the Creative Commons Attribution 4.0 License.
the Creative Commons Attribution 4.0 License.
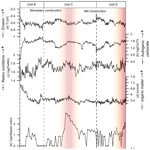
A 1100-year multi-proxy palaeoenvironmental record from Lake Höglwörth, Bavaria, Germany
Sudip Acharya
Maximilian Prochnow
Thomas Kasper
Linda Langhans
Peter Frenzel
Paul Strobel
Marcel Bliedtner
Gerhard Daut
Christopher Berndt
Sönke Szidat
Gary Salazar
Antje Schwalb
Roland Zech
Acharya, S., Prochnow, M., Kasper, T., Langhans, L., Frenzel, P., Strobel, P., Bliedtner, M., Daut, G., Berndt, C., Szidat, S., Salazar, G., Schwalb, A., and Zech, R.: A 1100-year multi-proxy palaeoenvironmental record from Lake Höglwörth, Bavaria, Germany, E&G Quaternary Sci. J., 72, 219–234, https://doi.org/10.5194/egqsj-72-219-2023, 2023.
Anthropogenic activities have exerted strong influence on ecosystems worldwide, particularly since 1950 CE. The local impact of past human activities often started much earlier and deserves detailed study. Here, we present an environmental record from a 278 cm long sedimentary core from Lake Höglwörth (Bavaria, Germany). Sedimentological and geochemical parameters indicate that the organic-rich bottom sediments of the record consist of peat that formed prior to CE, when lake sediments started to accumulate. After CE, distinct shifts in lithology, elemental composition, and the biological record are visible and are interpreted to result from the construction of a monastery on the lake peninsula in 1125 CE and/or the damming of the lake. From 1120±120 to CE, the lake environment was relatively stable. This period was followed by enhanced deforestation that led to a more open landscape and soil erosion, visible in increased allochthonous input from to CE. This was accompanied by high aquatic productivity and bottom or interstitial water anoxia from to CE, possibly triggered by increased nutrient availability. Enhanced allochthonous input and a substantial shift in the aquatic community can be assigned to the construction of a flour mill and related rerouting of a small creek in 1701 CE. High aquatic productivity and bottom or interstitial water anoxia after 1960±10 CE correspond to recent eutrophication resulting from accelerated local anthropogenic activities. The sedimentary record from Lake Höglwörth exemplarily demonstrates that anthropogenic activities have had substantial environmental impacts on aquatic environments during the past millennium.
Anthropogene Aktivitäten haben insbesondere seit dem Jahr 1950 CE weltweit einen starken Einfluss auf Ökosysteme. Jedoch hatten menschliche Aktivitäten oft schon viel früher Auswirkungen auf Ökosysteme und verdienen eine detaillierte Untersuchung. Hier präsentieren wir sedimentologische, geochemische und paläontologische Ergebnisse eines 278 cm langen Sedimentbohrkerns aus dem Höglwörther See (Bayern, Deutschland), der die letzten 1100 Jahre abdeckt. Sedimentologische und geochemische Parameter deuten darauf hin, dass die unteren organikreichen Sedimente aus Torf bestehen, der sich vor etwa CE bildete, bevor sich die Seesedimente ablagerten. Nach CE sind deutliche Änderungen in der Lithologie, der Elementkomposition und der biologischen Zusammensetzung zu erkennen, die auf den Bau des Klosters Höglwörth auf der Halbinsel des Sees um 1125 CE und/oder eine Aufstauung des Sees zurückzuführen sind. Von 1120±120 bis CE war die Ökologie des Sees relativ stabil. Danach folgte eine verstärkte Entwaldung, die zu einer offeneren Landschaft und stärkerer Bodenerosion führte, was durch erhöhten allochthonen Eintrag von etwa to CE sichtbar ist. Dies führte zu einer hohen aquatischen Produktivität und Anoxie des Hypolimnions von etwa to CE – möglicherweise ausgelöst durch eine erhöhte Nährstoffverfügbarkeit. Der Bau einer Getreidemühle und die damit verbundene Umleitung eines kleinen Baches im Jahr 1701 CE führten zu einem verstärkten allochthonen Eintrag und einer erheblichen Veränderung der aquatischen Gesellschaft. Die hohe aquatische Produktivität und die Anoxie des Hypolimnions nach 1960±10 CE zeigen die rezente Eutrophierung, die auf verstärkte lokale anthropogene Aktivitäten zurückzuführen ist. Die Sedimente des Höglwörther Sees zeigen somit beispielhaft, dass anthropogene Aktivitäten während des letzten Jahrtausends erhebliche Auswirkungen auf aquatische Ökosysteme gehabt haben.
- Article
(6667 KB) - Full-text XML
-
Supplement
(1231 KB) - BibTeX
- EndNote
Abrupt shifts in ecosystems all over the globe during the past decades are related to human activities and are in stark contrast with ranges of the natural variability evident at least during the Holocene (Steffen et al., 2015). To define this marked shift in Earth's environment, the term “Anthropocene” has been suggested for a new geological era, which is proposed to have started around 1950 CE and has prompted intense scientific and societal debate (Waters and Turner, 2022; Crutzen and Stoermer, 2021; Lewis and Maslin, 2015). However, the onset of human impact often occurred much earlier, both locally and regionally (Ruddiman et al., 2020; Waters et al., 2016), and requires more detailed, local palaeoenvironmental studies.
Bavaria is a region significantly impacted by humans as far back as the early medieval period (∼ 1000 CE) (Bauer and Bauer, 1993) with increased settlements and agricultural activities (Gilck and Poschlod, 2020; Klein Goldewijk et al., 2011). The shift in land cultivation practices from subsistence agriculture with livestock and crop production to cattle grazing for meat and dairy production during the medieval period led to the establishment of pastureland (Enters et al., 2008, 2006). This is documented in lacustrine records as a decrease in tree pollen and an intensification of soil erosion due to the widespread land use (Dotterweich, 2003). A significant decrease in population during the late medieval period (from 1300 to 1450 CE) and beyond can be attributed to military conflicts, epidemic plagues, and cooler climate conditions associated with the Little Ice Age and led to the abandonment of numerous villages and settlements (Küster, 2020; Franz, 2019; Enters et al., 2006; Jäger, 1958). These results are supported by findings of archeological excavations and historical documents from the region (Blei, 2011; Waldner, 1983). An increase in human impacts such as nutrient loading, excessive use of fertilizers, and changes in land use/land cover has led to a substantial alteration in aquatic ecosystems, including the loss of biodiversity, eutrophication, and anoxia over the past few decades (Enters et al., 2006; Alefs and Müller, 1999). Although these significant human activities have been documented, it remains unclear if and how the human activities during the past millennium impacted the natural ecosystem in the region. Also, the available palaeoenvironmental records are mainly derived from large lakes (Rösch et al., 2021; Schubert et al., 2020; Lauterbach et al., 2011; Richard, 1996), which capture regional signals and exhibit different environmental responses compared to small lakes that record local signals (Adrian et al., 2009; Enters et al., 2006).
Here, we present a study from Lake Höglwörth, a small, eutrophic lake situated in south-eastern Bavaria, Germany (Wasserwirtschaftsamt Traunstein, 2018). The lake has undergone severe ecological alternation (algal production and anoxia) over the past decades (Fam and Kerstin, 2018; Wasserwirtschaftsamt Traunstein, 2018). A 278 cm long sediment record from Lake Höglwörth was analysed using sedimentological, geochemical, and biological methods. Specifically, our study aims to investigate (1) whether the recent algal production and anoxia in the lake did occur in the past and (2) whether and how past human activities affected the lithological, geochemical, and biological environment of Lake Höglwörth over the past millennium.
2.1 Site description
Lake Höglwörth (47.81∘ N, 12.84∘ E; 531 m above sea level; Fig. 1) is a small (surface area 0.14 km2), irregularly shaped, and eutrophic lake, located in a paraglacial meltwater channel that formed during the Last Glacial Maximum when the Salzach Glacier forced drainage along its ice margin in a north-western direction at the northern forelands of the European Alps in south-eastern Bavaria, Germany. Postglacial landslide and mudflow deposits of still unknown age (presumably during the Middle to Late Holocene) are present north-west of the lake and are thought to be responsible for blocking the valley and former damming of the lake as seen in lidar imagery (Landesamt für Digitalisierung, 2022). Today, the lake has a maximum water depth of 6 m; has an average depth of 3.1 m (for a description of bathymetry, please refer to the Supplement); and is fed by three small creeks, i.e. Höglwörther Schornbach, Höglwörther Seebach, and Moosgraben. Lake Höglwörth is drained by the Rauschbach. The lake has a catchment area of 2.3 km2, and it is geologically characterized by deposits of the last glacial period and patches of Cretaceous to Eocene bedrock (Glückert, 1974). Cambisols and Luvisols are the most common catchment soil types, whereas Stagnosols and Gleysols have developed in the surrounding valleys (Landesamt für Digitalisierung, 2022).
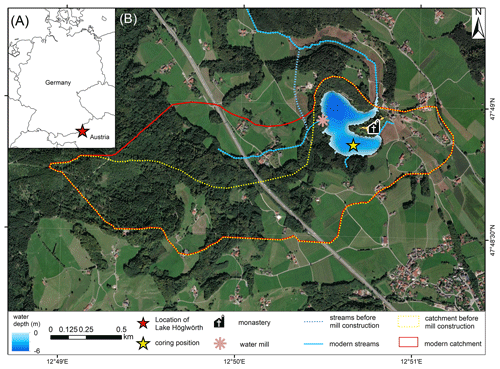
Figure 1(a) Overview of the study area. Red star shows the location of Lake Höglwörth. (b) Image of Lake Höglwörth and its modern catchment indicated by red line (modified from © Google Earth, 2022). Blue line indicates the streams. Dashed yellow and blue lines indicate the catchment and streams, respectively, before mill construction. The coring position is indicated by a yellow star.
On the peninsula of the lake, an Augustinian monastery is located, constructed in 1125 CE (Brugger et al., 2008). On the western side of the lake, a flour mill is situated, built in 1701 CE by diverting the Höglwörther Seebach into the lake (Brugger et al., 2008); consequently the catchment of the lake has enlarged since. The mill was torn down in 1922 CE.
At the meteorological station in Piding, ∼ 10 km south-east of Lake Höglwörth, mean annual precipitation (1991 to 2020) is 1340 mm. Mean annual temperature is 9.2 ∘C, with a temperature maximum during summer (June–July 18.5 ∘C) and a temperature minimum during winter (January–February −0.2 ∘C) (DWD Climate Data Center, 2020).
2.2 Sediment coring, core processing, and dating
Sediment cores were retrieved from Lake Höglwörth in 2019 from a water depth of 4.7 m (Figs. 1 and S2 in the Supplement). For the uppermost (∼ 1 m) sediment section, a UWITEC gravity corer with a diameter of 90 mm was used, while longer sediment cores with a diameter of 63 mm were retrieved in overlapping core sections (2 m each), using a platform-based UWITEC piston coring system. Cores were split, photographed, and lithologically described at the laboratory of the Physical Geography Department of the Friedrich Schiller University Jena. Lithological marker layers within the core sections were used to establish a continuous sediment sequence with a total length of 278 cm (Fig. S2).
In cooperation with the Laboratory for the Analysis of Radiocarbon (LARA), University of Bern, Switzerland, 14C ages were obtained from eight macrofossil samples and one bulk organic matter sample (Table 1) (Salazar et al., 2015; Szidat et al., 2014), using a Mini Carbon Dating System (MICADAS) accelerator mass spectrometer (AMS) coupled online to an Elementar analyser (Ruff et al., 2010). Prior to radiocarbon analysis, samples were treated with ∼4 % HCl at 60 ∘C for 8 h to remove carbonates. Ages obtained from LARA were calibrated using the online version of the software Calib 8.2 (Stuiver and Reimer, 1993), combined with the IntCal20 calibration dataset (Reimer et al., 2020) and the bomb peak Northern Hemisphere 1 calibration dataset (Hua et al., 2013), particularly for the 14C age at 8 cm depth. Additionally, the upper 40 cm sediment of core was analysed for 137Cs activity at Technische Universität Dresden, Germany. Final age–depth modelling was carried out with the help of the software package rbacon 2.4.3 (Blaauw and Christen, 2011).
2.3 Lithology and geochemical analysis
Non-destructive X-ray fluorescence (XRF) scanning was carried out with an ITRAX XRF core scanner at the Geomorphology and Polar Research Group (GEOPOLAR), University of Bremen, Germany. Single halves of core segments were scanned at 5 mm intervals using a molybdenum tube (Mo) as the X-ray source operating at 30 kV voltage, 50 mA current, and an exposure time of 5 s. In order to eliminate sediment matrix effects (i.e. interferences with water content, surface roughness, and grain size variations), raw elemental counts were normalized by logarithmic transformation and are given as centred log ratios (Ramisch et al., 2018; Weltje et al., 2015). XRF scanning produced reliable counts (elements with counts >100 and mean square errors <2) for titanium (Ti), iron (Fe), manganese (Mn), strontium (Sr), calcium (Ca), and potassium (K). The degree of incoherent scattering (inc) and coherent scattering (coh) is used to calculate the inc coh ratio, which is a proxy for organic matter content (Fortin et al., 2013; Guyard et al., 2007). Magnetic susceptibility was determined at 2 mm resolution, using a Bartington MS2E surface scanning sensor (Bartington Instruments Ltd., Witney, UK).
Grain size and elemental analyses were conducted on 1 cm sediment slices collected at an interval of 12 cm. For grain size analyses, ∼ 0.2 g of dry sediment was treated with H2O2 (∼ 10 % and ∼ 30 %) and ∼ 30 % HCl to remove organic matter and carbonates. Grain size measurements were performed using an LS 13 320 Laser Diffraction Particle Size Analyzer (Beckman Coulter, Brea, CA, USA). Samples were measured with the aqueous liquid module in several 60 s cycles until a reproducible signal was obtained. The “Fraunhofer” optical model of light scattering was used for computing grain size distribution. Further statistical calculations were done with a modified version of the software GRADISTAT 4.2 (Blott and Pye, 2001).
Approximately 20 mg of the freeze-dried sediments (−50 ∘C, >72 h) was packed into tin capsules for the measurement of total carbon (TC), total nitrogen (TN), and isotopic composition of bulk nitrogen (δ15N). The total organic carbon (TOC) and isotopic composition of bulk organic carbon (δ13C) were measured on decalcified sediment samples, treated with ∼4 % HCl at 60 ∘C for 8 h, and washed with deionized water until reaching pH neutrality. Carbonate content was calculated as CaCO3 from the difference between TC and TOC, and the ratio was calculated as the molar ratio. The measurements were carried out using an elemental analyser (vario EL cube) coupled to an isotope ratio mass spectrometer (IRMS, isoprime precisION; both devices from Elementar, Langenselbold, Germany). The precision was checked by co-analysing L-Prolin, EDTA, and USGS6 with known isotopic composition. The analytical uncertainty for these standards was <0.1 ‰ for both δ15N and δ13C. The isotopic values of 15N and 13C are expressed in delta notation (δ15N, δ13C) against air and Vienna Pee Dee Belemnite (V-PDB), respectively.
2.4 Biological analysis
Sediment samples of 2–3 cm thickness and a volume of 16 to 47 mL each were stirred with deionized water in an overhead-shaker for 1 to 5 h and sieved for organic and inorganic macrofossils over mesh sizes of 1 mm and 200 µm, respectively. Because samples below 238 cm depth could not be disintegrated, H2O2 (5 %) was added, but disintegration was insufficient, allowing a rough identification of the main sediment components only. After sieving, residues were washed with deionized water and dried at ∼ 50 ∘C. All macrofossils from the 1 mm sieve were identified to the lowest possible taxonomic level. Dried smaller sieve residues served for the picking of specific microfossils. Ostracods, testate amoebae and statoblasts of bryozoans were documented on the species level, while counting of the other remnants (ephippia of Cladocera, oospores of Charophyta, glochidia of Bivalvia, eggs of uncertain origin, remains of fishes such as vertebra and scales, oribatid mites, and charcoal) was on the group level only. Relative abundance data for Ostracoda, testate amoebae, and statoblasts rely on complete counts of all specimens, but usually only up to 300 per group within the samples were counted. All taxa were photographed with the help of a Keyence VHX-6000 digital microscope. Additionally, remains of other molluscs, insects such as weevils, and plants such as fruits and seeds were documented (Table S1 in the Supplement). Identification and autecological interpretation of microfossils rely on Meisch (2000) and Fuhrmann (2012) for Ostracoda, Schönborn (1966) and Scott et al. (2001) for testate amoebae, Glöer (2015) for aquatic molluscs, Klausnitzer (2019) for bryozoan statoblasts (Frenzel, 2019), and datasets available on the Smithsonian Institution's National Museum of Natural History (https://naturalhistory.si.edu/, last access: 4 October 2021) for other groups in a more general way.
3.1 Lithology and dating
The sediment record from Lake Höglwörth shows four different lithological units (Figs. 2–4), which are derived from changes in sediment colour, texture, and properties: Unit A (from 278 to 238 cm) consists of overall dark-brown-to black-coloured sediments with high organic carbon and sand contents, as well as low carbonate and low clay contents (Figs. 3 and 4). Greyish sand deposits were observed in several depths throughout Unit A. A sharp boundary in lithology and sediment colour is present at 238 cm depth and marks a transition from Unit A to Unit B. The sediments in Unit B (from 238 to 196 cm) are light reddish at the bottom and grey-coloured at the top. Unit B has lower sand but higher clay contents compared to Unit A, and silt is the dominant grain size. Silt contents remain very high (>70 %) in Unit C (from 196 to 88 cm). Sediment colour changes distinctly at 196 cm, ranging from grey to black colour in a mottled pattern. The change from dark grey to light grey sediments at 88 cm marks the transition to Unit D (from 88 cm to the top). Brownish colours occur at 60 cm, whereas the top part of the core reveals brighter sediments (Fig. 2).
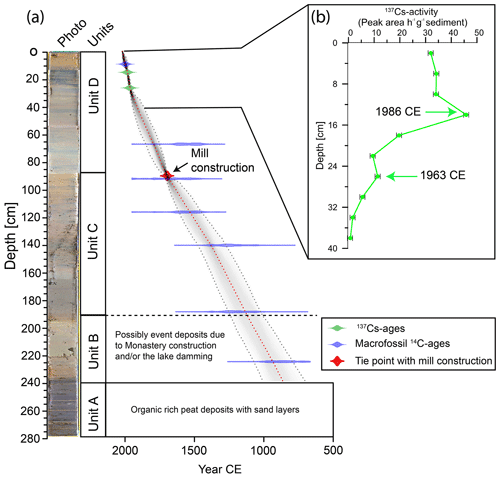
Figure 2(a) Photo of the sediment core from Lake Höglwörth, units, and bacon age–depth model (Blaauw and Christen, 2011). The age–depth model is based on the tie point of mill construction at 1701 CE, indicated by a red symbol, calibrated radiocarbon ages are displayed as probability density functions of the 2σ distributions indicated by a blue symbol, and 137Cs ages are shown in green. (b) The 137Cs activity (peak area h−1 g−1 sediment).
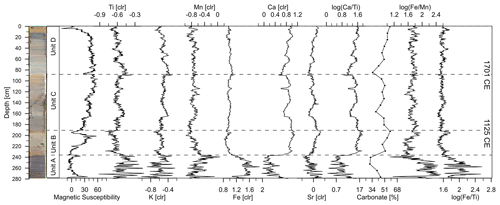
Figure 3Variations in magnetic susceptibility and selected elements as well as carbonate content [%] along the depth profile at Lake Höglwörth. Elemental data are shown as centred log ratios (clr).
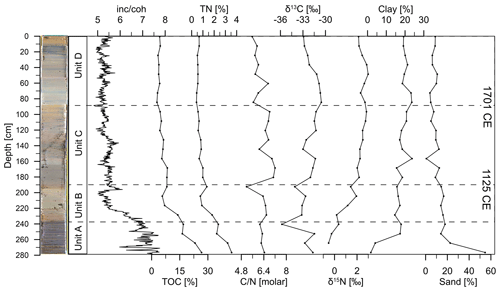
Figure 4Variations in inc coh, total organic carbon (TOC), total nitrogen (TN), the molar ratio of TOC [%] and TN [%] ( [molar]), and the bulk isotopic composition of organic carbon δ13C and nitrogen δ15N [‰] as well as sand [%] and clay [%] contents along the depth profile of Lake Höglwörth.
The 14C ages from the Lake Höglwörth sediment core are shown in Table 1. The calibrated median 14C ages range from 1620±120 BCE at 276 cm, to 2007±12 CE at 8 cm. The majority of the 14C ages from the Lake Höglwörth sediment core are in stratigraphic order (Fig. 2; Table 1). Only one sample at 67 cm depth shows an age reversal; however its error range overlaps with the age range of the sample at 92 cm depth (Fig. 2).
The 137Cs activity shows two peaks at 14 and 26 cm (Fig. 2). The first peak at 14 cm likely reflects the fallout from the 1986 CE Chernobyl accident, while the second peak at 26 cm likely reflects the fallout from the weapon testing in 1963 CE (Sirocko et al., 2013; Appleby et al., 1991; Appleby and Oldfield, 1978).
3.2 Geochemical analysis
Magnetic susceptibility values are low in Unit A and the lower part of Unit B. The upper part of Unit B reveals the maximum magnetic susceptibility of the entire record. After a significant drop at the transition from Unit B to Unit C, values remain on a quite constant high level with only minor fluctuations up to 20 cm composite depth. However, one minimum stands out at 88 cm depth, marking the transition to Unit D (Fig. 3). A trend towards lower values characterizes the top part of the core. The XRF analysis shows several changes in elemental records, associated with lithological changes (Fig. 3). Ti and K as proxies for allochthonous minerogenic input and catchment erosion (Strobel et al., 2021; Boes et al., 2011; Kastner et al., 2010) yield mostly the same pattern throughout the record, with high dynamic variability in Unit A but rather small variability in the following units. Ca and Sr show an opposite pattern compared to Ti and K. Ca and Sr are often linked to authigenic carbonate precipitation, e.g. due to enhanced lake productivity and/or evaporation (Kasper et al., 2012). The ratio can thus be used as a proxy for lake productivity versus minerogenic input. The very similar pattern of the ratio and CaCO3 of our record corroborates this interpretation (Fig. 3). Mn and Fe follow a very similar pattern with decreasing trends from Unit A towards Unit B, followed by persistently lower values. The ratio was proposed as a proxy for redox conditions due to the higher solubility of Mn in a reducing environment relative to Fe (Makri et al., 2021; Żarczyński et al., 2019). Higher values thus indicate a stratified water column or reducing conditions. The ratio might well be influenced by other processes such as diagenesis and detrital input (Makri et al., 2021). As during anoxia and when diagenetic processes are dominant within the sediments, both the Fe and the Mn are dissolved, while Ti remains a stable component (Aufgebauer et al., 2012; Boes et al., 2011). This would significantly alter the ratio compared to the ratio. Since this is not the case and both the ratios follow very similar trends, the effects of diagenesis and detrital input on the redox conditions derived from the ratio are supposed to be negligible in Lake Höglwörth (Evans et al., 2020; Kylander et al., 2013). Additionally, reconstructed anoxia based on is corroborated by the biological record discussed below. Despite the shift from Unit A to Unit B, ratios show only small changes.
TOC values range between 2.8 % and 26.6 %, exhibit the highest values in Unit A, and decrease towards Unit D. The inc coh ratio shows a quite similar pattern to TOC and thus can be used as a proxy for organic matter as well (Fortin et al., 2013; Guyard et al., 2007). TN values range from 3.5 % to 0.4 % and follow the pattern of TOC in the entire record (Fig. 4). The ratio ranges from 5.1 to 7.1 with an average value of 6.3, indicating that organic matter is primarily derived from autochthonous sources (Meyers, 2003). δ13C ranges from −34.1 ‰ to −30.5 ‰ and shows a pattern similar to the ratio (Fig. 4), implying organic matter is mainly authigenically derived. δ15N values vary between −0.2 ‰ and 3.0 ‰ and show an increasing trend from the bottom towards the top of the record.
3.3 Biological remains
Analysis of biological remains from the sediment core documents at least 30 different taxa from the sediment core (Fig. S4a–b, Table S1). Species level identification was achieved for Mollusca, Ostracoda, and Bryozoa. Other fossils encountered were oribatid mites, ephippia of cladocerans, beetle remains, fruits and seeds of angiosperm plants, oospores of charophytes, and fragments of mosses. Most taxa are aquatic, but there are a considerable number of terrestrial taxa as well.
Fossil remains are very rare in Unit A, suggesting unfavourable conditions for their preservation. Higher abundances in other units indicate better preservation. Broad maxima are visible in Units B and C for most taxa, suggesting higher deposition of fossil remains in these units. Glochidia do not appear before the upper part of Unit C and flourish in Unit D, whereas charophytes and Hippuris are abundant in Units B and C and disappear in Unit D.
Oribatid mites are highly abundant in Units B and C, whereas their abundance decreased in the earlier portion of Unit D. Ephippia are highly abundant in Unit B, but abundances decrease afterwards to the top.
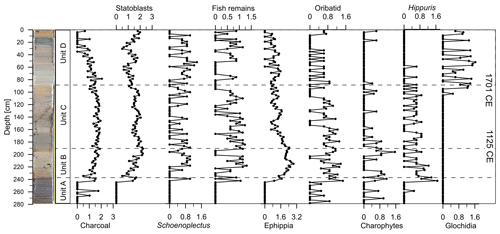
Figure 5Quantity of charcoal, statoblasts of freshwater bryozoans, Schoenoplectus, fish remains, ephippia of cladocerans, oribatid mites, charophyte oospores, Hippuris, and glochidia of unionid bivalves (log(individual species (x) + 1) 100 mL) along core depth.
Fish remains in the sediment show a broad maximum in the middle of Unit B, suggesting the start of and a substantial increase in the fish population in Lake Höglwörth with a short-lived breakdown at the limit to Unit C (Fig. 5).
Schoenoplectus is abundant in Units B and D but scarce in Unit C. Bryozoan statoblasts are relatively stable in Unit C, whereas they show a steady increase in the upper part of Unit D. Charcoal is very rare in Unit A, abundances are high in Units B and C, and they decrease throughout Unit D (Fig. 5).
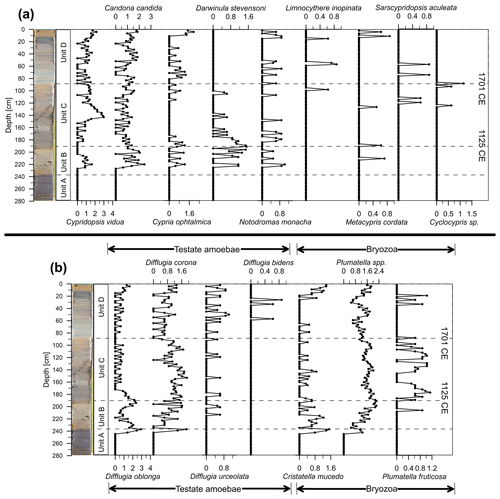
Figure 6(a) Distribution of ostracods and (b) testate amoebae and bryozoan statoblasts within the core from Lake Höglwörth. Ostracoda, testate amoebae, and Bryozoa abundances are given as abundance for 100 mL of sediment and are log-transformed [log (x+1)].
Dominant ostracod species are Cypridopsis vidua and Candona candida (Fig. 6a). Swimming ostracod species such as Cypridopsis vidua can avoid the oxygen deficiency of the bottom water by swimming in between macrophytes and are often found in highly productive waterbodies (Meisch, 2000). Their abundance is high at the middle of Unit C and in the later portion of Unit D. Cypria ophtalmica reaches high abundances in Units B and D but is almost absent from Unit C. Limnocythere inopinata, Sarscypridopsis aculeata, and Cyclocypris sp. are rare and restricted to the younger half of the core. In contrast, Darwinula stevensoni, known to live on mud in waterbodies such as fishponds (Meisch, 2000), is frequent in the older part of the core and vanishes in Unit D.
Testate amoebae can be found in the younger three units of the core in high abundances (Fig. 6b). There is a change recognizable from dominating Difflugia oblonga in basal Units C and B to prevailing Difflugia corona above in Unit D. Difflugia urceolata is a species that prefers cooler waters and can tolerate low oxygen contents as well (Scott et al., 2001). Difflugia urceolata and Difflugia bidens are rare but typical of Unit D.
Bryozoan statoblasts and most of the other fossil remains are missing from Unit A (Figs. 4–6). The dominating taxon is Plumatella spp., comprising the species Plumatella casmiana, Plumatella geimermassardi, and Plumatella repens, which are hard to discriminate based on badly preserved fossil material. Bryozoan statoblasts show highest abundances in Unit C and steadily increase in the upper part of Unit D (Fig. 5). The rarer species Plumatella fruticosa and Cristatella mucedo replace each other over the record: the former is typical of Units B and C, whereas the latter species is abundant in Unit B and the youngest part of the core. The zebra mussel Dreissena polymorpha appears in the upper 25 cm of the sediment core.
4.1 Chronostratigraphy
Unit A is characterized by organic-rich, low clay, and high sand contents and rare fossils. The calibrated 14C age in this unit is substantially higher (>2400 years) than the samples from other units, suggesting a very low accumulation rate or a discontinuous accumulation of the deposits (depositional gaps and/or erosion events). The lithological, geochemical, and biological records show a distinctly different depositional environment in Unit A compared to the other units. A local small wetland, peat, or a floodplain of a small creek with the presence of sedges and reeds is assumed. Since peat or floodplain deposits with presumably discontinuous character were not the primary focus of this study, the 14C age at 276 cm was excluded from age–depth modelling. For Units B to D, an age–depth model was calculated where during the first iteration, only the 137Cs and 14C ages were considered (Fig. S3). This reveals that the record roughly covers the last 1100 years. At 88 cm depth, where a distinct shift in lithology and ecological species as well as minerogenic input occurred, an age of CE is obtained. Considering the error range, this shift in the lacustrine environment matches the time frame of the flour mill construction and diversion of the input channel in 1701 CE. Therefore, we refined our chronology in a second iteration and included this age as a tie point for the final age–depth model (Fig. 2). It should be noted that the 14C age at 67 cm shows a slightly older age in both iterations, probably due to the input of older carbon as a result of enhanced minerogenic input after the channel diversion. However, this is still within the 2σ uncertainty range of the age–depth model (Fig. 2).
4.2 Stages of lake evolution
4.2.1 Pre-lake deposits prior to CE
The lowermost part of the sediment record represents most probably the pre-lake valley floor or a former wetland area (floodplain of the creek), with peat forming prior to the modern Lake Höglwörth. Authigenic carbonate precipitation was not favourable in this phase, as suggested by low contents of CaCO3 and ratios. Furthermore, the presence of preserved plant macro-remains and high ratios indicate anoxia that is typical in swamp/fen/peat bog environments. Several sandy layers suggest occasionally occurring flash-flooding events. Two peaks of charcoal suggest rare fire events in the area. The dominance of oribatid mites, with some rare findings of charophytes, implies a terrestrial or semi-aquatic habitat with dense vegetation and the presence of smaller waterbodies at this location (Frenzel, 2019).
4.2.2 From to 1120±120 CE
An abrupt change from high organic peat-like deposits to more silty deposits suggests increasing water levels that established a lacustrine environment after CE (Figs. 3–6). Fossils become much more abundant and diverse during this phase, and remains of many groups appear for the first time (e.g. statoblasts of bryozoans, Schoenoplectus, fish remains) or increase dramatically (e.g. Hippuris, oribatid mites). High counts of Cladocera, Difflugia oblonga, statoblasts, and ostracods such as Candona candida and Darwinula stevensoni characterize Lake Höglwörth as a shallow and macrophyte-rich waterbody (Fuhrmann, 2012; Meisch, 2000). In general, high abundances of ostracods suggest high aquatic production (Ruiz et al., 2013), which is further supported by increased carbonate precipitation. Decreasing TOC and TN values document a shift in nutrients, which is further supported by increasing δ15N and δ13C values (Meyers, 2003). Finer-grained sediments, a decrease in submerged macrophytes after CE, and a substantial increase in fish remains around CE points towards a slowly rising water level. A distinct increase in charcoal abundance infers increasing fire events in the lake catchment (Fig. 5).
The monastery Höglwörth on the lake peninsula was constructed in 1125 CE (Bauer and Bauer, 1993). During the construction phase, the peninsula was probably enlarged by soil material previously extracted from an artificial ditch around the construction area (Landesamt für Digitalisierung, 2022). In addition, the lake was probably dammed, but whether this was done before or during the construction of the monastery and when these activities happened are not known. Therefore, we cannot rule out that the sediments that accumulated between and 1120±120 CE result, at least partly, from these construction activities and/or the damming.
4.2.3 From 1120±120 to 1701 CE
Human activities in the lake catchment increased during this stage, as indicated by the charcoal content that likely reflects high levels of firewood burning (Whitlock and Larsen, 2001; Fig. 5). This is contemporaneous with an increase in human activities in the region (Gilck and Poschlod, 2020; Enters et al., 2006).
Decreasing abundance of statoblasts, ephippia, and charophytes, as well as lower diversity in ostracods and testate amoebae and increasing δ13C and δ15N values, points to an increased trophic state of the lake (Figs. 4–6; Poikane et al., 2018; Torres et al., 2012; Hartikainen et al., 2009).
In 1120±120 CE, enhanced flux of minerogenic material (Ti, K) was probably caused by the monastery construction in 1125 CE and would very likely have increased aquatic productivity (Haas et al., 2019). A further increase in minerogenic input from to CE associated with higher clay contents as well as increased values for magnetic susceptibility (Dearing et al., 1996) suggests increased soil erosion in the catchment of the lake (Fig. 3). This coincides with the massive medieval deforestation in Bavaria during the 13th century as a result of a growing population and increasing agropastoral activities (Gilck and Poschlod, 2020; van der Knaap et al., 2019; Enters et al., 2008). However, enhanced terrestrial input could also be related to a series of extreme precipitation and flood events such as the St Mary Magdalene flood in 1342/1343 (Bauch, 2019; Reinhardt-Imjela et al., 2018). During almost the same time (from to CE), anoxia occurred, as reflected by higher ratios and the abundance of Cypridopsis vidua and Difflugia urceolata because these species can avoid oxygen deficiency by swimming in between macrophytes (Fig. 7; Scott et al., 2001; Meisch, 2000). As the lake is relatively shallow and water might be seasonally mixed, the anoxia could mainly have occurred at the bottom of or in the interstitial water (Fam and Kerstin, 2018). In combination with bottom or interstitial water anoxia, abundances of ostracods as well as charophytes and pronounced calcite precipitation indicate overall enhanced aquatic productivity. Therefore, deforestation probably led to higher allochthonous input, which likely caused increased nutrient delivery. Similar processes were reported from other sites such as Lake Salęt in Poland, where anthropogenic activities during the medieval period led to stronger soil erosion and higher productivity (Gąsiorowski et al., 2021). Lacustrine carbonate precipitation ( ratios, CaCO3) is not only affected by biological productivity, but also driven by temperature and thus higher evaporation (Kasper et al., 2012; Mueller et al., 2009; Brown et al., 2007). Considering the chronological uncertainties, carbonate precipitation increased in Lake Höglwörth during periods of higher temperatures during the past millennium for Europe (PAGES 2k Consortium, 2013). Hence, increased biological production and higher temperatures might have been responsible for the authigenic carbonate precipitation in our record. The presence of glochidia, which are larval stages of unionid bivalves that live as parasites on fishes (Glöer, 2015), reflect the fact that abundant fish lived in Lake Höglwörth after CE. Their dominance within the uppermost, youngest part of the record might have been driven by fish aquaculture as practised by many monastery societies (Brugger et al., 2008).
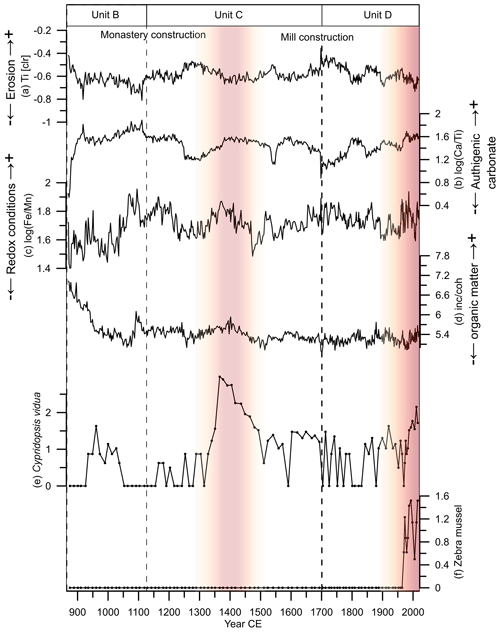
Figure 7Distribution of selected environmental proxies for the core from Lake Höglwörth. (a) The Ti centred log ratio (Ti [clr]) represents the minerogenic input; (b) indicates the authigenic carbonate precipitation; (c) reflects redox conditions; (d) inc coh refers to organic matter deposition. (e, f) Absolute abundance (log(x + 1) 100 mL−1) of Cypridopsis vidua and zebra mussels, respectively. Red shading indicates the periods with prevailing bottom or interstitial water anoxia in the lake as inferred from high and abundance of Cypridopsis vidua as well as other species mentioned in the text.
4.2.4 From 1701 to 2019 CE
High allochthonous input and a substantial shift in aquatic communities (e.g. disappearance of Plumatella fruticosa, Hippuris, Darwinula stevensoni, and charophytes and appearance of glochidia) around 1701 CE coincided with the construction of a flour mill and the related rerouting of the Höglwörther Seebach (Figs. 3–7; Gałczyńska et al., 2019; Melzer, 1999). An increased influx of water and minerogenic input, associated with the mill construction, might have affected lake water turbidity, water chemistry, and/or mixing regimens, consequently changing the aquatic fauna (Bhateria and Jain, 2016; Nilsson and Renöfält, 2008; Bunn and Arthington, 2002). Additionally, the production of the mill might have discharged wastewater into Lake Höglwörth, causing enrichment in nutrients such as N and P.
Enhanced carbonate precipitation is documented from to 2019 CE (Fig. 7). This was accompanied by a reduced minerogenic input and thus perhaps caused by increased primary productivity in the lake, leading to epilimnetic carbonate precipitation (Sun et al., 2019; Balci et al., 2018). Additionally, the post-industrial revolution rise in global temperature may have led to increased lake evaporation and thus enhanced carbonate precipitation (DWD Climate Data Center, 2023; IPCC, 2021; DWD Climate Data Center, 2020). The high abundance of Difflugia urceolata and Cypridopsis vidua after CE point towards anoxia in the lake (Fig. 7). The high abundance of the testate amoebae Difflugia urceolata and the appearance of Difflugia bidens after CE suggest a higher trophic state, possibly due to increased nutrient input from anthropogenic activities. This is corroborated by decreasing δ15N and δ13C values.
After around the 1960s, Lake Höglwörth was affected by eutrophication due to increased agriculture activities and the associated input of nutrients as inferred from decreasing δ15N values. This is in line with increased anthropogenic activities globally and the start of the industrial revolution in the region (Steffen et al., 2015; De Vries, 1994). Eutrophication is common for lakes in Bavaria (Enters et al., 2006; Bayrisches Landesamt, 1996) and central Europe (Bartram et al., 2002). The zebra mussel Dreissena polymorpha, a neozoon, an invasive species, and native to lakes in Russia and Ukraine, appeared in Lake Höglwörth around 1960±10 CE (Fig. 7; Neumann and Jenner, 1992). This is contemporaneous with its spread to alpine lakes (Minchin et al., 2002; Binder, 1965).
This study analysed a sediment core from Lake Höglwörth for lithology, geochemistry, and biology to investigate the palaeoenvironmental conditions and anthropogenic impact on a lacustrine system. Our findings suggest that a wetland environment (peat formation) existed prior to CE, when the lake sediment started to accumulate. Distinct shifts in sedimentology, elemental composition, and the biological record from to 1120±120 CE are attributed to the construction of the monastery in 1125 CE and/or damming of the lake. Our record documents increased allochthonous input from to CE related to enhanced soil erosion. Prevailing bottom or interstitial water anoxia from to CE can be related to higher nutrient input as a consequence of enhanced anthropogenic activities such as deforestation. A shift in the sedimentological and biological record at 1701 CE is possibly caused by the diversion of a small creek necessary for the operation of a newly built mill. Channel diversion increased the allochthonous input into the lake and had a significant impact on aquatic communities. Overall, this study shows that past human activities during the last millennium have had a significant impact on the lithological, geochemical, and biological environment, causing algal blooms and anoxia in Lake Höglwörth repeatedly during the last millennium prior to recent decades. Also, this study demonstrates that dam construction can have severe impacts on aquatic ecosystems. Lakes have undergone significant environmental changes in the past for various reasons, and understanding the causes and impacts of these changes can be valuable for predicting future ecological pathways as well as for restoration efforts.
The data presented in this paper can be found as an Excel dataset in the Supplement of this paper.
The supplement related to this article is available online at: https://doi.org/10.5194/egqsj-72-219-2023-supplement.
SA: conceptualization, formal analysis, investigation, discussions, writing (original draft, review and editing); MP: investigation, discussions, writing (review and editing); TK: fieldwork, writing (review and editing); LL: biological analyses and documentation, writing of a biological draft, writing (review and editing); PF: conceptualization and supervision of biological work, taxonomic identification, biological interpretation, co-writing of a biological draft, writing (review and editing); PS: conceptualization, investigation, discussions, fieldwork, writing (review and editing); MB: conceptualization, discussions, writing (review and editing); GD: fieldwork, writing (review and editing); CB: sample preparation, taxonomic identification, writing (review and editing); SS: writing (review and editing); GS: writing (review and editing); AS: funding acquisition, discussions, writing (review and editing); RZ: conceptualization, fieldwork, discussions, supervision, writing (review and editing).
The contact author has declared that none of the authors has any competing interests.
Publisher’s note: Copernicus Publications remains neutral with regard to jurisdictional claims made in the text, published maps, institutional affiliations, or any other geographical representation in this paper. While Copernicus Publications makes every effort to include appropriate place names, the final responsibility lies with the authors.
This study was funded by the German Research Foundation (DFG) via the International Research Training Group “Geo-ecosystems in Transition on the Tibetan Plateau (TransTiP)” (DFG grant 317513741/GRK 2309). We acknowledge support by the German Research Foundation, project no. 512648189, and the Open Access Publication Fund of the Thueringer Universitaets- und Landesbibliothek Jena. Maximilian Prochnow gratefully acknowledges the support of a fellowship from the state of Thuringia (Landesgraduiertenstipendium 2022). The authors want to thank Julian Struck (Friedrich Schiller University Jena) for contributions during discussions and help with lab work. Rafael Stiens is acknowledged for performing the XRF scanning at the University of Bremen. Jana Löhrlein, Theresa Henning, Moritz Mäding, and Josefin Sperling are thanked for their support in the laboratory. We thank associate editor Elisabeth Dietze and the two reviewers for their valuable and helpful comments that improved this paper.
This research has been supported by the German Research Foundation (DFG, grant no. 317513741/GRK 2309). Article processing charges were supported by the German Research Foundation (project no. 512648189) and the Open Access Publication Fund of the Thueringer Universitaets- und Landesbibliothek Jena.
This paper was edited by Elisabeth Dietze and reviewed by Christoph Mayr and one anonymous referee.
Adrian, R., O'Reilly, C. M., Zagarese, H., Baines, S. B., Hessen, D. O., Keller, W., Livingstone, D. M., Sommaruga, R., Straile, D., Van Donk, E., Weyhenmeyer, G. A., and Winder, M.: Lakes as sentinels of climate change, Limnol. Oceanogr., 54, 2283–2297, https://doi.org/10.4319/lo.2009.54.6_part_2.2283, 2009.
Alefs, J. and Müller, J.: Differences in the eutrophication dynamics of Ammersee and Starnberger See (Southern Germany), reflected by the diatom succession in varve-dated sediments, J. Paleolimnol., 21, 395–407, 1999.
Appleby, P. G. and Oldfield, F.: The calculation of lead-210 dates assuming a constant rate of supply of unsupported 210Pb to the sediment, Catena, 5, 1–8, https://doi.org/10.1016/S0341-8162(78)80002-2, 1978.
Appleby, P. G., Richardson, N., and Nolan, P. J.: 241Am dating of lake sediments, Hydrobiologia, 214, 35–42, https://doi.org/10.1007/BF00050929, 1991.
Aufgebauer, A., Panagiatopoulos, K., Wagner, B., Schaebitz, F., Viehberg, F. A., Vogel, H., Zanchetta, G., Sulpizio, R., Leng, M. J., and Damaschke, M.: Climate and environmental change over the last 17 ka recorded in sediments from Lake Prespa (Albania/F.Y.R. of Macedonia/Greece), Quatern. Int., 274, 122–135, https://doi.org/10.1016/j.quaint.2012.02.015, 2012.
Balci, N., Demirel, C., Akcer Ön, S., Gültekin, A. H., and Kurt, M. A.: Evaluating abiotic and microbial factors on carbonate precipitation in Lake Acigöl, a hypersaline lake in Southwestern Turkey, Quatern. Int., 486, 116–128, https://doi.org/10.1016/j.quaint.2017.12.046, 2018.
Bartram, J., Thyssen, N., and Gowers, A.: Water and health in Europe: a joint report from the European Environment Agency and the WHO Regional Office for Europe, WHO Regional Office for Europe, WHO Regional Publications European Series No. 93, ISBN 9289013605, 2002.
Bauch, M.: Die Magdalenenflut 1342 am Schnittpunkt von Umwelt- und Infrastrukturgeschichte: Ein compound event als Taktgeber für mittelalterliche Infrastrukturentwicklung und Daseinsvorsorge, NTM. Zeitschrift für Geschichte der Wissenschaften, Technik und Medizin, 27, 273–309, https://doi.org/10.1007/s00048-019-00221-y, 2019.
Bauer, H. and Bauer, A.: Klöster in Bayern: eine Kunst-und Kulturgeschichte der Klöster in Oberbayern, Niederbayern und der Oberpfalz, CH Beck, ISBN 3406377548, 1993.
Bayrisches Landesamt: Kostenbewußte Abwasserentsorgung, in: Informationsberichte des Bayrischen Landesamtes für Wasserwirtschaft, Bayrisches Landesamt für Wasserwirtschaft, Heft 2/96, 1996.
Bhateria, R. and Jain, D.: Water quality assessment of lake water: a review, Sustainable Water Resources Management, 2, 161–173, https://doi.org/10.1007/s40899-015-0014-7, 2016.
Binder, E.: Un mollusque envahissant, la Dreissena polymorpha, Musee de Geneve, 54, 2–4, 1965.
Blaauw, M. and Christen, J. A.: Flexible Paleoclimate Age-Depth Models Using an Autoregressive Gamma Process, Bayesian Anal., 6, 457–474, https://doi.org/10.1214/ba/1339616472, 2011.
Blei, J.: Die Saline Bad Reichenhall–Überlegungen zu herrschaftlichen Besitzverhältnissen und Handelsmechanismen von der Römerzeit bis zu den Bajuwaren, edited by: Herz, P., Schmid, P., and Stoll, O., Ökonomie und Politik, Facetten europäischer Geschichte im Imperium Romanum und dem frühen Mittelalter, RiU, 5, 151–170, 2011.
Blott, S. J. and Pye, K.: GRADISTAT: a grain size distribution and statistics package for the analysis of unconsolidated sediments, Earth Surf. Proc. Land., 26, 1237–1248, https://doi.org/10.1002/esp.261, 2001.
Boes, X., Rydberg, J., Martinez-Cortizas, A., Bindler, R., and Renberg, I.: Evaluation of conservative lithogenic elements (Ti, Zr, Al, and Rb) to study anthropogenic element enrichments in lake sediments, J. Paleolimnol., 46, 75–87, https://doi.org/10.1007/s10933-011-9515-z, 2011.
Brown, E. T., Johnson, T. C., Scholz, C. A., Cohen, A. S., and King, J. W.: Abrupt change in tropical African climate linked to the bipolar seesaw over the past 55,000 years, Geophys. Res. Lett., 34, L20702, https://doi.org/10.1029/2007gl031240, 2007.
Brugger, W., Dopsch, H., and Wild, J.: Höglwörth, Das Augustiner-Chorherrenstift mit den Pfarreien Anger und Piding, Verein Freunde der Salzburger Geschichte, ISBN 9783902582034, 2008.
Bunn, S. E. and Arthington, A. H.: Basic principles and ecological consequences of altered flow regimes for aquatic biodiversity, Environ. Manage., 30, 492–507, https://doi.org/10.1007/s00267-002-2737-0, 2002.
Crutzen, P. J. and Stoermer, E. F.: The “Anthropocene”(2000), in: Paul J. Crutzen and the Anthropocene: A New Epoch in Earth's History. The Anthropocene: Politik–Economics–Society–Science, edited bu: Benner, S., Lax, G., Crutzen, P. J., Pöschl, U., Lelieveld, J., and Brauch, H. G., Springer, Cham, https://doi.org/10.1007/978-3-030-82202-6_2, 2021.
Dearing, J. A., Dann, R., Hay, K., Lees, J., Loveland, P., Maher, B. A., and O'grady, K.: Frequency-dependent susceptibility measurements of environmental materials, Geophys. J. Int., 124, 228–240, https://doi.org/10.1111/j.1365-246X.1996.tb06366.x, 1996.
De Vries, J.: The industrial revolution and the industrious revolution, J Econ. Hist., 54, 249–270, https://doi.org/10.1017/S0022050700014467, 1994.
Dotterweich, M.: Land Use and Soil Erosion in northern Bavaria during the last 5000 Years, in: Long Term Hillslope and Fluvial System Modelling, Springer, 201–229, https://doi.org/10.1007/3-540-36606-7_11, 2003.
DWD Climate Data Center: Hohenpeißenberg Jahresmittel der Temperatur 1781–2022, https://www.dwd.de/DE/forschung/atmosphaerenbeob/zusammensetzung_atmosphaere/hohenpeissenberg/bild/lange_tempreihe.html (last access: 6 February 2023), 2023.
DWD Climate Data Center: Recent and historical dataset: Monthly means of precipitation totals, monthly mean air temperature and highest daily maximum temperature of a month for station Piding (CDC-ID 7424), DWD Climate Data Center [dataset], https://opendata.dwd.de/climate_environment/CDC/observations_germany/climate/multi_annual/mean_91-20/ (last access: 16 September 2022), 2020.
Enters, D., Lucke, A., and Zolitschka, B.: Effects of land-use change on deposition and composition of organic matter in Frickenhauser See, northern Bavaria, Germany, Sci. Total Environ., 369, 178–187, https://doi.org/10.1016/j.scitotenv.2006.05.020, 2006.
Enters, D., Dörfler, W., and Zolitschka, B.: Historical soil erosion and land-use change during the last two millennia recorded in lake sediments of Frickenhauser See, northern Bavaria, central Germany, The Holocene, 18, 243–254, https://doi.org/10.1177/0959683607086762, 2008.
Evans, G., Augustinus, P., Gadd, P., Zawadzki, A., Ditchfield, A., and Hopkins, J.: A multi-proxy paleoenvironmental interpretation spanning the last glacial cycle (ca. 117±8.5 ka BP) from a lake sediment stratigraphy from Lake Kai Iwi, Northland, New Zealand, J. Paleolimnol., 65, 101–122, https://doi.org/10.1007/s10933-020-00151-z, 2020.
Fam, C. and Kerstin, W.: Gewässerökologische Untersuchung des Höglwörther Sees, Gesellschaft für Landschaftsökologie, Gewässerbiologie & Umweltplanung mbH, 2018.
Fortin, D., Francus, P., Gebhardt, A. C., Hahn, A., Kliem, P., Lisé-Pronovost, A., Roychowdhury, R., Labrie, J., and St-Onge, G.: Destructive and non-destructive density determination: method comparison and evaluation from the Laguna Potrok Aike sedimentary record, Quaternary Sci. Rev., 71, 147–153, https://doi.org/10.1016/j.quascirev.2012.08.024, 2013.
Franz, G.: Der dreissigjährige Krieg und das deutsche Volk, De Gruyter Oldenbourg, https://doi.org/10.1515/9783110509328, 2019.
Frenzel, P.: Fossils of the southern Baltic Sea as palaeoenvironmental indicators in multi-proxy studies, Quatern. Int., 511, 6–21, https://doi.org/10.1016/j.quaint.2018.09.014, 2019.
Fuhrmann, R.: Atlas quartärer und rezenter Ostrakoden Mitteldeutschlands, Altenburger Naturwissenschaftliche Forschungen 15, Naturkundliches Museum Mauritianum Altenburg, 1–320, 2012.
Gałczyńska, M., Milke, J., Gamrat, R., and Stoltman, M.: Common mare's tail (Hippuris Vulgaris L.) in the assessment of water status and their phytoremediation, Folia Pomeranae Universitatis Technologiae Stetinensis Agricultura, Alimentaria, Piscaria et Zootechnica, 348, 57–70, https://doi.org/10.21005/aapz2019.49.1.06, 2019.
Gąsiorowski, M., Sienkiewicz, E., Ciołko, U., Kaucha, K., Kupryjanowicz, M., and Szal, M.: Cultural eutrophication of a Central European lowland lake from the Bronze Age to the present recorded in diatom and Cladocera remains, Catena, 204, 105404, https://doi.org/10.1016/j.catena.2021.105404, 2021.
Gilck, F. and Poschlod, P.: The history of human land use activities in the Northern Alps since the Neolithic Age. A reconstruction of vegetation and fire history in the Mangfall Mountains (Bavaria, Germany), The Holocene, 31, 579–591, https://doi.org/10.1177/0959683620981701, 2020.
Glöer, P.: Süßwassermollusken – ein Bestimmungsschlüssel für die Muscheln und Schnecken im Süßwasser der Bundesrepublik Deutschland, Deutscher Jugendbund für Naturbeobachtung, Augsburg, ISBN 9783923376025, 2015.
Glückert, G.: On Pleistocene glaciations in the German Alpine foreland, Bull. Geol. Soc. Finl., 46, 117–131, 1974.
Guyard, H., Chapron, E., St-Onge, G., Anselmetti, F. S., Arnaud, F., Magand, O., Francus, P., and Mélières, M.-A.: High-altitude varve records of abrupt environmental changes and mining activity over the last 4000 years in the Western French Alps (Lake Bramant, Grandes Rousses Massif), Quaternary Sci. Rev., 26, 2644–2660, https://doi.org/10.1016/j.quascirev.2007.07.007, 2007.
Haas, M., Baumann, F., Castella, D., Haghipour, N., Reusch, A., Strasser, M., Eglinton, T. I., and Dubois, N.: Roman-driven cultural eutrophication of Lake Murten, Switzerland, Earth Planet. Sc. Lett., 505, 110–117, https://doi.org/10.1016/j.epsl.2018.10.027, 2019.
Hartikainen, H., Johnes, P., Moncrieff, C., and Okamura, B.: Bryozoan populations reflect nutrient enrichment and productivity gradients in rivers, Freshwater Biol., 54, 2320–2334, https://doi.org/10.1111/j.1365-2427.2009.02262.x, 2009.
Hua, Q., Barbetti, M., and Rakowski, A. Z.: Atmospheric Radiocarbon for the Period 1950–2010, Radiocarbon, 55, 2059–2072, https://doi.org/10.2458/azu_js_rc.v55i2.16177, 2013.
IPCC: Climate Change 2021: The Physical Science Basis. Contribution of Working Group I to the Sixth Assessment Report of the Intergovernmental Panel on Climate Change, edited by: Masson-Delmotte, V., Zhai, P., Pirani, A., Connors, S. L., Péan, C., Berger, S., Caud, N., Chen, Y., Goldfarb, L., Gomis, M. I., Huang, M., Leitzell, K., Lonnoy, E., Matthews, J. B. R., Maycock, T. K., Waterfield, T., Yelekçi, O., Yu, R., and Zhou, B., Cambridge University Press, Cambridge, United Kingdom and New York, NY, USA, 2391 pp,, https://doi.org/10.1017/9781009157896, 2021.
Jäger, H.: Entwicklungsperioden agrarer Siedlungsgebiete im mittleren Westdeutschland seit dem frühen 13. Jahrhundert, Würzb. Geogr. Arb., 6, 1–136, 1958.
Kasper, T., Haberzettl, T., Doberschütz, S., Daut, G., Wang, J., Zhu, L., Nowaczyk, N., and Mäusbacher, R.: Indian Ocean Summer Monsoon (IOSM)-dynamics within the past 4 ka recorded in the sediments of Lake Nam Co, central Tibetan Plateau (China), Quaternary. Sci. Rev., 39, 73–85, https://doi.org/10.1016/j.quascirev.2012.02.011, 2012.
Kastner, S., Ohlendorf, C., Haberzettl, T., Lücke, A., Mayr, C., Maidana, N. I., Schäbitz, F., and Zolitschka, B.: Southern hemispheric westerlies control the spatial distribution of modern sediments in Laguna Potrok Aike, Argentina, J. Paleolimnol., 44, 887–902, https://doi.org/10.1007/s10933-010-9462-0, 2010.
Klausnitzer, B.: Stresemann – Exkursionsfauna von Deutschland – Bd. 1 Wirbellose (ohne Insekten), 9. Aufl., Springer Verlag, Berlin, 735 pp., ISBN 3662553538,, 2019.
Klein Goldewijk, K., Beusen, A., Van Drecht, G., and De Vos, M.: The HYDE 3.1 spatially explicit database of human-induced global land-use change over the past 12,000 years, Global Ecol. Biogeogr., 20, 73–86, https://doi.org/10.1111/j.1466-8238.2010.00587.x, 2011.
Küster, H.: Die Alpen: Geschichte einer Landschaft, CH Beck, ISBN 9783406748288, 2020.
Kylander, M. E., Klaminder, J., Wohlfarth, B., and Löwemark, L.: Geochemical responses to paleoclimatic changes in southern Sweden since the late glacial: the Hässeldala Port lake sediment record, J. Paleolimnol., 50, 57–70, https://doi.org/10.1007/s10933-013-9704-z, 2013.
Landesamt für Digitalisierung: Breitband und Vermessung, https://geoportal.bayern.de/bayernatlas/?zoom=12&lang=de&topic=ba&bgLayer=historisch&E=787743.03&catalogNodes=11 (last access: 23 June 2022), 2022.
Lauterbach, S., Brauer, A., Andersen, N., Danielopol, D. L., Dulski, P., Hüls, M., Milecka, K., Namiotko, T., Obremska, M., and Von Grafenstein, U.: Environmental responses to Lateglacial climatic fluctuations recorded in the sediments of pre-Alpine Lake Mondsee (northeastern Alps), J. Quaternary Sci., 26, 253–267, https://doi.org/10.1002/jqs.1448, 2011.
Lewis, S. L. and Maslin, M. A.: Defining the anthropocene, Nature, 519, 171–180, https://doi.org/10.1038/nature14258, 2015.
Makri, S., Wienhues, G., Bigalke, M., Gilli, A., Rey, F., Tinner, W., Vogel, H., and Grosjean, M.: Variations of sedimentary Fe and Mn fractions under changing lake mixing regimes, oxygenation and land surface processes during Late-glacial and Holocene times, Sci. Total Environ., 755, 143418, https://doi.org/10.1016/j.scitotenv.2020.143418, 2021.
Meisch, C.: Freshwater Ostracoda of western and central Europe, Sußwasserfauna von Mitteleuropa, 8/3, ISBN 9783827410016, 522 pp., 2000.
Melzer, A.: Aquatic macrophytes as tools for lake management, Hydrobiologia, 398, 182–190, https://doi.org/10.1023/A:1017001703033, 1999.
Meyers, P. A.: Applications of organic geochemistry to paleolimnological reconstructions: a summary of examples from the Laurentian Great Lakes, Org. Geochem., 34, 261–289, https://doi.org/10.1016/S0146-6380(02)00168-7, 2003.
Minchin, D., Lucy, F., and Sullivan, M.: Zebra Mussel: Impacts and Spread, in: Invasive Aquatic Species of Europe. Distribution, Impacts and Management, edited by: Leppäkoski, E., Gollasch, S., and Olenin, S., Springer, Dordrecht, 135–146, https://doi.org/10.1007/978-94-015-9956-6_15, 2002.
Mueller, A. D., Islebe, G. A., Hillesheim, M. B., Grzesik, D. A., Anselmetti, F. S., Ariztegui, D., Brenner, M., Curtis, J. H., Hodell, D. A., and Venz, K. A.: Climate drying and associated forest decline in the lowlands of northern Guatemala during the late Holocene, Quaternary Res., 71, 133–141, https://doi.org/10.1016/j.yqres.2008.10.002, 2009.
Neumann, D. and Jenner, H. A.: The zebra mussel Dreissena polymorpha: Ecology, Biological Monitoring and First Applications in the Water Quality Management, Schweizerbart science publishers, Stuttgart, ISBN 978-3-510-53002-1, 1992.
Nilsson, C. and Renöfält, B. M.: Linking Flow Regime and Water Quality in Rivers a Challenge to Adaptive Catchment Management, Ecol. Soc., 13, 18 pp., 2008.
PAGES 2k Consortium: Continental-scale temperature variability during the past two millennia, Nat. Geosci., 6, 339–346, https://doi.org/10.1038/ngeo1797, 2013.
Poikane, S., Portielje, R., Denys, L., Elferts, D., Kelly, M., Kolada, A., Maemets, H., Phillips, G., Sondergaard, M., Willby, N., and van den Berg, M. S.: Macrophyte assessment in European lakes: Diverse approaches but convergent views of 'good' ecological status, Ecol. Indic., 94, 185–197, https://doi.org/10.1016/j.ecolind.2018.06.056, 2018.
Ramisch, A., Tjallingii, R., Hartmann, K., Diekmann, B., and Brauer, A.: Echo of the Younger Dryas in Holocene Lake Sediments on the Tibetan Plateau, Geophys. Res. Lett., 45, 11154–11163, https://doi.org/10.1029/2018gl080225, 2018.
Reimer, P. J., Austin, W. E. N., Bard, E., Bayliss, A., Blackwell, P. G., Bronk Ramsey, C., Butzin, M., Cheng, H., Edwards, R. L., Friedrich, M., Grootes, P. M., Guilderson, T. P., Hajdas, I., Heaton, T. J., Hogg, A. G., Hughen, K. A., Kromer, B., Manning, S. W., Muscheler, R., Palmer, J. G., Pearson, C., van der Plicht, J., Reimer, R. W., Richards, D. A., Scott, E. M., Southon, J. R., Turney, C. S. M., Wacker, L., Adolphi, F., Büntgen, U., Capano, M., Fahrni, S. M., Fogtmann-Schulz, A., Friedrich, R., Köhler, P., Kudsk, S., Miyake, F., Olsen, J., Reinig, F., Sakamoto, M., Sookdeo, A., and Talamo, S.: The IntCal20 Northern Hemisphere Radiocarbon Age Calibration Curve (0–55 cal kBP), Radiocarbon, 62, 725–757, https://doi.org/10.1017/rdc.2020.41, 2020.
Reinhardt-Imjela, C., Imjela, R., Bölscher, J., and Schulte, A.: The impact of late medieval deforestation and 20th century forest decline on extreme flood magnitudes in the Ore Mountains (Southeastern Germany), Quatern. Int., 475, 42–53, https://doi.org/10.1016/j.quaint.2017.12.010, 2018.
Richard, V.: Paläolimnologische und vegetationsgeschichtliche Untersuchungen an Sedimenten aus Fuschlsee und Chiemsee (Salzburg und Bayern) [A study of the paleolimnology and vegetation history of sediments from lakes Fuschlsee and Chiemsee (Austria and Germany)], Schweizerbart Science Publishers, Stuttgart, Germany, ISBN 978-3-443-64182-5, 1996.
Rösch, M., Friedmann, A., Rieckhoff, S., Stojakowits, P., and Sudhaus, D.: A Late Würmian and Holocene pollen profile from Tüttensee, Upper Bavaria, as evidence of 15 Millennia of landscape history in the Chiemsee glacier region, Acta Palaeobotanica, 61, 136–147, https://doi.org/10.35535/acpa-2021-0008, 2021.
Ruddiman, W. F., He, F., Vavrus, S. J., and Kutzbach, J. E.: The early anthropogenic hypothesis: A review, Quaternary Sci. Rev., 240, 106386, https://doi.org/10.1016/j.quascirev.2020.106386, 2020.
Ruff, M., Fahrni, S., Gäggeler, H. W., Hajdas, I., Suter, M., Synal, H. A., Szidat, S., and Wacker, L.: On-line Radiocarbon Measurements of Small Samples Using Elemental Analyzer and MICADAS Gas Ion Source, Radiocarbon, 52, 1645–1656, https://doi.org/10.1017/s003382220005637x, 2010.
Ruiz, F., Abad, M., Bodergat, A. M., Carbonel, P., Rodríguez-Lázaro, J., González-Regalado, M. L., Toscano, A., García, E. X., and Prenda, J.: Freshwater ostracods as environmental tracers, Int. J. Environ. Sci. Te., 10, 1115–1128, https://doi.org/10.1007/s13762-013-0249-5, 2013.
Salazar, G., Zhang, Y. L., Agrios, K., and Szidat, S.: Development of a method for fast and automatic radiocarbon measurement of aerosol samples by online coupling of an elemental analyzer with a MICADAS AMS, Nuclear Instruments and Methods in Physics Research Section B: Beam Interactions with Materials and Atoms, 361, 163–167, https://doi.org/10.1016/j.nimb.2015.03.051, 2015.
Schönborn, W.: Beschalte Amöben:(Testacea), Ziemsen, Wittenberg-Lutherstadt, 1966.
Schubert, A., Lauterbach, S., Leipe, C., Scholz, V., Brauer, A., and Tarasov, P. E.: Anthropogenic and climate controls on vegetation changes between 1500 BCE and 500 CE reconstructed from a high-resolution pollen record from varved sediments of Lake Mondsee, Austria, Palaeogeogr. Palaeocl., 559, 109976, https://doi.org/10.1016/j.palaeo.2020.109976, 2020.
Scott, D. B., Medioli, F. S., and Schafer, E. T.: Monitoring in coastal environments using foraminifera and thecamoebian indicators, Cambridge University Press, ISBN 0-521-56173-6, 2001.
Sirocko, F., Dietrich, S., Veres, D., Grootes, P. M., Schaber-Mohr, K., Seelos, K., Nadeau, M.-J., Kromer, B., Rothacker, L., Röhner, M., Krbetschek, M., Appleby, P., Hambach, U., Rolf, C., Sudo, M., and Grim, S.: Multi-proxy dating of Holocene maar lakes and Pleistocene dry maar sediments in the Eifel, Germany, Quaternary Sci. Rev., 62, 56–76, https://doi.org/10.1016/j.quascirev.2012.09.011, 2013.
Steffen, W., Broadgate, W., Deutsch, L., Gaffney, O., and Ludwig, C.: The trajectory of the Anthropocene: The Great Acceleration, The Anthropocene Review, 2, 81–98, https://doi.org/10.1177/2053019614564785, 2015.
Strobel, P., Struck, J., Zech, R., and Bliedtner, M.: The spatial distribution of sedimentary compounds and their environmental implications in surface sediments of Lake Khar Nuur (Mongolian Altai), Earth Surf. Proc. Land., 46, 611–625, https://doi.org/10.1002/esp.5049, 2021.
Stuiver, M. and Reimer, P. J.: Extended 14C data base and revised CALIB 3.0 14C age calibration program, Radiocarbon, 35, 215–230, 1993.
Sun, D., He, Y., Wu, J., Liu, W., and Sun, Y.: Hydrological and Ecological Controls on Autochthonous Carbonate Deposition in Lake Systems: A Case Study From Lake Wuliangsu and the Global Perspective, Geophys. Res. Lett., 46, 6583–6593, https://doi.org/10.1029/2019gl082224, 2019.
Szidat, S., Salazar, G. A., Vogel, E., Battaglia, M., Wacker, L., Synal, H.-A., and Türler, A.: 14C analysis and sample preparation at the new Bern Laboratory for the Analysis of Radiocarbon with AMS (LARA), Radiocarbon, 56, 561–566, https://doi.org/10.2458/56.17457, 2014.
Torres, I. C., Inglett, P. W., Brenner, M., Kenney, W. F., and Ramesh Reddy, K.: Stable isotope (δ13C and δ15N) values of sediment organic matter in subtropical lakes of different trophic status, J. Paleolimnol., 47, 693–706, https://doi.org/10.1007/s10933-012-9593-6, 2012.
van der Knaap, W. O., van Leeuwen, J. F. N., Fahse, L., Szidat, S., Studer, T., Baumann, J., Heurich, M., and Tinner, W.: Vegetation and disturbance history of the Bavarian Forest National Park, Germany, Veg. Hist. Archaeobot., 29, 277–295, https://doi.org/10.1007/s00334-019-00742-5, 2019.
Waldner, W.: Salt Mining and Trading in the Berchtesgaden and Salzburg Area, in: Mineral Deposits of the Alps and of the Alpine Epoch in Europe, edited by: Schneider, H. J., Special Publication No. 3 of the Society for Geology Applied to Mineral Deposits, 3, Springer, Berlin, Heidelberg, https://doi.org/10.1007/978-3-642-68988-8_1, 1983.
Wasserwirtschaftsamt Traunstein: Höglwörther See: https://www.wwa-ts.bayern.de/fluesse_seen/gewaesserportraits/hoeglwoerther_see/index.htm (last access: 25 August 2022), 2018.
Waters, C. N. and Turner, S. D.: Defining the onset of the Anthropocene, Science, 378, 706–708, https://doi.org/10.1126/science.ade2310, 2022.
Waters, C. N., Zalasiewicz, J., Summerhayes, C., Barnosky, A. D., Poirier, C., Galuszka, A., Cearreta, A., Edgeworth, M., Ellis, E. C., Ellis, M., Jeandel, C., Leinfelder, R., McNeill, J. R., Richter, D., Steffen, W., Syvitski, J., Vidas, D., Wagreich, M., Williams, M., Zhisheng, A., Grinevald, J., Odada, E., Oreskes, N., and Wolfe, A. P.: The Anthropocene is functionally and stratigraphically distinct from the Holocene, Science, 351, aad2622, https://doi.org/10.1126/science.aad2622, 2016.
Weltje, G. J., Bloemsma, M. R., Tjallingii, R., Heslop, D., Röhl, U., and Croudace, I. W.: Prediction of Geochemical Composition from XRF Core Scanner Data: A New Multivariate Approach Including Automatic Selection of Calibration Samples and Quantification of Uncertainties, in: Micro-XRF Studies of Sediment Cores: Applications of a non-destructive tool for the environmental sciences, edited by: Croudace, I. W. and Rothwell, R. G., Springer Netherlands, Dordrecht, 507–534, https://doi.org/10.1007/978-94-017-9849-5_21, 2015.
Whitlock, C. and Larsen, C.: Charcoal as a fire proxy, in: Tracking Environmental Change Using Lake Sediments, in: Developments in Paleoenvironmental Research, edited by: Smol, J. P., Birks, H. J. B., Last, W. M., Bradley, R. S., and Alverson, K., Springer, Dordrecht, 3, 75–97, https://doi.org/10.1007/0-306-47668-1_5, 2001.
Żarczyński, M., Wacnik, A., and Tylmann, W.: Tracing lake mixing and oxygenation regime using the Fe/Mn ratio in varved sediments: 2000 year-long record of human-induced changes from Lake Żabińskie (NE Poland), Sci. Total Environ., 657, 585–596, https://doi.org/10.1016/j.scitotenv.2018.12.078, 2019.