrichments of Sb and Cu in the period of the late La Tène culture (∼230–∼50 cal BCE) point towards a phase of reinvigorated settlement and mining activity. Unlike areas in the Eisenerz Alps (Klemm, 2003; Drescher-Schneider, 2003), no indications of intense human activity during Roman times were found with regard to the local environment of Pürgschachen Moor.
Distinct enrichments of Pb and Sb in one sample, corresponding to ∼900 cal CE, are attributed to medieval siderite mining activity in the immediate vicinity of Pürgschachen Moor (Preßlinger and Köstler, 2002).
By combining palynological and geochemical analyses, we were able to provide a substantial reconstruction of prehistoric human and metallurgical activity as well as the palaeoclimate in the region of Pürgschachen Moor that is largely coherent with previous palaeoenvironmental and archaeological studies. After first indications of low settlement (∼2900 cal BCE) and mining activity (∼2500 cal BCE) in the region in the late Copper Age, a reduction in human presence in the early Bronze Age is suggested by a lack of evidence in the geochemical and palynological records. The period from ∼1450 to ∼1250 cal BCE and another period from ∼1050 to ∼800 cal BCE, both related to a phase of climate warming (∼1400–∼800 cal BCE) after the Löbben oscillation in the middle and late Bronze Age, are characterized by slightly increased geochemical and palynological signals of human presence. We propose that these signals reflect elevated mining activity in the Eisenerz Alps and in the more southern Palten valley. Following a clear decrease in anthropogenic signals in the early and middle Iron Age (∼750–∼300 cal BCE), a phase of more intense human activity occurs in the period from ∼300 to ∼1 cal CE. Relatively insignificant evidence of human presence and metallurgical activity during the Roman period was found.
The palaeoenvironmental assessment of the pollen data was further substantiated and refined by interrelating it with the humification degree and ash content. Owing to this fact, we were able to correlate climate optima in the late Holocene with changes in the geochemical and pollen and NPP records. Even though considered negligible for the interpretation of this present study, the close interrelationship between humification and tracer elements such as Pb and Sb demonstrates the necessity for a stronger analytical consideration of the humification index in the context of interpreting anthropogenic pollutants from peat bog archives. In order to improve the explanatory power of palaeoenvironmental assessments, future studies may try to further disentangle humification-related trace element sorption from mineralization processes in peat soils.
All underlying chemical data have been submitted to PANGAEA https://doi.org/10.1594/PANGAEA.919320 (Knierzinger, 2020).
The supplement related to this article is available online at: https://doi.org/10.5194/egqsj-69-121-2020-supplement.
WK (corresponding author) designed the study, prepared sample material for subsequent analysis, conducted geochemical and laboratory analyses, and wrote the manuscript. The peat core was retrieved by WK and RDS. Pollen analysis was performed by RDS. KHK conducted the FTIR analyses; SD provided FTIR data normalization. AL, LB and FH were involved in planning and supervision of the geochemical analyses. MW contributed to the design and the structure of the manuscript. DF established the Bayesian age–depth model.
The authors declare that they have no conflict of interest.
This present work was funded by the uni:docs fellowship programme for doctoral candidates (University of Vienna). We would like to thank Mathias Steinbichler, Susanne Klemm, Stephan Glatzel, Andreas Maier, Tomasz Goslar, Susanne Gier, Sabine Hruby-Nichtenberger and Lars Scharfenberg for technical and logistical support. We are also grateful to Benjamin Schmid for proofreading the manuscript.
This research has been supported by the University of Vienna uni:docs fellowship programme for doctoral candidates (grant no. 6395).
The article processing charges were partly funded by
the Quaternary scientific community, as represented by
the host institution of EGQSJ, the German Quaternary
Association (DEUQUA).
This paper was edited by Christopher Lüthgens and reviewed by two anonymous referees.
Abouchami, W. and Zabel, M.: Climate forcing of the Pb isotope record of terrigenous input into the Equatorial Atlantic, Earth Planet. Sci. Lett., 21, 221–234, 2003.
Allan, M., Pinti, D. L., Ghaleb, B., Verheyden, S., Mattielli, N., and Fagel, N.: Reconstruction of Atmospheric Lead Pollution During the Roman Period Recorded in Belgian Ombrotrophic Peatlands Cores, Atmosphere, 9, 1–18, https://doi.org/10.3390/atmos9070253, 2018.
Artz, R. R. E., Chapman, S. J., Robertson, A. H. J., Potts, J. M., Laggoun-Défarge, F., Gogo, S., Comont, L., Disnar, J. R., and Francez, A. J.: FTIR spectroscopy can be used as a screening tool for organic matter quality in regenerating cutover peatlands, Soil Biol. Biochem., 40, 515–527, 2008.
Babayemi, J. O., Dauda, K. T., Nwude, D. O., and Kayode, A. A. A.: Evaluation of the Composition and Chemistry of Ash and Potash from Various Plant Materials-A Review, J. App. Sci., 10, 1820–1824, 2010.
Behre, K. E.: The interpretation of anthropogenic indicators in pollen diagrams, Pollen Spores, 23, 225–245, 1981.
Behre, K. E.: The role of man in European vegetation history, in: Vegetation history, edited by: Huntley, B. W. and Webb III, T., Kluwer, Dordrecht, 633–672, Kluwer, Dordrecht, 1988.
Bell, M. C., Riton, J. P., Verhoef, A., Brazier, R. E., Templeton, M. R., Graham, N. J. D., Freeman, C., and Clark, J. M.: Sensitivty of peatland litter decomposition to changes in temperature and Rainfall, Geoderma, 331, 29–37, 2018.
Berglund, B. E. and Ralska-Jasiewiczowa, M.: Pollen analysis and pollen diagrams, in: Handbook of the Holocene Palaeoecology and Palaeohydrology, edited by: Berglund, B. E. and Ralska-Jasiewiczowa, M., John Wiley & Sons, Chichester, 455–484, 1986.
Beug, H. J.: Leitfaden der Pollenbestimmung für Mitteleuropa and angrenzende Gebiete, Pfeil, Munich, 2004.
Biester, H., Hermanns, Y. M., and Cortizas, A. M.: The influence of organic matter decay on the distribution of major and trace elements in the ombrotrophic mires – a case study from the Harz Mountains, Geochim. Cosmochim. Acta, 84, 126–136, 2012.
Biester, H., Knorr, K.-H., Schellekens, J., Basler, A., and Hermanns, Y.-M.: Comparison of different methods to determine the degree of peat decomposition in peat bogs, Biogeosciences, 11, 2691–2707, https://doi.org/10.5194/bg-11-2691-2014, 2014
Bindler, R.: Estimating the natural background atmospheric deposition rate of mercury utilizing ombrotrophic bogs in southern Sweden, Environ. Sci. Technol., 37, 40–46, 2003.
Birker, R.: Zur Ökologie und Torfstratigraphie des Pürgschachen Moores, PhD. thesis, University of Graz, Graz, 251 pp., 1979.
Blaauw, M. and Christen, A. J.: Flexible Paleoclimate Age-Depth Models Using an Autoregressive Gamma Process, Bayesian Anal., 6, 457–474, 2011.
Bobrov, V. A., Bogush, A. A., Lenova, G. A., Krasnobaev, V. A., and Anoshin, G. N.: Anomalous Concentrations of Zinc and Copper in Highmoor Peat Bog, Southeast Coast of Lake Baikal, Doklady Earth Sci., 439, 784–788, 2011.
Boch, R., Spötl, C., and Kramers, J.: High-resolution isotope records of early Holocene rapid climate change from two coeval stalagmites of Katerloch Cave, Austria Quaternary Sci. Rev., 28, 2527–2538, 2009.
Bodí, M. B., Martin, D. A., Balfour, V. N., Santín, C., Doerr, S. H., Pereira, P., Cerdà, A., and Mataix-Solera, J.: Wildland fire ash: Production, composition and eco-hydro-geomorphic effects, Earth Sci. Rev., 130, 103–127, 2014.
Boelter, D. H.: Physical properties of peats as related to degree of decomposition, Soil Sci. Soc. Am. Proc., 33, 606–609, 1969.
Boës, X., Rydberg, J., Martínez-Cortizas, A., Bindler, R., and Renberg, I.: Evaluation of conservative lithogenic elements (Ti, Zr, Al, and Rb) to study anthropogenic element enrichments in lake sediments, J. Paleolimn., 46, 75–87, 2011.
Bränvall, M. L., Bindler, R., Emteryd, O., and Renberg, I.: For thousand years of atmospheric lead pollution in northern Europe: a summary from Swedish lake sediments, J. Paleolimnol., 25, 421–435, 2001.
Breitenlechner, E., Stöllner, T., Thomas, P., Lutz, J., and Oeggl, K.: An interdisciplinary study on the environmental reflection of prehistoric mining activities at the Mitterberg main lode (Salzburg, Austria), Archaeometry, 56, 102–128, 2014.
Broder, T., Blodau, C., Biester, H., and Knorr, K. H.: Peat decomposition records in three pristine ombrotrophic bogs in southern Patagonia, Biogeosciences, 9, 1479–1491, https://doi.org/10.5194/bg-9-1479-2012, 2012.
Büntgen, U., Esper, J., Frank, D. C., Nicolussi, K., and Schmidhalter, M. A.: 1052-year tree-ring proxy for Alpine summer temperatures, Clim. Dynam., 25, 141–153, 2005.
Bunting, M. J., Tipping, R., and Downes, J.: “Anthropogenic” Pollen Assemblages form the Bronze Age Cemetery at Linga Fiold, West Mainland, Orkney, J. Archaeol. Sci., 28, 487–500, 2001.
Cloy, J. M., Farmer, J. G., Graham, M. C., MacKenzie, A. B., and Cook, G. T.: A comparison of antimony and lead profiles over the past 2500 years in Flanders Moss ombrotrophic peat bog, Scotland, J. Environ. Monit., 7, 1137–1147, 2005.
Cloy, J. M., Farmer, J. G., Graham, M. C., MacKenzie, A. B., and Cook, G. T.: Historical records of atmospheric Pb deposition in four Scottish ombrotrophic peat bogs: An isotopic comparison with other records from western Europe and Greenland, Global Biogeochem. Cy., 22, 1–16, 2008.
Cloy, J., Farmer, J. G., Graham, M., and Mackenzie, A. B.: Rentention of As and Sb in Ombrotrophic Peat Bogs: Records of As, Sb, and Pb Deposition at Four Scottish Sites, Environ. Sci. Technol., 43, 1756–1762, 2009.
Czernik, J. and Goslar, T.: Preparation of graphite targets in the Gliwice Radiocarbon Laboratory for AMS 14C dating, Radiocarbon, 43, 283–291, 2001.
De Vleeschouwer, F.: Peat as an archive of atmospheric pollution and environmental change: A case study of lead in Europe, PAGES Mag., 18, 20–22, 2010.
De Vleeschouwer, F., Gerard, L., Goormaghtigh, C., Mattielli, N., Le Roux, G., and Fagel, N.: Atmospheric lead and heavy metal pollution records from a Belgian peat bog spanning the last two millenia: human impact on a regional to global scale, Sci. Total Environ., 377, 282–295, 2007.
De Vleeschouwer, F., Piotrowska, N., Sikorski, J., Pawlyta, J., Cheburkin, A., Le Roux, G., Lamentowicz, M., Fagel, N., and Mauquoy, D.: Multiproxy evidence of `Little Ice Age` palaeoenvironmental changes in a peat bog from northern Poland, Holocene, 19, 625–637, 2009.
Díaz-Somoano, M., Kylander, M. E., López-Antón, M. A., Suárez-Ruiz, I., Martínez-Tarazona, M. R., Ferrat, M., Kober, B., and Weiss, D. J.: Stable lead isotope compositions in selected coals from around the world and implications for present day aerosol source tracing, Environ. Sci. Technol., 43, 1078–1085, 2009.
Drescher-Schneider, R.: Die Vegetations- und Besiedelungsgeschichte der Region Eisenerz auf der Basis pollenanalytischer Untersuchungen im Leopoldsteiner See und in der Eisenerzer Ramsau., in: Montanarchäologie in den Eisenerzer Alpen, Steiermark. Archäologische und naturwissenschaftliche Untersuchungen zum prähistorischen Kupferbergbau in der Eisenerzer Ramsau, edited by: Klemm, S., Verlag der Österreichischen Akademie der Wissenschaften, Vienna, 174–197, 2003.
Drexler, J. Z., Alpers, C. N., Neymark, L. A., Paces, J. B., Taylor, H. E., and Fuller, C. C.: A millennial-scale record of Pb and Hg contamination in peatlands of the Sacramento-San Joaquin Delta of California, USA, Sci. Total Environ., 551–552, 738–751, 2016.
Drollinger, S., Kuzyakov, Y., and Glatzel, S.: Effects of peat decomposition on δ13C and δ15N depth profiles Alpine bogs, Catena, 178, 1–10, 2019.
Drollinger, S., Knorr, K. H., Knierzinger, W., and Glatzel, S.: Peat decomposition proxies of Alpine bogs along a degradation gradient, Geoderma 369, 1–12, https://doi.org/10.1016/j.geoderma.2020.114331, 2020.
Drzymulska, D.: Peat decomposition – shaping factors, significance in environmental studies and methods of determination; a literature review, Geologos, 22, 61–69, 2016.
Engel, Z., Skrzypek, G., Paul, D., Drzewicki, W., and Nývlt, D.: Sediment lithology and stable isotope composition of organic matter in a core from a cirque in the Krkonoše Mountains, Czech Republic, J. Paleolim., 43, 609–624, 2010.
Ettler, V.: Soil contamination near non-ferrous metal smelters: A review, App. Geochem., 64, 56–74, 2016.
Festi, D., Putzer, A., and Oeggl, K.: Mid and late Holocene land-use changes in the Ötztal Alps, territory of the Neolithic Iceman “Ötzi”, Quaternary Int., 353, 17–33, 2014.
Finsinger, W., Tinner, W., van der Knaap, W. O., and Ammann, B.: The expansion of hazel (Corylus avellana L.) in the southern Alps: a key for understanding its early Holocene history in Europe?, Qaternary Sci. Rev., 25, 612–631, 2006.
Flament, P., Bertho, M. L., Deboudt, K., Véron, A., and Puskaric, E.: European isotopic signatures for lead in atmospheric aerosols: a source appointment based upon 206Pb/207Pb ratios, Sci. Total Environ., 296, 35–57, 2002.
Forel, B., Monna, F., Petit, C., Bruguier, O., Losno, R., Fluck, P., Begeot, C., Richard, H., Bichet, V., and Chateau, C.: Historical mining and smelting in the Vosges Mountains (France) recorded in two ombrotrophic peat bogs, J. Geochem. Explor., 107, 9–20, 2010.
Galuszka, A., Migaszewski, Z. M., and Zalasiewicz, J.: Assessing the Anthropocene with geochemical methods, Geo. Soc. Spec. Publ., 395, 221–238, 2014.
Gao, W. and Huang, Y.: Effects of humic acid and compost on speciation transformation of zinc and lead in soil, Chin. J. Environ. Eng., 3, 549–554, 2009.
Gasser, D., Gusterhuber, J., Krische, O., Puhr, B., Scheucher, L., Wagner, T., and Stüwe, K.: Geology of Styria: An overview, Mit. Naturw. Ver. Steierm., 139, 5–36, 2009.
Geologische Bundesanstalt: Geological maps of Austria, BundesAT_GBA_GK100_200: available at: https://gisgba.geologie.ac.at/gbaviewer/?url=https://gisgba.geologie.ac.at/ArcGIS/rest/services/image/AT_GBA_GK100_200/ImageServer, last access: 28 October 2019.
Grimm, E.: TGView, Illinois State Museum, Research and Collections Center, Springfield, Massachusetts, 2004.
Grögler, N., Grünenfelder, M., and Schroll, E.: Ein Hinweis auf Jungpräkambrium und Altpaläozoikum im Altkristallin Kärntens, Tschermaks Mineral. Petrogr. Mitt., 10, 586–594, 1965.
Gulson, B., Kamenov, G. D., Manton, W., and Rabinowitz, M.: Concerns about Quadrupole ICP-MS Lead Isotopic Data and Interpretations in the Environment and Health Fields, Int. J. Environ. Res. Pub. Health, 15, 1–18, 2018.
Hanning, E.: Reconstructing Bronze Age Copper Smelting in the Alps: an ongoing process, Experim. Archäo. Eur., 11, 75–86, 2012.
Haubner, R., Strobl, S., Klemm, S., and Trebsche, P.: Prähistorische Kupfergewinnung im südöstlichen Niederösterreich – archäometallurgische Untersuchungen an alten und neuen Fundstücken, in: Beiträge zum Tag der Niederösterreichischen Landesarchäologie 2015, edited by: Lauermann, E. and Trebsche, P. E., 26–33, Katalog des Niederösterreichischen Landesmuseums N. F. 521, Asparn/Zaya, 26–33, 2015.
Heiri, O., Lotter, A. F., Hausmann, S., and Kienast, F.: A chironomid-based Holocene summer air temperature reconstruction from the Swiss Alps, Holocene, 13, 477–484, 2003.
Herbert, B.: Urgeschiche und Römerzeit in der Steiermark. Geschichte der Steiermark 1, Böhlau Verlag, Vienna, 2015.
Herzschuh, U.: Reliability of pollen ratios for environmental reconstructions on the Tibetan Plateau, J. Biogeog., 34, 1265–1273, 2007.
Ho, Y. S., McKay, G., Wase, D. A. J., and Foster, C. F.: Study of the Sorption of Divalent Metal Ions on to Peat, Adsor. Sci. Technol., 18, 639–650, 2000.
Hodgkins, S. B., Richardson, C. J., Dommain, R., Wang, H., Glaser, P. H., Brittany, V., Winkler, B. R., Cobb, A. R., Rich, V. I., Missilmani, M., Flanagan, N., Ho, M., Hoyt, A. M., Harvey, C. F., Vining, S. R., Hough, M. A., Moore, T. R., Richard, P . J. H., De La Cruz, F. B., Toufaily, J., Hamdan, R., Cooper, W. T. C., and Chanton, J. P.: Tropical peatland carbon storage linked to global latitudinal trends in peat recalcitrance, Nat. Commun., 9, 1–13, https://doi.org/10.1038/s41467-018-06050-2 , 2018.
Holmgren, A. and Norden, B.: Characterization of peat samples by diffuse reflectance FTIR spectroscopy, App. Spectrosc., 42, 255–262, 1988.
Hogue, B. A. and Inglett, P. W.: Nutrient release from combustion residues of two contrasting herbaceous vegetation types, Sci. Total Environ., 431, 9–19, 2012.
Huang, T., Cheng, S., Mao, X., Hong, B., Hu, Z., and Zhou, Y.: Humification degree of peat and its implications for Holocene climate change in Hani peatland, Northeast China, Chin. J. Geochem., 32, 406–412, 2013.
Huber, K., Weckström, K., Drescher-Schneider, R., Knoll, J., Schmidt, J., and Schmidt, R.: Climate changes during the last glacial termination inferred from diatom-based temperatures and pollen in a sediment core from Längsee (Austria), J. Paleolimn., 43, 131–147, 2010.
Ivy-Ochs, S., Kerschner, H., Maisch, M., Christl, M., Kubik, P. W., and Schlüchter, C.: Latest Pleistocene and Holocene glacier variations in the European Alps, Quaternary Sci. Rev., 28, 2137–2149, 2009.
Judd, C. D. and Swami, K.: ICP-MS determinattion of lead isotope ratios in legal and couterfeit cigarette tobacco samples: Isotopes, Environ. Health Stud., 46, 484–494, 2010.
Kalbitz, K., Geyer, W., and Geyer, S.: Spectroscopic properties of dissolved humic substances – a reflection of land use history in a fen area, Biogeochemistry, 47, 219–238, 1999.
Keil, M. and Neubauer, F.: Initiation and development of a fault-controlled, orogen-parallel overdeepened valley: the upper Enns Valley, Austria, Aust. J. Earth Sci., 102, 80–90, 2009.
Kempter, H.: Der Verlauf des anthropogenen Elementeintrags in Regenwassermoore des westlichen Mitteleuropas während des jüngeren Holozäns, Gustav Fischer Verlag, Stuttgart, 1996.
Klemm, S.: Montanarchäologie in den Eisenerzer Alpen, in: Montanarchäologie in den Eisenerzer Alpen, Steiermark. Archäologische und naturwissenschaftliche Untersuchungen zum prähistorischen Kupferbergbau in der Eisenerzer Ramsau, edited by: Klemm, S., Verlag der Österreichischen Akademie der Wissenschaften, Vienna, 11–43, 2003.
Knierzinger, W.: (Bio)geochemical data Pürgschachen Moor, PANGAEA, https://doi.org/10.1594/PANGAEA.919320, 2020.
Krachler, M., Mohl, C., Emons, H., and Shotyk, W.: Analytical procedures for the determination of selected trace elements in peat and plant samples by inductively coupled plasma mass spectrometry, Spectrochim. Acta B, 57, 1277–1289, 2002.
Kraus, S.: Archäometallurgische Studien zur bronzezeitlichen Kupferverhütungstechnologie am Kupferschmelzplatz S1 in der Eisenerzer Ramsau (Steiermark, Österreich), Dissertation, Eberhard Karls Universität Tübingen, Germany, 147 pp., 2013, Tübingen, 2014.
Kucha, H. and Raith, J. G.: Gold-oxysulphides in copper deposits of the greywacke Zone, Austria: a mineral chemical and infrared fluid inclusion study, Ore Geo. Rev., 35, 87–100, 2009.
Kutschera, W., Patzelt, G., Steier, P., and Wild, E. M.: The Tyrolean Iceman and his glacial environment during the Holocene, Radiocarbon, 59, 395–405, 2017:
Kylander, M. E., Weiss, D. J., Martínez-Cortizas, A., Spiro, B., Garcia-Sanchez, R., and Coles, B. J.: Refining the pre-industrial atmospheric Pb isotope evolution curve in Europe using a 8000 year old peat core from NW Spain, Earth Plan. Sci. Lett., 240, 467–485, 2005.
Kylander, M. E., Martínez-Cortizas, A., Bindler, R., Greenwod, S. L., Mörth, C. M., and Rauch, S.: Potentials and problems of building detailed dust records using peat archives: An example from Store Mosse (the “Great Bog”) Sweden, Geochim. Cosmochim. Acta, 190, 156–174, 2016.
Laflamme, J. H. G. and Cabri, L. J.: Silver and antimony contents of galena from the Brunswick No. 12 mine, CANMET Mineral Sci. Lab. Div. Rep. MSL, 86–138, 1–13, 1986.
Leifeld, J., Gubler, L., and Grünig, A.: Organic matter losses from temperate ombrotrophic peatlands: an evaluation of the ash residue method, Plant. Soil, 341, 349–361, 2011.
Longman, J., Veres, D., Finsinger, W., and Ersek, V.: Exceptionally high levels of lead pollution in the Balkans from the Early Bronze Age to the Industrial Revolution, P. Natl. Acad. Sci. USA, 115, E5661–E5668, 2018.
Lutz, J. and Pernicka, E.: Prehistoric copper from the Eastern Alps, Op. J. Archaeometry, 1, 122–127, 2013.
Mariet, A. L., Bégeot, C., Gimbert, F., Gauthier, J., Fluck, P., and Walter-Simonnet, A. V.: Past mining activities in the Vosges Mountains (eastern France): Impact on vegetation and metal contamination over the past millennium, Holocene, 26, 1225–1236, 2016.
Martínez-Cortizas, A., Pontevedra-Pombal, X., Garcıa-Rodeja, E., Novoa-Munoz, J. C., and Shotyk, W.: Mercury in a Spanish peat bog: archive of climate change and atmospheric metal deposition, Science, 284, 939–942, 1999.
Martínez Cortizas, A., Biester, H., Mighall, T., and Bindler, R.: Climate-driven enrichment of pollutants in peatlands, Biogeosciences, 4, 905–911, https://doi.org/10.5194/bg-4-905-2007, 2007.
Mighall, T. M., Timberlake, S., Foster, I. D. L., Krupp, E., and Singh, S.: Ancient copper and lead pollution records from a raised bog complex in Central Wales, UK, J. Archaeol. Sci., 36, 1504–1515, 2009.
Mighall, T., Martínez-Cortizas, A., Silva-Sánchez, N., Foster, I. D. L., Singh, S., Bateman, M., and Pickin, J.: Identifying evidence for past mining and metallurgy from a record of metal contamination preserved in an ombrotrophic mire near Leadhills, SW Scotland, UK, Holocene, 24, 1719–1730, 2014.
Mihaljevič, M., Zuna, M., Ettler, V., Šebek, O., Strnad, L., and Goliáš, V.: Lead fluxes, isotopic and concentration profiles in a peat deposit near a lead smelter (Příbram, Czech Republic), Sci. Total Environ., 372, 334–344, 2006.
Millot, R., Allègre, C. J., Gaillardet, J., and Rox, S.: Lead isotopic systematics of major river sediments: a new estimate of the Pb isotopic composition of the Upper Continental Crust, Chem. Geo., 203, 75–90, 2004.
Miola, A.: Tools for Non-Pollen Palynomorphs (NPPs) analysis: A list of Quaternary NPP types and reference literature in English language (1972–2011), Rev. Palaeobot. Palyno., 186, 142–161, 2012.
Monna, F., Lancelot, J., Croudace, I. W., Cundy, A. B., and Lewis, J. T.: Pb Isotopic Composition of Airborne Particulate Material from France and the Southern United Kingdom: Implications for Pb Pollution Sources in Urban Areas, Environ. Sci. Technol., 31, 2277–2287, 1997.
Monna, F., Galop, D., Carozza, L., Tual, M., Beyrie, A., Marembert, F., Chateau, C., Dominik, J., and Grousset, F. E.: Environmental impact of early Basque mining and smelting recorded in a high ash minerogenic peat deposit, Sci. Total Environ., 327, 197–214, 2004.
Nicolussi, K., Kaufmann, M., Patzelt, G., van der Plicht, J., and Thurner, A.: Holocene tree-line variability in the Kauner Valley, Central Eastern Alps, indicated by dendrochronological analysis of living trees and subfossil logs, Veget. Hist. Archaeobot., 14, 221–234, 2005.
Niemeyer, J., Chen, Y., and Bollag, J. M.: Characterization of humic acids, composts, and peat by diffuse reflectance Fourier-transform infrared spectroscopy, Soil Sci. Soc. Am. J., 56, 135–140, 1992.
Novak, M., Zemanova, L., Voldrichova, P., Stepanova, M., Adamova, M., Pacherova, P., Komarek, A., Krachler, M., and Prechova, E.: Experimental evidence for mobility/immobility of metals in peat, Environ. Sci. Technol., 45, 7180–7187, 2011.
O'Brien, W.: Prehistoric Copper Mining in Europe. 5500–500 BC, Oxford University Press, Oxford, 2015.
Olid, C., Garcia-Orellana, J., Martínez-Cortizas, A., Masqué, P., Peiteado-Varela, E., and Sanchez-Cabeza, J. A.: Multiple site study of recent atmospheric metal (Pb, Zn and Cu) deposition in the NW Iberian Peninsula using peat cores, Sci. Total Environ., 408, 5540–5549, 2010.
Pernicka, E., Lutz, J., and Stöllner, T.: Bronze Age Copper Produced At Mitterberg, Austria, and its Distribution, Arach. Austriaca, 100, 19–55, 2016.
Pichler, T., Nicolussi, K., Schröder, J., Stöllner, T., Thomas, P., and Thurner, A.: Tree-ring analyses on Bronze Age mining timber from the Mitterberg Main Lode, Austria – did the miners lack wood?, J. Arch. Sci Rep., 19, 701–711, 2018.
Pilarski, J., Waller, P., and Pickering, W.: Soprtion of antimony species by humid acid, Water Air Soil Poll., 84, 51–59, 1995.
Pratte, S., Garneau, M., and De Vleeschouwer, F.: Late-Holocene atmospheric dust deposition in eastern Canada (St. Lawrence North Shore), Holocene, 27, 12–25, 2017.
Preßlinger, H. and Köstler, H. J.: Zur Geschichte des Eisenerzbergbaus am Blahberg bei Admont, Res. montanarum, 27, 21–26, 2002.
Preßlinger, H., Eibner, C., and Preßlinger, B.: Archäologische Belege der bronzezeitlichen Kupfererzverhüttung im Paltental, Res. Montanarum, 46, 35–45, 2009.
R Core Team: R: A Language and Environment for Statistical Computing, available at: https://www.r-project.org (last access: 11 April 2020), 2018.
Rausch, N., Ukonmaanaho, L., Nieminen, T. M., Krachler, M., and Shotyk, W.: Porewater evidence of metal (Cu, Ni, Co, Zn, Cd) mobilization in an acidified, ombrotrophic peat bog impacted by a smelter, Harjavalta, Finland, Environ. Sci. Technol., 39, 8207–8213, 2005.
Reimer, P. J., Bard, E., Bayliss, A., Beck, J. W., Blackwell, P. G., Bronk Ramsey, C., Buck, C. E., Cheng, H., Edwards, R. L., Friedrich, M., Grootes, P. M., Guilderson, T. P., Haflidason, H., Hajdas, I., Hatte, C., Heaton, T. J., Hoffmann, D. L., Hogg, A. G., Hughen, K. A., Kaiser, K. F., Kromer, B., Manning, S. W., Niu, M., Reimer, R. W., Richards, D. A., Scott, E. M., Southon, J. R., Staff, R. A., Turney, C. S. M., and van der Plicht, J.: IntCal13 and Marine13 radiocarbon age calibration curves 0–50,000 years cal BP, Radiocarbon, 55, 1869–1887, 2013.
Röpke, A. and Krause, R.: High montane–subalpine soils in the Montafon Valley (Austria, northern Alps) and their link to land-use, fire and settlement history, Quaternary Int., 308–309, 178–189, 2013.
Rösch, M. and Lechterbeck, J.: Seven Millennia of human impact as reflected in a high resolution pollen profile from the profundal sediments of Litzelsee, Lake Constance region, Germany, Veg. Hist. Archaeobot., 25, 339–358, 2016.
Rothwell, J. J., Taylor, K. G., Chenery, S. R. N., Cundy, A. B., Evans, M. G., and Allott, T. E. H.: Storage and Behavior of As, Sb, Pb, and Cu in Ombrotrophic Peat Bogs under Contrasting Water Table Conditions, Environ. Sci. Technol., 44, 8497–8502, 2010.
Schmidt, R., Kamenik, C., Tessadri, R., and Koinig, K. A.: Climatic changes from 12,000 to 4,000 years ago in the Austrian Central Alps tracked by sedimentological and biological proxies of a lake sediment core, J. Paleolimon., 35, 491–505, 2006.
Schnyder, E., Štrok, M., Kosonen, Z., Skudnik, M., Mazej, D., Jeran, Z., and Thöni, L.: Lead concentrations and stable lead isotope ratios in moss in Slovenia and Switzerland, Ecol. Indicat., 95, 250–259, 2018.
Schumann, W.: Der neue BLV Steine- und Mineralienführer, BLV Verlagsgesellschaft mbH, München, 2002.
Segnana, M., Oeggl, K., Poto, L., Gabrieli, J., Festi, D., Kofler, W., Cesco-Frare, P., Zaccone, C., and Barbante, C.: Holocene vegetation history and human impact in the eastern Italian Alps: a multi-proxy study on the Coltrondo peat bog, Comelico Superiore, Italy, Veget. Hist. Archeobot., 29, 407–426, https://doi.org/10.1007/s00334-019-00749-y, 2019.
Sharp, T. G. and Buseck, P. R.: The distribution of Ag and Sb in galena: Inclusions versus solid solution, Am. Mineral., 78, 85–95, 1993.
Shotyk, W., Weiss, D., Kramers, J. D., Frei, R., Cheburkin, Gloor, M., and Reese, S.: Geochemistry of the peat bog at Etang de Gruere, Jura Mountains, Switzerland, and its record of atmsopheric Pb and lithogenic trace metals (Sc, Ti, Y, Zr, and REE) since 12,370 14C yr BP, Geochim. Cosmochim. Acta, 65, 2337–2360, 2001.
Shotyk, W., Weiss, D., Heisterkamp, M., Cherbukin, A. K., Appleby, P. G., and Adams, F. C.: New Peat Bog Record of Atmospheric Lead Pollution in Switzerland: Pb Concentrations, Enrichment Factors, Isotopic Composition, and Organolead Species, Eviron. Sci. Technol., 36, 3893–3900, 2002.
Shotyk, W., Krachler, M., and Chen, B.: Antimony in recent, ombrotrophic peat from Switzerland and Scotland: Comparison with natural background values (5,320 to 8,020 14C yr BP) and implication for the global atmospheric Sb cycle, Global Biogeochem. Cy., 18, 1–13, 2004.
Shotyk, W., Kempter, H., Krachler, M., and Zaccone, C.: Stable (206Pb, 207Pb, 208Pb) and radioactive (210Pb) lead isotopes in 1 year of growth of Sphagnum moss from four ombrotrophic bogs in southern Germany: Geochemical significance and environmental implications, Geochim. Cosmochim. Acta, 163, 101–125, 2015.
Shotyk, W., Appleby, P. G., Bicalho, B., Davies, L. J., Froese, D., Grant-Weaver, I., Mangan, G., Mullan-Bourdreau, G., Noernberg, T., Pelletier, R., Shannon, B., van Bellen, S., and Zaccone, C.: Peat Bogs Document Decades of Declining Atmospheric Contamination by Trace Metals in the Athabasca Bituminous Sands Region, Environ. Sci. Technol., 51, 6237–6249, 2017.
Sîre, J., Klavins, M., Purmalis, O., and Melecis, V.: Experimental study of peat humification indicators, Proc. Latv. Acad. Sci. Sect. B Nat. Exact. Appl. Sci., 62, 18–26, 2008.
Smieja-Król, B. and Fiałkiewicz-Kozieł, B.: Quantitative determination of minerals and anthropogenic particles in some Polish peat occurrences using a novel SEM point-counting method, Environ. Monit. Assess., 188, 2573–2587, 2014.
Stattegger, K.: Schwermineraluntersuchungen in der Östlichen Grauwackenzone (Steiermark/Österreich) und deren statistische Auswertung, Verh. Geol. B.-A., 1982, 107–121, 1982.
Steinnes, E.: Trace element profiles in ombrogenous peat cores from Norway: Evidence of long range atmospheric transport, Water Air Soil Poll., 100, 405–413, 1997.
Stivrins, N., Ozola, I., Galka, M., Kuske, E., Alliksaar, T., Andersen, T. J., Lamentowicz, M., Wulf, S., and Reitalu, T.: Drivers of peat accumulation rate in a raised bog: impact of drainage, climate, and local vegetation composition, Mires Peat, 19, 1–19, 2017.
Stockmarr, J.: Tablets with spores used in absolute pollen analysis, Pollen et Spores, 13, 615–621, 1971.
Stöllner, T.: Der Mitterberg als Großproduzent für Kupfer in der Bronzezeit: Fragestellungen und bisherige Ergebnisse, in: Die Geschichte des Bergbaues in Tirol und seinen angrenzenden Gebieten. Proceedings zum 5. Milestone-Meeting des SFB HiMAT vom 07.–10.10.2010 in Mühlbach, edited by: Oeggl, K., Goldenberg, G., Stöllner, T., and Prast, M., Innsbruck University Press, Innsbruck, 93–106, 2011.
Tipping, E., Smith, E. J., Lawlor, A. J., Hughes, S., and Stevens, P. A.: Predicting the release of metals from ombrotrophic peat due to drought-induced acidification, Environ. Pollut., 123, 239–253, 2003.
Tolonen, K.: Interpretation of changes in the ash content of ombrotrophic peat layers, B. Geol. Soc. Finland, 56, 207–219, 1984.
Ukonmaanaho, L., Nieminen, T. M., Rausch, N., and Shotyk, W.: Enrichment of Cu, Ni, Zn, Pb and As in an ombrotrophic peat bog near a Cu-Ni smelter in Southwest Finland, Sci. Total Environ., 292, 81–89, 2002.
Ukonmaanaho, L., Nieminen, T. M., Rausch, N., and Shotyk, W.: Heavy metal and arsenic profiles in ombrogenous peat cores from four differently loaded areas in Finland, Water Air Soil Pollut., 158, 277–294, 2004.
van der Knaap, W. O., Lametowicz, M., van Leeuwn, J. F. N., Hangartner, S., Leuenberger, M., Mauquoy, D., Goslar, T., Mitchell, E. A. D., Lamentowicz, L., and Kamenik, C.: A multi-proxy, high resolution record of a peatland development and its drivers during the last millennium from the subalpine Swiss Alps, Quaternary Sci. Rev., 30, 3467–3480, 2011.
van der Linden, M. and van Geel, B.: Late Holocene climate change and human impact recorded in a south Swedish ombrotrophic peat bog, Palaeogeogr. Palaeoclimatol. Palaeoecol., 240, 649–667, 2006.
Von Post, L.: Das genetische System der Organogenen Bildungen Schwedens. Memoires sur la nomenclature et la classification des sols, International Committee of Soil Science, 287–304, Helsinki, 1924.
von Scheffer, C., Lange, A., De Vleeschouwer, F., Schrautzer, J., and Unkel, I.: 6200 years of human activities and environmental change in the northern central Alps, E&G Quat. Sci. J., 68, 13–28, 2019.
Wagreich, M.: Sedimentologie und Beckenentwicklung des tieferen Abschnittes (Santon-Untercampan) der Gosauschichtgruppe von Gosau und Rußbach (Oberösterreich-Salzburg), Jb. Geol. B.-A., 131, 663–685, 1988.
Wagreich, M. and Draganits, E.: Early mining and smelting lead anomalies in geological archives as potential stratigraphic markers for the base of an Early Anthropocene, Anthropocene Rev., 5, 177–201, 2018.
Walker, M., Head, M. J., Lowe, J., Berkelhammer, M., Björck, S., Cheng, H., Cwynar, L., Fisher, D., Gkinis, V., Long, A., Newham, R., Rasmussen, S., and Weiss, H.: Subdividing the Holocene Series/Epoch: formalization of stages/ages and subseries/subepochs, and designation of GSSPs and auxiliary stratotypes, J. Quat. Sci., 34, 1–14, 2019.
Weiss, D., Shoytk, W., Appleby, P. G., Kramers, J. D., and Cheburkin, A. K.: Atmospheric Pb deposition since the Industrial Revolution recorded in five Swiss peat profiles: Enrichment factors, fluxes, isotopic composition, and sources, Environ. Sci. Technol., 33, 1340–1352, 1999.
Xiao, H., Cheng, S., Mao, X., Huang, T., Hu, Z., Zhou, Y., and Liu, X.: Characteristics of peat humification, magnetic susceptibility and trace elements of Hani peatland, northeastern China: paleoclimatic implications, Atmos. Sci. Lett., 18, 140–150, 2017.
Yang, T. and Hodson, M. E.: The copper complexation ability of a synthetic humic-like acid formed by an abiotic humification process and the effect of experimental factors on its copper complexation ability, Environ. Sci. Poll. Res., 25, 15873–15884, 2018.
Yang, Y., Wang, B., Guo, X., Zou, C. W., and Tan, X. D.: Investigating adsorption performance of heavy metals onto humic acid from sludge using Fourier-transform infrared combinend with two-dimensional correlation spectroscopy, Envrion. Sci. Poll. Res., 26, 9842–9850, 2019.
Zhang, Y., Kong, Z., Wang, G., and Ni, J.: Anthropogenic and climatic impacts on surface pollen assemblages along a precipitation gradient in north-eastern China, Global Ecol. Biogeogr., 19, 621–631, 2010.