the Creative Commons Attribution 4.0 License.
the Creative Commons Attribution 4.0 License.
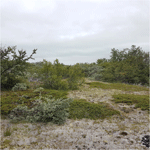
Diverse phenotypes of Late Glacial–Early Holocene downy birch (Betula pubescens Erh.) and the morphology of early Preboreal tree stands in southern Schleswig-Holstein
Krüger, S.: Diverse phenotypes of Late Glacial–Early Holocene downy birch (Betula pubescens Erh.) and the morphology of early Preboreal tree stands in southern Schleswig-Holstein, E&G Quaternary Sci. J., 73, 23–40, https://doi.org/10.5194/egqsj-73-23-2024, 2024.
The flexibility in growth of Betula pubescens is taken as example for possible erroneous imaginations of Late Glacial–Early Holocene living conditions. The article highlights the factors influencing the phenotype of the contemporary plants, as well as the small-scale patchiness of the distribution of the vegetation in the landscape.
The area of investigation is the southern Weichselian younger morainic area of Schleswig-Holstein, including a major Late Glacial archaeological type locality – the Ahrensburg tunnel valley. The period under investigation spans the time between 11 700 and 11 400 cal BP and hence the Late Glacial–Early Holocene transition, which is characterized by a significant shift in the vegetation. This shift has often been seen as a simple transition from treeless tundra to birch forest. The aim of the article is to provide a more realistic image of plant cover of the late Dryas 3 period and the Early Holocene period before the Preboreal oscillation by considering past natural growth limits of Betula pubescens in the study area. The intention is to provide a more accessible interpretation of palaeobotanical data to non-specialists, especially in archaeology.
Dieser Artikel beleuchtet die Flexibilität im Wuchs von Betula pubescens und hinterfragt damit mögliche Fehlvorstellungen von spätglazialen/frühholozänen Lebensbedingungen. Das Untersuchungsgebiet ist das südliche Weichseljungmoränengebiet Schleswig-Holsteins, inklusive einer bedeutenden spätglazialen archäologischen Typuslokalität – dem Ahrensburger Tunneltal. Der Fokus der Untersuchung umfasst die Zeit zwischen 11 700 und 11 400 cal BP und damit den Übergang vom Weichselspätglazial zum frühen Holozän, welcher durch eine deutliche Vegetationsveränderung gekennzeichnet ist. Diese Veränderung wurde bisher oft als schneller Wechsel von baumloser Tundra zu einem lichten Birkenwald gesehen, indem die Moorbirke (Betula pubescens) das dominierende Element darstellt. Der Artikel beleuchtet die Faktoren, die den Phänotyp der Moorbirke beeinflussen, und hebt die Uneinheitlichkeit und kleinräumige Vegetationsverteilung in der Landschaft hervor. Ziel des Artikels ist es, die oft vereinfacht dargestellte Vegetationsveränderung genauer zu untersuchen und ein realistischeres Bild der Flora der späten Dryas 3-Periode und des frühen Holozäns zu zeichnen. Dies geschieht, indem die natürlichen Wachstumsgrenzen von Betula pubescens mit rekonstruierten und gesammelten Daten aus dem Untersuchungsgebiet verglichen werden.
Zusätzlich wird mit einer Modellierung von Pollendaten ergründet, ob die Verwendung der Bezeichnung lichter Birkenwald weiterhin gerechtfertigt ist. Mit den Ergebnissen und dargestellten Wuchsformen soll anderen Disziplinen, insbesondere der Archäologie, eine leichter zugängliche Interpretation paläobotanischer Daten ermöglicht werden.
- Article
(9780 KB) - Full-text XML
-
Supplement
(1216 KB) - BibTeX
- EndNote
To consider palaeoenvironmental reconstructions in the understanding of Late Glacial–Early Holocene hunter-gatherer life conditions has for long been common practice in archaeology, especially in Schleswig-Holstein (Bokelmann, 1986; Schütrumpf, 1937, 1943; Usinger, 1998). Nevertheless, the information used to contextualize hunter-gatherers in their natural environment poses certain challenges. Among these challenges is the handling of the statements made by palaeobotany. The discipline of palaeobotany often intends to provide regional and over-regional overviews based on one or a synthesis of several investigations. This can lead to oversimplifications in the reconstruction of palaeovegetation, homogenizing vast areas into one type of vegetation cover.
The Late Glacial Younger Dryas vegetation is often described as a mainly treeless mosaic landscape dominated by shrub tundra, whereas the Early Holocene and Preboreal vegetation is regarded as a light or open birch forest (Behre, 1966, 2008; Weber et al., 2011). The vegetational history of the Late Glacial–Early Holocene transition is, however, a longer process that took place, even on a small-scale, in a variety of ways. The overall development from tundra to birch forest is an ecological gradient, which has been described as mosaic woodland, open birch woodland, or forest. The common perception of an Early Holocene forest rapidly replacing the tundra has been stated by various archaeobotanists and palynologists. Schütrumpf (1943) interpreted the pollen data from Stellmoor from the end of the Late Glacial as birch forests in the sense of “Lappish birch forests”, which are replaced by a “dense birch forest” in the Preboreal. Averdieck (1990) reported on a phase of “forest development” reflected by the beginning of the Preboreal or a rapid “closing of forest cover” (Averdieck, 1986). In more recent publications, Usinger (2004) further refers to Early Holocene vegetation in Schleswig-Holstein as “birch forest”, while Iversen (1973) mentions a rapid Early Holocene vegetation transforming into a “continuous forest” in the Danish region.
All these stated assumptions are understandable given the high Betula pollen values in the associated published pollen records. It is therefore likewise understandable why archaeologists like Weber et al. (2011) include the statement that “...at the transition Younger Dryas-Preboreal [...] light forests reappeared even in the northern lowland area ...” in their reconstruction of past living conditions. This perception is a popular and frequently used image of the landscape (Grimm et al., 2021b; Weber and Baales, 2013; Weber et al., 2011).
A differentiation between tundra plants and forest plants appears to be a straightforward distinction between crawling shrubs and trees. However, this is a misconception based on the comparison of contemporary shrubs and trees in optimal growing locations. Ultimately, this could result in the erroneous assumption that hunter-gatherers roamed and lived in treeless tundra landscapes of the Late Glacial or birch forests of the Early Holocene, similar to, for example, those found in Sweden (Västergötland) today.
But what actually is meant by “Preboreal forest” What would Early Holocene tree stands have looked like in the study area? To understand the most probable phenotype (observable traits) of single birch trees and the structure of tree stands in the Late Glacial and Early Holocene different controlling factors need to be considered. Decisive factors are temperature (growing season), soils and soil quality (nutrients), depth (permafrost), humidity and precipitation, and exposure (protection of growing location).
In this study, the aforementioned conditions are compiled on the basis of published data for the time period of the late Late Glacial and Early Holocene in southern Schleswig-Holstein. A comparison with corresponding conditions in modern subarctic regions (Norway, Greenland, Canada), where Betula pubescens is native, can provide an indication of the diversity of past growth forms of both individual birches and the character of birch stands and woodlands.
The aim of this paper is to give a more realistic impression of the phenotype of birches in southern Schleswig-Holstein, especially Betula pubescens, based on natural conditions and a more profound understanding of the development taking place during the Late Glacial–Early Holocene transitional phase. In addition, REVEALS (Regional Estimates of VEgetation Abundance from Large Sites) is applied to a high-resolution pollen dataset in order to trace the distribution of tree birches and their assumed dominance in the landscape. Together, this addresses the question of whether the description “light birch forest” would still be justified for the Early Holocene.
The study additionally aims to reduce misunderstandings between palaeobotany and archaeology and provide a more understandable translation of Late Glacial to Early Holocene palaeobotanical data and their interpretation.
The area of investigation is situated in the centre of the Weichselian younger morainic area of southern Schleswig-Holstein around the Nahe palaeolake (NAH), from which an existing high-resolution multi-proxy palaeoenvironmental record is available (Dreibrodt et al., 2020; Krüger, 2020; Krüger and Bogaard, 2020; Krüger et al., 2020).
This area covers a rather small region at the natural boundary of the eastern Weichselian and the Saalian moraines (Fig. 1a and b). This micro-region is used as an example of how diverse birches can grow, even in such a small area.
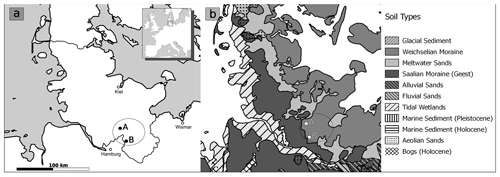
Figure 1(a) Map of modern Schleswig-Holstein and (b) soil types. The focus area is indicated within the black circle along with locations of the Nahe palaeolake (A) and the Ahrensburg tunnel valley (B).
The area of the Nahe palaeolake forms a geological unit with the area of the Ahrensburg tunnel valley. This is significant for this study as the latter is one of the major archaeological find areas dating from the Late Glacial and continuing into the beginning of the Holocene. Thus, human presence is evident in the focus area within the considered time span of vegetation change (Bratlund, 1999; Krüger, 2020; Rust, 1943).
The temporal setting of the study is given by the transition of the Late Glacial to the Early Holocene. The focus lies on the transition from the later part of the locally defined biostratigraphic Dryas 3 period to the reflection of the Preboreal oscillation (PBO) in the vegetation record – between 11 700 and 11 400 cal BP (Krüger et al., 2020).
3.1 Fieldwork
Assessment of different growth forms or phenotypes of Betula pubescens was conducted in southern Greenland in the summer of 2021 and 2022. Various individual plants and phenotypes were described and photographed and their growing conditions recorded at different sites in the vicinity of Narsarsuaq (61∘11′27.0′′ N, 45∘20'60.0′′ W), Qassiarsuk (61∘13′21.7′′ N, 45∘34'49.1′′ W), and Igaliku (61∘01′27.3′′ N, 45∘24'08.9′′ W). Further observations have been recorded in central Norway (61∘40′36.1′′ N, 7∘12′55.4′′ E) in late summer 2013.
3.2 Estimation of growth-limiting factors
Estimates for Late Glacial–Early Holocene growth-limiting factors such as permafrost, wind, and nutrient supply have been compiled on the basis of literature research and published records. Data on soils and geomorphology of southern Schleswig-Holstein are publicly available data from the Landesamt für Landwirtschaft, Umwelt und ländliche Räume Schleswig-Holstein (http://www.umweltdaten.landsh.de/atlas/script/index.php?thema=topo,geotope, last access: 8 November 2021).
The reconstruction of minimum mean July temperatures for the Late Glacial–Early Holocene is based on pollen data (Nahe palaeolake (NAH) – Krüger et al., 2020; Stellmoor (STM) – Hartmut Usinger, unpublished data) and macrofossil data (Usinger – Supplement Tables S1–S5) following the approach of Bos (1998) and Isarin and Bohncke (1999). The approximation of precipitation ratios for the Late Glacial–Early Holocene in southern Schleswig-Holstein is based on geo-statistics (Chandler et al., 2019), chironomid data (Pawłowski et al., 2015), and modelled precipitation data based on relations to present climate (Renssen, 2001).
Recent data of precipitation and temperature of comparison locations are publicly available on the portals of the respective national meteorological services – Norway (https://seklima.met.no/observations/, last access: 20 October 2021), Canada (https://climate.weather.gc.ca/historical_data/search_historic_data_e.html, last access: 20 October 2021), and Greenland (https://www.dmi.dk/vejrarkiv/normaler-gronland/, last access: 18 October 2021). In all three cases, the mean monthly precipitation and temperature of the last 30 years were calculated.
3.3 Legacy data
The previously unpublished macrofossil records of different sites analysed by Hartmut Usinger († 2015) were reviewed and are provided in the Supplement (Tables S1–S5). Usinger's palynological record from Stellmoor (STM), which is located in the Ahrensburg tunnel valley and used for temperature estimates, will be published separately.
3.4 Modelling of vegetation cover
Statistical analyses were carried out using R and RStudio (version 4.2.2) with the packages DISQOVER for calculating REVEALS in R (Theuerkauf et al., 2016). The pollen record from the Nahe palaeolake (Krüger et al., 2020) is used, with a minimum number of 550 counted total terrestrial pollen (TTP) per sample. Only pollen taxa with > 3 % of the TTP were used for the modelling of the vegetation cover. An overview on the pollen productivity estimates (PPEs) used and fall speed of pollen can be found in the Supplement (Table S6).
This section compiles the growth-limiting factors for Betula pubescens in southern Schleswig-Holstein, such as soils, landscape morphology, temperature and permafrost, humidity and precipitation, wind conditions, exposure, and nutrient supply. These are all described according to publicly available, published data and macrofossil data presented here for the Late Glacial and Early Holocene period.
Additionally, the study includes recent temperature and precipitation data from three chosen areas in the subarctic region of the Northern Hemisphere. Based on the comparison to recent sites and the reconstructed growth-limiting factors, it will then be determined whether, or in what way, habitat conditions affected the actual morphology of Late Glacial–Early Holocene Betula pubescens.
4.1 Soils and landscape morphology
The geomorphology of Schleswig-Holstein was shaped by the Saalian and Weichselian glaciations (Gripp, 1964; Woldstedt, 1935, 1954). The glaciations generally left four characteristic depositions that form a major component of the substrates that plants root into. These four characteristic depositions are listed according to their prevalence including main features (Fig. 2):
-
glacial deposits – till of ground moraines and terminal moraines; clayey, sandy, gravelly; boulder clay often overlying boulder marl due to leaching of limestone over time;
-
glaciofluvial deposits – sand, disordered gravel, glaciofluvial meltwater sands and gravels, and drainage valleys and kames (Woldstedt 1954); in places ice-margined; ice-margined with boulder clay interbeds;
-
glacilimnic deposits – silt, clay, partly fine sandy;
-
glacilimnic deposits – above boulder clay, fine sand, silty, in places over silt, clayey, sandy, gravelly (often above melted buried dead ice).
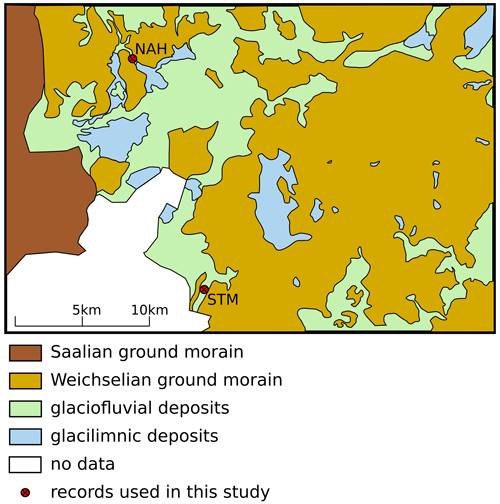
Figure 2Map of general soil types in the focus area. NAH – Nahe palaeolake; STM – Stellmoor and Ahrensburg tunnel valley.
Within the Weichselian ground moraines – within which the study area is embedded – the continuous melting process of the glaciers after the Late Glacial Maximum (∼ 23 000 BP) resulted in large amounts of buried dead ice as well as incision channels. In the course of the subsequent Glacial Interstadial 1 (GI-1; 14 700–12 840 BP) (Björck et al., 1998; Rasmussen et al., 2014), melting of the dead ice and the formation of kettle holes occurred extensively. Further, silting up processes and bog formation took place on different scales.
In the course of the following Glacial Stadial 1 (GS-1; 12 840–11 650 BP), erosion – usually an important factor of shaping the landscape during this time – played a minor role in the study area due to the closed vegetation cover of low-growing plants that had developed since the beginning of the GI-1 (Krüger et al., 2020). Aeolian sands were mainly deposited only to the west and north of the area of investigation (Dücker and Maarleveld, 1958; Kaiser and Clausen, 2005).
On a small scale, the morphology of the ground and terminal moraine and minor differences in elevation caused varying degrees of material deposition. Differences of only a few centimetres in layer thickness of, for example, sand or gravel can be decisive for the formation of soil. It cannot be estimated how much soil growth actually occurred as it is controlled by too many small- and large-scale factors. Nevertheless, it can be stated with certainty that boulder clay or boulder marl was a major component of the substrates in the study area and that the distribution of different substrate types can be characterized as highly fragmented even on a small scale.
4.2 Temperature and permafrost
Grace (1989) states that temperature is the most important factor controlling tree growth and fluctuations along the treeline. Local reconstructions of minimum mean July temperature – based on the minimum mean requirements of diverse species – suggests temperatures of around ≥ 10 ∘C during the biostratigraphic late Dryas 3 period in the study area (Table 1). In contrast, an increase in the minimum mean July temperature to ≥ 13 ∘C during the Early Holocene can be observed. This agrees with further observations, for example the presence of specific pollen types (Table 1) but also in the increase in local pollen precipitation (Averdieck, 1986; Björck et al., 1998; Usinger, 2004). This increase or sudden burst in pollen is inferred from a strong increase in pollen concentration and influx (Krüger et al., 2020).
Table 1Pollen types (nomenclature following Beug, 2004) and indicative minimum mean temperature from Bos (1998) and Isarin and Bohncke (1999). The datasets used are the Nahe palaeolake record (NAH; Krüger et al., 2020) and the Stellmoor record from the Ahrensburg tunnel valley (STM; H. Usinger, unpublished data). Presence or absence is indicated with x and –. As birch pollen separation was not carried out for the STM record, unavailable data are abbreviated with n.a. D3 signifies Dryas 3 period, and early PB signifies early Preboreal–Holocene period.
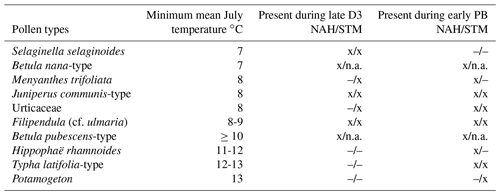
The temperature estimates based on NAH and STM agree with pollen-based reconstructions by Birks and Birks (2014) for the region but are generally lower than those based on chironomid data from Poland (Pawlowski, 2017; Pawłowski et al., 2015). An explanation for the lower estimates could be that the focus area is located much further west, that it is studied on a smaller scale, and/or that it was characterized by an oceanic rather than a continental climate during the biostratigraphic Dryas 3 period. The increased oceanity during the later Dryas 3 period is, for example, also reflected in the increase in Betula pubescens and Empetrum pollen values, both of which are well adapted to an oceanic climate (Usinger, 2004).
To avoid the circular argument of deriving the local minimum mean July temperature from pollen and inferring the presence of plants from the derived temperature, plant macrofossils must be considered. Macrofossils are the primary indicators of actual local plant presence. As can be seen from Table 2, there is evidence of actual Betula pubescens presence in the area of investigation, as well as from the wider surrounding region during the Dryas 3 period (Fig. 3). This supports the validity of the temperature approximations derived from pollen data.
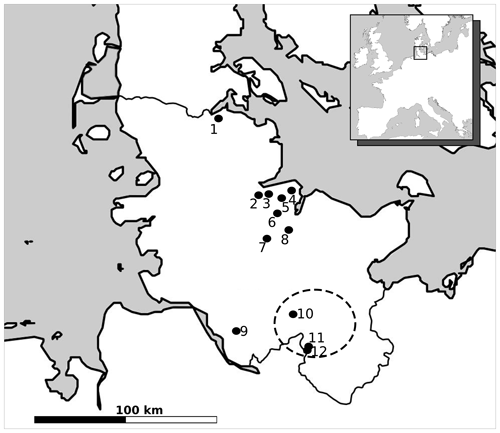
Figure 3Map of modern Schleswig-Holstein including macrofossil records of Betula pubescens from the biostratigraphic Dryas 3 period as mentioned in the text (1 – Blixmoor; 2 – Ruchmoor; 3 – Krögensmoor; 4 – Scharnhager Moor; 5 – Stauner Moor; 6 – Hasenmoor; 7 – Großes Moor/Dätgen; 8 – Kirchmoor; 9 – Esinger Moor; 10 – Nahe palaeolake; 11 – Stellmoor; 12 – Meiendorf). Dashed line indicates focus area.
Table 2Macrofossil finds and Betula pubescens fruits or nutlets in Schleswig-Holstein of the later Dryas 3 period based on palynostratigraphic dating. Sites marked with * indicate uncertain identification of Betula sp. or Betula alba. Locations are shown in Fig. 3.
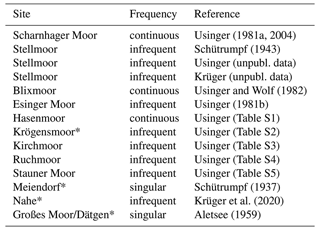
Permafrost
Crucial for the growth of plants during the Dryas 3 period was the depth at which the permafrost boundary was positioned. Permafrost formation during GS-1 in the area was rather discontinuous but can be assumed locally for longer periods, considering that the reconstructed mean annual temperature ranged between −4 and −8 ∘C (Isarin, 1997; Renssen and Vandenberghe, 2003). According to Delisle (1998), the permafrost boundary of the study area ranged roughly between 0.6 and 1.2 m depending on the proximity of waterbodies (Pielou, 2012). It is important to note that the study area is characterized by numerous waterbodies (kettle holes, incision lakes, water filled depressions, and channels) of Late Glacial origin (see Sect. 4.1 Soils and landscape morphology). It can therefore be reasoned that the permafrost boundary was probably very heterogeneously distributed.
The permafrost boundary decreased rapidly with the rising temperatures in the Holocene, and permafrost rapidly disappeared during the Early Holocene.
4.3 Humidity and precipitation
There are no reconstructions of the precipitation during the Dryas 3–Early Holocene transition in the area of investigation. Nevertheless, there are precipitation estimates based on geo-statistics (Chandler et al., 2019), chironomid data (Pawłowski et al., 2015), and modelled precipitation data based on relations to present climate (Renssen, 2001) within a wider northern European scale. Therein, a general west–east precipitation gradient is observable across the area with conditions of increasing continentality towards the east (Chandler et al., 2019).
Mean annual precipitation estimates range between 826±331 mm yr−1 in Scotland (Chandler et al., 2019), 731–802 mm yr−1 in the Netherlands (Renssen, 2001), and 400–500 mm yr−1 in central Poland (Pawłowski et al., 2015). Pawłowski et al. (2015) also calculated mean spring and summer precipitation estimates based on chironomid data for the later part of the Dryas 3 period in central Poland of about 270 to 340 mm yr−1. It is likely that comparable or potentially slightly higher values can be assumed for the study area for the Dryas 3 period.
The estimated precipitation rate per year also increased in northern central Europe in conjunction with the temperature rise of the Early Holocene (Rach et al., 2014). Models by Renssen (2001) showed a 14 % higher precipitation rate per year and an increased frequency of heavy rainfall. Chironomid data indicate higher precipitation of about 100 mm yr−1 for central Poland (Pawłowski et al., 2015).
Furthermore, a generally higher Early Holocene precipitation in the Netherlands, northern Germany and thus also the focus area relative to the Dryas 3 precipitations is revealed by inferred higher lake levels from multiple lake records (Usinger, 2004; Krüger et al., 2020; Bohncke and Wijmstra, 1988).
4.4 Wind conditions
A strong change in north Atlantic wind systems following the Laacher See eruption has been reconstructed based on different lake records, including the Meefelder Maar or the Nahe palaeolake (Brauer et al., 2008; Dreibrodt et al., 2020). The emerging southward shift in the westerlies had an ongoing effect throughout the Dryas 3 period. An intensification of the westerlies in northern central Europe during the Dryas 3 period was due to the strong cooling over the Atlantic and extended sea ice, which steepened the meridional temperature gradient (Renssen, 2001). Strong wind activity is evidenced by sand accumulation and dune formation north and west of the focus area (Kaiser and Clausen, 2005).
Coarse-grained material in the sediment sequence of the Dryas 3 in the Nahe palaeolake record, palynological indicators of redeposition, and secondary and tertiary charcoal highlight increased erosional processes in the focus area (Krüger et al., 2020). Especially the latter is assumed to reflect long-distance aeolian transport that was favoured by strong westerlies.
Although Isarin (1997) suggested that the aeolian processes occurred predominantly during winter, constant winds cannot be ruled out for the rest of the year due to the more southerly position of the sea ice boundary. This may have resulted in continuous wind stress for plants in exposed stands throughout the year during the Dryas 3 period.
In contrast, the rapidly northward-shifting sea ice boundary during the Early Holocene resulted in a calming of westerlies over the study area and, hence, in a likely reduction in wind stress.
4.5 Nutrient supply
Nutrient supply is one of the three major growth-limiting factors for modern Betula sp. at high latitudes along with temperature and precipitation (Boyle et al., 2022; Weih and Karlsson, 1997). While temperature and precipitation during the Late Glacial–Early Holocene can be approached via robust proxy data, the assessment of nutrients in the soil remains a matter of estimation.
The nutrient pools of a soil are a function of organic matter content. While past organic content or specific elements of soils cannot be accessed directly, a rough estimate may be possible via loss-on-ignition data and the distribution of indicative plant species.
For the late Dryas 3 period erosional proxies are still at elevated levels, and certain non-pollen palynomorph (NPP) types indicate large-scale rearrangement processes (Krüger et al., 2020). Therefore, it is not unreasonable to assume that the strong westerlies have removed large amounts of nutrient-rich humus which had developed during the previous Allerød period or that humus layers were covered by aeolian material (Kaiser and Clausen, 2005).
Pollen diagrams from along the present-day North Sea coast reveal that Empetrum (cf. nigrum) is a major component of the vegetation during the late Dryas 3 period. The spread of the species along the northern Atlantic seaboard, including the study area, during the late Dryas 3 period is associated both with an increasing oceanic climate and with the leaching of carbonates giving a low soil nutrient content (Behre, 1967; Hoek et al., 1999; Krüger et al., 2020; Overbeck, 1975; Usinger, 2004).
Furthermore, the organic sediment content in the Nahe palaeolake (Krüger et al., 2020), estimated by loss-on-ignition, is very low during the Dryas 3 period relative to mid-Holocene values. Assuming that the organic sediment content is the sum of organic production in the lake, material from aeolian input and surface water run-off, it can be inferred from the given high mineral content that a low organic content of the surrounding soils is reflected in the data for both the late Dryas 3 and the Early Holocene periods.
4.6 Proportion of the total vegetation of the Nahe palaeolake data (REVEALS)
REVEALS in R (Theuerkauf et al., 2016) is applied to the NAH dataset to model the proportions of the main species involved in the vegetation cover. The results are shown in Fig. 4.
In the studied time interval, a dominance of Empetrum between 60 % and 50 % of vegetation cover is modelled for the late Dryas 3 period (11 700 to about 11 600 cal BP; Fig. 4, period 1). Some photophilous (light-demanding) herbs, such as Ranunculus acris, Helianthemum, and Chenopodiaceae, but also Pinus, are represented with values around 10 %. The modelled values of both Betula nana and Betula pubescens are around or below 1 %.
For the Early Holocene (from about 11 600 cal BP until the beginning of the biostratigraphic PBO around 11 400 cal BP; Fig. 5, phase 2) Empetrum continues to dominate the modelled composition of vegetation with only slightly decreasing values. Only after the end of the studied time period do these values decrease strongly to less than 30 %. The values of Betula pubescens rise only slowly until about 11 500 cal BP and then increase rapidly from 5 % to about 20 %. Together with this increase, the values of Juniperus and Salix also rise. Pinus values remain almost stable around 10 %.
This discussion examines how and in what way the reconstructed growth-limiting factors influence the actual growth of Betula pubescens. In combination with the modelled data on vegetation cover, it is suggested which possible phenotypes of Betula pubescens were probable in the study area during the late Dryas 3 period and the Early Holocene. Furthermore, the question of whether it is justified to use the description “light birch forest” will be addressed on the basis of the presented data. Finally, the results are contextualised with regard to their archaeological importance.
5.1 Growth-limiting factors in relation to Betula pubescens
5.1.1 Effects of reconstructed soils and substrates on Betula pubescens growth
The composition of the soil and the depth into which roots can penetrate is critical to the establishment or growth heights of a number of plants. The growth height and ability to produce biomass of especially pioneer plants, such as Betula pubescens, is an important factor in the amount of organic material in the substrate. Accordingly, the proportion of organic material in the soil after deglaciation increased rapidly or slowly with dramatic local gradients as it is partly coupled to the spatially very diverse plant growth.
The combination of substrate and other abiotic factors such as temperature, exposure or sunlight can produce favourable Betula pubescens growth areas of different sizes, sometimes of only a few square metres. These favourable areas for growth were, for example, sunny, south-facing sites in the lee and on the slopes of moraines, the steeper slopes of incision lakes, and generally riparian areas alongside waterbodies and river courses that are abundant in the study area. This implies that there were diverse and highly patchy distributions of Betula pubescens but also plant species in general.
The patchiness in the quality of the substrate for plant growth developed gradually over GI-1, persisted in most cases in the vegetation cover of GS-1 and consequently determined plant habitus and distribution during the beginning of the Holocene.
Betula pubescens has few requirements of its environment as it is very flexible and tolerant towards the media into which it roots (Hytönen et al., 2014). Studies from Ireland have shown that a compact clay layer similar to boulder clay can have a negative effect on roots and act as a barrier (Nieuwenhuis and Barrett, 2002). The dominant substrate in the study area at the time period of investigation is boulder clay or boulder marl. It has a low pore volume of 30 %–35 %, is rather densely settled and thus it is difficult for plants to colonize by root growth (Mauer and Palátová, 2003).
The low pore volume and density of the clay forms a barrier for rainwater drainage and may lead to waterlogging but cannot prevent Betula pubescens growth. For example, Betula pubescens can adapt to waterlogging by temporarily forming more fine roots (Wang et al., 2016). The plant species is also able to form very shallow root systems with a rootstock developing a system of several major rhizomes of different size. The root system is not confined to particularly deep nor particularly shallow soils and wide roots and can be adapted to site conditions (Anschlag et al., 2008). Indeed, the distribution of Betula pubescens during the Late Glacial tends to be particularly associated with boulder clay substrates (Theuerkauf and Joosten, 2009).
However, assuming that root growth of the majority of roots occurs in the upper 40 cm (Mauer and Palátová, 2003), even a few centimetres are already sufficient for growth of Betula pubescens. Nevertheless, the rooting depth determines the resistance of the plant to extreme weather, such as wind in particular. Hence, tree growth was coupled to a rooting depth that exceeds way beyond a few centimetres.
5.1.2 Effects of reconstructed temperature and permafrost on Betula pubescens growth
According to Grace (1989) fluctuations in treeline are linked to temperature more than to any other factor. Numerous studies demonstrate that the treeline is related to mean summer temperature, which coincides roughly with the 10∘-July-isotherm (Grace and Norton, 1990; Moreau et al., 2020; Birks and Birks, 2014).
Based on the pollen-based temperature estimates for the Dryas 3 period, reconstructed temperatures in the study region meet the 10∘C July temperature minimum required for Betula pubescens, suggesting it would be possible for it to grow in this region and time period.
This is in line with several papers on the statistical separation of birch pollen types. In these, Usinger (1981b) and Usinger and Wolf (1982) state that, based on the high calculated proportion of Betula pubescens in the total birch pollen percentage, a presence of the species during Dryas 3 period is out of the question.
On the basis of plant macrofossil analysis, it must be assumed that Betula pubescens was present as a species during the Dryas 3 period in the study area and beyond. This assumption is consistent with the pollen-derived minimum mean July temperature, which in turn would allow species growth.
Nevertheless, proven presence does not mean presence as 25 m tall specimens like in optimal habitats (Rothmaler, 2017). Betula pubescens was evidently present as a species, but the phenotype is still a matter of discussion.
Temperature controls the growing season and hence largely controls wood growth (Paulsen et al., 2000; Elkington and Jones, 1974). During the Dryas 3 period the growing season was a very limited annual period in which temperatures were high enough for photosynthesis, allowing the plant to produce new plant tissues. In this context, wood was a luxury (Pielou, 2012). As demonstrated by Elkington and Jones (1974), the products of photosynthesis are primarily used to grow leaves in climate zones of short growing seasons similar to the conditions in southern Greenland today. Here, only very low growth rates for wood are achievable by plants. As a consequence, many leaves grow densely on the short branches and shoot axes and due to the permanent wind exposure they nestle to the ground without any remarkable growth over several years. Maximum heights of only 3–4 m are observed in very patchy and sheltered stands of southern Greenland.
The Early Holocene warming dramatically altered the duration of the growing season. Spring and autumn became warmer, there was probably fewer late spring frosts and autumn began later allowing for accelerated wood growth compared to the previous stadial phase (GS-1). However, it is likely that the increased temperatures affected plant growth to a larger extent than the lengthened growing season (Boyle et al., 2022). It is possible that many single and tall Betula pubescens shoots emerged within short periods of time.
Permafrost
The permafrost boundary in the study area ranged roughly between 0.6 and 1.2 m depending on the proximity of waterbodies during the Dryas 3 period (Delisle, 1998; Pielou, 2012). Even where permafrost was higher than 0.6 m below the surface, the effect on the root growth of Betula pubescens would be limited as the majority of rooting occurred above that depth, mainly in the active layer.
Taller individuals with deeper roots could only grow in very confined areas where the substrate was suitable and the waterbody pushed down the permafrost boundary – for example, along river courses or the shores of lakes.
The permafrost thawed rapidly during the Early Holocene and did not affect the growth or the phenotype of Betula pubescens during this period.
5.1.3 Effects of reconstructed and modern humidity and precipitation values on Betula pubescens growth
Betula pubescens is sensitive to summer drought (Dörfler, 2017; Kühn, 1981; Rothmaler, 2017) but this is highly dependent on the geological characteristics rather than annual/seasonal precipitation (Mortensen et al., 2014). Indeed, Betula pubescens growth appears to be little affected by precipitation. Studies in Iceland (Levanič and Eggertsson, 2008), Finland (Welling et al., 2004), and Sweden (Kullman, 1993) have shown that temperature is the significantly more decisive growth parameter (Boyle et al., 2022). Nevertheless, an approximate precipitation minimum can be inferred from the present distribution of the species in the subarctic regions of the Northern Hemisphere.
In order to do so, three comparative locations (Canada, Greenland, Norway) were chosen at which Betula pubescens is native. At all three sites, the minimum mean average temperature over the past 30 years ranged around 10 ∘C between June and August, with only July above 10 ∘C in each case (Fig. 5).
In the Eagle Plains area (Yukon, NW Canada) low-growing Betula pubescens individuals of shrub height thrive between white-spruce-dominated open landscapes. The mean annual precipitation of the last 30 years reveals values around 376 mm yr−1 with the major rainfall during the spring and summer months of around 240 mm yr−1.
In Narsarsuaq (Kujalleq, SW Greenland) diverse phenotypes of thriving Betula pubescens individuals can be observed ranging from low-growing, wind-exposed individuals of knee height to crooked individuals in sheltered sunny stands of 3–4 m height. Here, the mean annual precipitation of the last 30 years reveals values around 603 mm yr−1 with about half the rainfall during the spring and summer months of around 316 mm yr−1.
In Porsa (Troms og Finnmark, N Norway) heavily branched Betula pubescens individuals of maximum 3–4 m height are dispersed in the open landscape. At this location, the mean annual precipitation of the last 30 years reveals values around 803 mm yr−1 with less than half the precipitation occurring during the spring and summer months of around 311 mm yr−1.
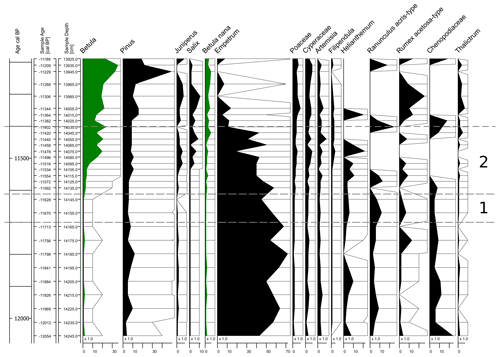
Figure 5Sequence of the Nahe palaeolake pollen dataset (Krüger et al., 2020) after applying REVEALS. Modelled Betula pubescens and Betula nana percentage values (share of total vegetation cover) are highlighted in green for improved reading. Time range in focus (11 700–11 400 cal BP) indicated by dashed lines. 1: Dryas 3 period; 2: Early Holocene before the PBO.
Given that the minimum mean temperature requirements of Betula pubescens is met at the three comparative locations and assuming that the decisive factor for growth in case of these three locations is precipitation, then a value significantly below 300 mm in the spring and summer months and only just below 400 mm on annual average, as in Eagle Plains, is still tolerable but a clearly growth-limiting factor. In the Greenlandic and Norwegian comparative regions, higher precipitation allows individuals of Betula pubescens to reach 3–4 m growth height and thus tree height in sheltered stands.
It follows that during Dryas 3, precipitation below 300 mm in the spring and summer months could have represented a growth limit for Betula pubescens. However, from the previous estimates and multi-proxy-based reconstructions, precipitation in the study area was likely just above the minimum requirements of Betula pubescens.
5.1.4 Effects of estimated wind conditions on Betula pubescens growth
Wind exposure and hence stress for plants varies significantly with respect to geomorphology even in the rather small area of investigation. Certainly, resistant tundra species like Betula nana, Empetrum nigrum, and Salix sp. formed low-growing or crawling stands in the open and windy terrains of the Dryas 3 period. Higher growth might have been limited not only by, for example, soil quality but also through abrasion processes resulting from winds. Exposed plants would be smaller and more compact with greater radial growth than sheltered plants (Gardiner et al., 2016). In the winter months small branches that protruded through the snow cover would have been eroded by windblown snow and ice crystals. In turn, aeolian sands could have had the same effect during the rest of the year under strong windy conditions. On less exposed stands constant wind could still have led to morphological adaptation resulting in bent or crooked phenotypes of naturally higher-growing plants as, for example, Betula pubescens. Generally, height growth is strongly limited by wind exposure. In glacial forelands Vetaas (1994) found that the highest growing and most mature individuals of Betula are found on the least wind exposed areas, indicating a clear abiotic control of growth height.
5.1.5 Effects of assumed nutrient supply on Betula pubescens growth
The capability to develop wood and a robust trunk is crucial for plant growth and would largely determine the phenotype of the plant. The main nutrients required for the annual production of Betula pubescens biomass are phosphorus, potassium, calcium, and nitrogen (Mälkönen, 1977), as well as carbon (Chapin III, 1989; Karlsson and Weih, 1996). Carbon and nitrogen are the principal nutrients required for wood formation (Chapin III, 1989; Karlsson and Weih, 1996; Weih and Karlsson, 1997).
Carbon is stored in plants as a product of photosynthesis, and also deadwood has always played a key role in the vegetation carbon cycle (Janisch and Harmon, 2002; Köster et al., 2015). Both carbon and nitrogen can be incorporated into the plant from decaying deadwood. Temperature is a limiting factor for nitrogen uptake as microbial activity affects the uptake rate through the root system (Cleve et al., 1981, 1990).
Mycorrhizal activity makes organic nitrogen available from above and below ground litter, and also dead animals and dung further contribute to nitrogen availability (Ritter, 2007). Therefore, grazing and browsing can increase the nitrogen abundance in an ecosystem through animal presence (Stark et al., 2007) but can likewise lead to large-scale defoliation that in turn limits wood formation and growth (Karlsson and Weih, 1996).
Also precipitation provides important amounts of nitrogen to the soil and, hence, to plants, which is vital for the nutrient resource balancing (Mälkönen, 1977). However, precipitation equally washes away nutrients.
Growth trials demonstrate that nitrogen accessibility is crucial in the first years of birch (wood) growth. A total of 44 % of nitrogen is released each growing season through leaf shedding of Betula pubescens and thus contributes to the nitrogen content of the newly forming soil (Karlsson and Nordell, 1987). Karlsson and Weih (1996) report that growth size is determined by nitrogen uptake through the root system. At a soil temperature of 15 ∘C, nitrogen uptake is 50 % higher than at a temperature of 10 ∘C and almost 10× higher than at 5 ∘C. This is strongly related to the microbial decomposition of soil litter (Cleve et al., 1981, 1990).
Generally nutrient-poor soil conditions can be assumed for the late Dryas 3 period in the study area.
In comparison, evidence for erosion (NPPs) and the proportion of Empetrum in the vegetation composition decreased rather moderately, indicating only slight shifts toward higher nutrient conditions during the Early Holocene. Nevertheless, it is likely that the growth height of single individuals (due to impaired wood production) and the further spread of the birches remained limited by the only gradually increasing nutrient availability.
Given that temperature, length of the vegetation period, precipitation, browsing pressure, wind speed, etc. all change rather quickly and promote improved growth of Betula pubescens at the onset of the Holocene (∼ 11 650 BP), nutrient (and especially nitrogen) availability could have been a potential bottleneck for tree-sized plant and consequently woodland or forest development in the Early Holocene.
5.2 Vegetation cover of the Late Glacial–Early Holocene transition in the study area
The composition and cover of vegetation during the Late Glacial–Early Holocene transition has often been oversimplified as a rapid shift from “treeless tundra” to “light birch forests” across the study area as well as across Schleswig-Holstein in general (Schütrumpf, 1943; Usinger, 2004; Weber et al., 2011). Securely dated palynological sequences covering this transitional period allowing modelling of the vegetation cover have only been available since 2020 (Krüger et al., 2020). As a result, this study presents the first model-based estimate of Late Glacial–Early Holocene vegetation cover for Schleswig-Holstein. This study is therefore the first to address the question of whether it is still justified to apply the term “light birch forest” based on modelled vegetation data in the region. The model also revealed different shares of plant species for the Dryas 3 period and the Early Holocene period than previously assumed.
For the biostratigraphic Dryas 3 period, a dominance of Betula nana was assumed regionally, alongside numerous grasses and forbs base on published pollen ratios and macrofossil data (Krüger et al., 2020; Averdieck, 1983; Usinger, 1975, 1981b, 2004). Further, a number of records indicate that Empetrum (cf. nigrum) witnessed a spread in areas of the contemporary North Sea coastline during the second half of the Dryas 3 period (Behre, 1967; Krüger et al., 2020; Overbeck, 1975). But the results of the present study reveal that Empetrum was in fact a landscape-dominating element with almost 70 % in the study area. In turn, Betula nana displays values below 5 %. These low numbers are unexpected considering the plant's proven presence reflected by high abundances of macrofossils (Tables S1–S5).
However, the values of Betula pubescens are below 5 % and thus within the expected range of distribution during the Dryas 3 period. The results in general describe a landscape that is dominated by tundra and heathland elements but with significantly different shares than previously thought (Behre, 2008; Krüger et al., 2020; Schütrumpf, 1943).
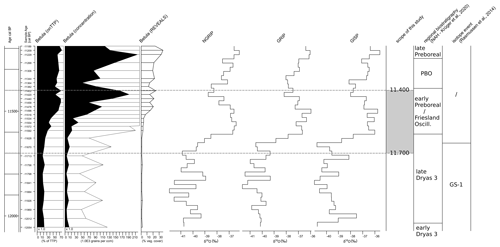
Figure 6Composite chart for comparison of Betula pubescens-type pollen values from the Nahe palaeolake pollen record (Krüger et al., 2020) calculated on (from left to right) total terrestrial pollen (TTP) and concentration values after applying REVEALS and Greenlandic oxygen isotope values (NGRIP, GRIP, GISP from Rasmussen et al., 2014). The scope of the study is indicated by the dashed lines.
The Early Holocene dispersal of Betula pubescens pollen along with the increase in its macrofossils (Krüger et al., 2020) is a clear reflection of temperature increase (Fig. 6). The temperature rise is also seen in the increasing modelled values of Filipendula and Juniperus. However, modelled Betula pubescens values only increase to about 20 %. Before the PBO (∼ 11 380) Empetrum (cf. nigrum) remained dominant. There may have been stands of, among others, Betula pubescens trees but not as dense woodlands or even forests. The presence of the low-growing and light-demanding Juniperus and Empetrum (cf. nigrum) of up to 10 % along with other light-demanding herbs and grasses does not suggest that it was a birch forest (Averdieck, 1986; Averdieck et al., 1990; Schütrumpf, 1943; Tybirk et al., 2000; Usinger, 2004), nor does it suggest that Betula pubescens was the dominating plant in the large catchment area of the Nahe palaeolake and, hence, the study area.
5.3 Speculative phenotypes of Betula pubescens between the late Dryas 3 period and the Early Holocene
Betula pubescens is extremely resistant and adaptable. This is evident from its growth on roofs and ruins, in sand pits, or in industrial wastelands and abandoned railroad station areas. Individuals of Betula pubescens can tolerate long periods of very poor conditions and can benefit from short climatic fluctuations (Kullman, 1984). Even where minimum requirements are barely met, there are individuals of 10–30 years of age and only a few decimetres in height. Kullman (1984) reports on frequent findings above the treeline of individuals which never reach tree height. Once Betula pubescens had migrated to a new area, the growth forms or phenotype depends on the factors listed above. The most decisive of these are (ranked in order of importance) temperature, soils (nutrients), and precipitation.
Modern landscapes and ecologies can be compared to the modelled estimates of each component in the Dryas 3 period and the transition to the Early Holocene. These areas can be found in the Narsarsuaq, Qassiarsuk, or Igaliku hinterlands in southern Greenland. The climate is classified as sub- or low-arctic and oceanic (Feilberg, 1984). Recent weather data from the past 30 years (Danish Meteorological Institute, DMI) give the minimum mean July temperature as just above 10 ∘C (11.2) and a mean annual precipitation of 603 mm yr−1 with about 316 mm precipitation in the spring and summer months. This region in southern Greenland therefore corresponds to the reconstructed Dryas 3 period in the study area regarding temperature and precipitation.
The soils of the Narsarsuaq, Qassiarsuk, and Igaliku areas are highly diverse and differ considerably in small areas. At most locations, a layer of boulder clay and gravel overlies the local bedrock. Here the plants grow in a few centimetres of substrate consisting of a gravel and bolder clay mixture partly overlaid by a 2–4 cm thick stratum of foliage and mosses. Fine aeolian sand is found in patches either with low-growing vegetation or in barren ground. In areas that are largely affected by spring melt, the subsoil mainly consists of compacted boulders of various sizes, mostly colonized by lichens and annual flowering plants (cf. Chamaenerion sp.). Due to the relief, there are geomorphologically favourable locations for the growth of, for example, Betula pubescens but also very exposed plains that are susceptible to the foehn winds. At such very exposed locations individuals of Betula pubescens nestle to the ground.
While the distinction between Betula nana and Betula pubescens based on fruit scales is straightforward, findings of macrofossils are by no means straightforward distinctions between shrub and tree. The creeping individuals of Betula pubescens that can be found in exposed areas in the Narsarsuaq, Qassiarsuk, and Igaliku hinterlands do produce fruit scales and nutlets of the same size as their tree-sized conspecifics (Peter Steen Henriksen, personal communication, 2 August 2021). Thus, if pollen and fruits of standard size of Betula pubescens are likewise produced by creeping individuals, it is not necessarily justified to assume actual tree-sized growth in the Late Glacial and Early Holocene.
The presence of the species even during the Dryas 3 period in the study area is suggested by the quantity of macrofossils, but the phenotype in which it could have occurred needs to be considered and remains speculative. This has implications for any representations of the vegetational landscape roamed by past hunter-gatherer societies.
5.3.1 Speculative phenotypes of Betula pubescens individuals during the late Dryas 3 period
During this period Betula pubescens individuals were most likely absent from exposed locations in the study area.
There would have been opportunities for growth in the lee of erratic boulders. Here, low shrub height was possible (Fig. 7b), but strong seasonal abrasion by wind-borne particles (sand/ice crystals) is to be expected and individuals were very likely to be short-lived (Fig. 7a).
The nutrient-poor soils of the region (leaching of carbonates indicated by the dominance of Empetrum nigrum in the region) and the temperature-driven short growing season generally resulted in limited annual growth of Betula pubescens. In places of high solar radiation with correspondingly high photosynthesis, it is possible that individuals which nestled close to the ground formed a thickened shoot axis. These could live for several decades (Fig. 7c and d).
Favourable growth locations for Betula pubescens included the southern-facing low moraines, steeper banks along incision lakes, kettle holes, and rivers. Due to protection from the wind and longer exposure to sunlight, these areas were more productive than the more exposed or shaded areas. Riparian zones are not expected to have occurred along the full length of waterbodies but rather at shorter stretches, depending on the orientation of the waterbody and its slopes.
Shrubs and individuals of Betula pubescens up to 2 m height were likely thriving in these growth-favouring areas. The shoot axes were probably very strongly curved or crooked and widely branched (Figs. 7e, f and S1). Single-stemmed individuals of taller growth height and straight branches and shoots are unlikely.
Growth of up to 2 m was only possible in limited growth-favouring locations due to several factors: wind-protected locations, long sun exposure and thus generally slightly higher temperatures, deeper root possibility due to lowering of the permafrost boundary by the waterbody, and possible higher nutrient supply near the river/lake due to higher bioproductivity.
To what extent several individuals also formed small-scale closed stands during the late Late Glacial cannot be estimated. However, the individuals of Betula pubescens during the Dryas 3 period can be described more accurately with the terms krummholz (German) or birkekrat (Danish) rather than with shrub or even tree.
5.3.2 Speculative phenotypes of Betula pubescens individuals and stands of trees during the Early Holocene period
The transition towards and the beginning of the Early Holocene was climatically by no means more stable than the Dryas 3 period but more favourable to the growth of Betula pubescens due to reduced wind activity, higher precipitation, increased temperatures, and a resulting prolonged growing season. Consequently, significantly increased organic production as well as soil and humus formation occurred across the study area. The higher air temperature for prolonged periods promoted plant flowering (including elevated pollen production) and thus improved reproduction. The result was an accelerated succession of generations.
It is conceivable that Betula pubescens still sporadically occurred as a shrub in very exposed locations (Fig. 8a). In many places in the landscape several individuals would have formed dense stands that gradually shaded out especially light-demanding forbs (cf. Helianthemum). In these dense stands, widely branched individuals without a main stem were dominant (Figs. 8c and d and S2–S4). They would have grown taller than during the Dryas 3 period, and single trees (individuals with one shoot axis and > 4 m height) would also be common within the dense stands (Fig. 8e and f). Whether they could grow higher than 6–8 m in the Early Holocene is questionable.
The temperature-controlled longer growing season relative to the Dryas 3 period and consequently increased reproduction caused fast-growing shoots of Betula pubescens. Within a few years they developed tall but very thin shoot axes (Fig. 8b). Their roots still had comparatively little opportunity to become established, and the individuals were probably often uprooted by wind.
During the Early Holocene, it was probably still the sunny and more sheltered places near waterbodies that offered more optimal growth conditions for Betula pubescens. Close to waterbodies, taller individual trees (6–8 m) would be likely, including also other tree species like Betula pendula, Salix sp., and Populus tremula1. This hypothesis is supported by the presence of the beaver, which requires tree growth and reappeared across northern central Europe during the Early Holocene (Aaris-Sørensen, 2009; Meadows et al., 2018; O'Connell et al., 2008).
It is evident that stands of Early Holocene tree species were not just pure accumulations of Betula pubescens individuals of differing heights but stands of different species. Also, these stands were not very close in character and could not shade the ground. As seen from the modelled pollen data (Fig. 4) and despite the presence of several tree species, the landscape remained open in character prior to the PBO. This is especially indicated by Juniperus. Juniperus would be creeping on the ground (Fig. 9a) during the Early Holocene and would be unlikely to be established if extensive stands of widely branched Betula pubescens individuals were shading the ground. Apart from Juniperus, there were other light-demanding plants like Empetrum (cf. nigrum; see Fig. 4) and many further grasses and forbs. In sum, these plants suggest an open and very fragmented character of the Early Holocene vegetation in the study area (Fig. 9b).
The patchy stands of different tree species including Betula pubescens cannot be described as “forest”. These small-scale stands of shrub work and woodland are probably numerous, partly continuous, but very open. There was just enough shade to outcompete Empetrum (cf. nigrum) and Helianthemum after 11 300 cal BP (see Fig. 4). The multiple stronger stems of Betula pubescens would mean these stands were impassable compared to the remaining landscape (Figs. S2–S4), which remained dominated by heath elements.
The connected but delayed animal shift from cold steppe and tundra fauna to forest animals is further evidence of the open character of the landscape that certainly persisted until after the PBO (around 11 380 cal BP).
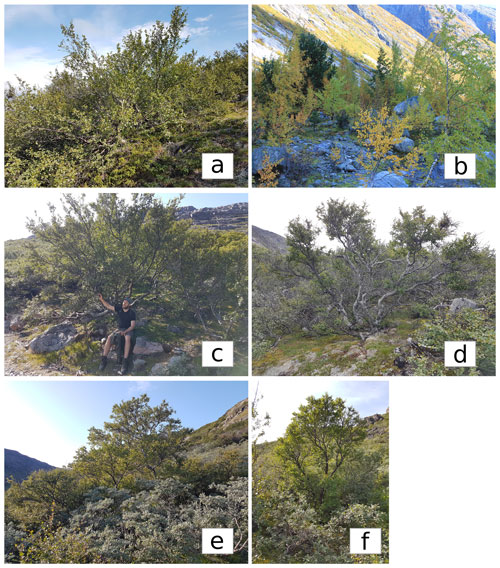
Figure 8(a)–(f) Photographs of Betula pubescens individuals in southern Greenland and Norway demonstrating reconstructed phenotypes for the Early Holocene period in the area of investigation. Pictures taken September 2013, July–August 2021, and July–August 2022 by the author.
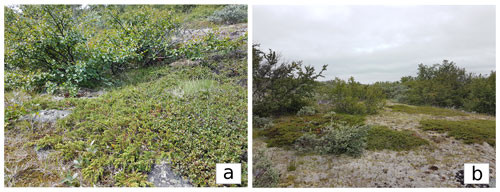
Figure 9(a) Picture of Betula pubescens (upper centre), Juniperus communis (lower centre), and Betula glandulosa (lower right) with representative growth heights (Greenland, July 2021, taken by the author). (b) Mosaic of the vegetation including open areas occupied by Juniperus communis and different lichen species and a dense shrub work of Betula pubescens and Salix glauca (Greenland, July 2021, taken by the author).
5.4 Transition of ecotones and its implication for archaeological reconstruction of human lives
The multi-proxy approach towards an understanding of the appearance of the vegetation structure and morphology highlights that a rapid transition from “treeless tundra” to “birch forest” across the study area is deceptive. The presented example-based explanation of probable ecotone morphology – which strongly contrasts with the often mentioned perception of an Early Holocene “light birch forest” – is certainly of value to archaeology, as archaeologists base their reconstructions of human life to a large extent on palaeobotany.
Two aspects are of crucial importance in gaining a more realistic picture of vegetation conditions during the late Dryas 3 and Early Holocene periods.
Firstly, during the late Dryas 3 period the tundra vegetation was by no means a treeless form of vegetation in the study area. Tree species were present in the aforementioned favourable growth locations. Betula pubescens individuals of < 2 m were present and useful as raw materials, fuel, or even landmarks. Further, the diversity of the tundra in the study area provided plant resources, such as the nutritious, sweet, and fat-rich crowberry (Empetrum nigrum) to a much greater extent than previously thought.
Secondly, hypothetical “birch forests” did not automatically spread with the temperature rise of the Early Holocene, as a quick glance at pollen diagrams might suggest. The temperature increases of the Early Holocene led to sudden bursts in pollen productivity. Pollen diagrams are calculated on total terrestrial plant pollen sums, and birches, especially Betula pubescens, produce large amounts of pollen, so pollen diagrams of this period can show a rather distorted picture of the actual conditions. These high Betula pollen values (routinely shown in published pollen ratio diagrams) are most likely the root of misunderstandings between palaeobotany/palynology and archaeology.
Referring to the Early Holocene vegetation as a “light birch forest” across the study area should therefore be avoided. In fact, Betula pubescens amounted only to maximum values of 20 % share of the total vegetation. In contrast, the dominant plant was still Empetrum (cf. nigrum) – a low-growing tundra element that also dominated the vegetation of the previous late Dryas 3 period. Just like the Dryas 3 period, the Early Holocene vegetation before the PBO continued to be a patchwork of many components. Tree-forming species were slowly spreading by the beginning of the Early Holocene, occupying more and more growth-favouring locations. Yet, most likely there were no large, extensive, and continuous woodlands in the study area but rather fragmented stands of shrub work and open woodlands (Fig. 9b).
The patchy stands of woody plants of diverse appearance structured the landscape in different ways. On the one hand, they provided shelter and resources, but on the other hand, they were very impassable (Figs. S2–S4) and might also have been perceived as a barrier by Late Glacial cultural groups, such as the Ahrensburgian, whose traditions persisted into the Early Holocene (Grimm et al., 2021a, b).
The pioneer species Betula pubescens (downy birch) is regarded as one key element of the vegetation shift from the Late Glacial to the Early Holocene in northern central Europe. As part of a strongly climate-controlled changing flora, Betula pubescens was exposed to various growth-controlling factors. The article discusses these growth-controlling factors with special focus on Betula pubescens and compiles corresponding regional reconstructed climatic scores. By using recent comparative sites and considering the reconstructed growth-limiting factors of the past, this study provides fact-based yet speculative phenotype descriptions of single individuals of Betula pubescens but also morphotypes of vegetation cover of the past across the study area during the late Dryas 3 period and the Early Holocene before the PBO, i.e. between ca. 11 700 and 11 400 cal BP.
Further, REVEALS is applied to a section of the Nahe pollen dataset (Krüger et al., 2020) covering the studied interval in order to estimate the actual contribution of Betula pubescens to the vegetation cover of the study area.
The overall results demonstrate why the often reproduced image of a rapid change from “treeless tundra” to “birch forest” should be avoided. The provided macrofossil data show that Betula pubescens as a species was in fact part of the tundra vegetation even during the late Dryas 3 period across the study area and further north. Even more importantly, the results highlight that tundra elements, especially Empetrum (cf. nigrum), dominated the vegetation of the whole investigated time interval, including the Early Holocene.
During the Early Holocene, different growth-controlling factors resulted in a few tree-sized (6–8 m) Betula pubescens individuals. Apart from Betula pubescens, the Early Holocene flora further included shrub and tree-sized individuals of Betula pendula, Salix sp., and Populus tremula, but their very fragmented stands were far from forming dense woodlands or forests.
Regarding hunter-gatherer societies, the very patchy vegetation that included fragmental stands of tree-sized individuals but mostly widely branched shrubs of Betula pubescens and other species in the landscape represented resources, on the one hand, but possibly likewise a barrier on the other that structured the still open landscape of the Early Holocene before the PBO.
The macrofossil data are available in the Supplement Tables S1–S5. Palynological data are available from the author upon reasonable request.
The supplement related to this article is available online at: https://doi.org/10.5194/egqsj-73-23-2024-supplement.
The author has declared that there are no competing interests.
Publisher’s note: Copernicus Publications remains neutral with regard to jurisdictional claims made in the text, published maps, institutional affiliations, or any other geographical representation in this paper. While Copernicus Publications makes every effort to include appropriate place names, the final responsibility lies with the authors.
Palynological investigations into sediments from the Nahe palaeolake (2017–2020) were part of the project B1 (Pioneers of the North, PI: B.V. Eriksen) of the CRC 1266, Scales of Transformation, funded by the German Research Foundation (DFG). Field observations in Greenland were made during summer fieldwork campaigns in Greenland in the frame of the project Activating Arctic Heritage: Exploring UNESCO World Heritage in Greenland funded by the Carlsberg Foundation.
I am thankful to Walter Dörfler for access to the Usinger Legacy data, Ruby Winter for digitalizing legacy data, Morten Fischer Mortensen and Berit V. Eriksen for comments on the manuscript, and also Catherine Jessen for improving the language.
This research has been supported by the Carlsbergfondet (CVR; grant no. 60223513).
This paper was edited by Ingmar Unkel and reviewed by two anonymous referees.
Aaris-Sørensen, K.: Diversity and dynamics of the mammalian fauna in Denmark throughout the last glacial-interglacial cycle, 115–0 kyr BP, Fossils and Strata, 57, 1–61, 2009.
Aletsee, L.: Zur Geschichte der Moore und Wälder des nördlichen Holsteins, Barth, Leipzig, Nova Acta Leopoldina NF 139, 3–51, 1959.
Anschlag, K., Broll, G., and Holtmeier, F.-K.: Mountain birch seedlings in the treeline ecotone, subarctic Finland: variation in above-and below-ground growth depending on microtopography, Arct. Antarct. Alp. Res., 40, 609–616, 2008.
Averdieck, F.-R.: Palynological investigations of the sediments of ten lakes in eastern Holstein, North Germany, Hydrobiologia, 103, 225–230, 1983.
Averdieck, F.-R.: Seen als Grundlage für palynologische Arbeiten in Schleswig-Holstein, in: Von der Eisenzeit zum Mittelalter. Siedlungsforschung auf Sylt, Angeln, Schwansen, Ostholstein, Mitteljütland, Bericht der Römisch-Germanischen Kommission, Mainz, Band 67, 514–528, 1986.
Averdieck, F.-R.: Untersuchungen zur Geobotanik bei Bad Oldesloe, Meyniana, 42, 115–122, 1990.
Behre, K.-E.: Untersuchungen zur spätglazialen und frühpostglazialen Vegetationsgeschichte Ostfrieslands, Eiszeitalter und Gegenwart, 17, 69–84, 1966.
Behre, K.-E.: The Late Glacial and Early Post Glacial History of Vegetation and Climate in Northwestern Germany, Rev. Palaeobot. Palyno., 4, 149–161, 1967.
Behre, K.-E.: Landschaftsgeschichte Norddeutschlands. Umwelt und Siedlung von der Steinzeit bis zur Gegenwart, Karl Wachholtz Verlag, Neumünster, 9–308, ISBN 978-3-529-02499-3, 2008.
Birks, H. H. and Birks, H. J. B.: To what extent did changes in July temperature influence Lateglacial vegetation patterns in NW Europe? Quaternary Sci. Rev., 106, 262–277, 2014.
Björck, S., Walker, M. J. C., Cwynar, L. C., Johnsen, S., Knudsen, K. L., Lowe, J. J., and Wohlfarth, B.: An event stratigraphy for the Last Termination in the North Atlantic region based on the Greenland ice-core record: a proposal by the INTIMATE group, J. Quaternary Sci., 13, 283–292, https://doi.org/10.1002/(SICI)1099-1417(199807/08)13:4<283::AID-JQS386>3.0.CO;2-A, 1998.
Bohncke, S. J. P. and Wijmstra, L.: reconstruction of Late-Glacial lake-level fluctuations in The Nethelands based on palaeobotanical analyses, geochemical results and pollen-density data, Boreas, 17, 403–425, https://doi.org/10.1111/j.1502-3885.1988.tb00971.x, 1988.
Bokelmann, K.: Rast unter Bäumen – Ein Ephemerer mesolithischer Lagerplatz aus dem Duvenseer Moor, Offa – Berichte und Mitteilungen zur Urgeschichte, Frühgeschichte und Mittelalterarchäologie, 43, 149–163, ISSN 0078-3714, 1986.
Bos, J. A. A.): Aspects of Lateglacial-Early Holocene Vegetation Development in Western Europe. Palynological and palaeobotanical investigations in Brabant (The Netherlands) and Hessen (Germany), LPP Contributions series No 10, Utrecht, 5–240, ISBN 90-393-1778-X, 1998.
Boyle, J. S., Angers-Blondin, S., Assmann, J. J., and Myers-Smith, I. H.: Summer temperature – but not growing season length – influences radial growth of Salix arctica in coastal Arctic tundra, Polar Biol., 45, 1257–1270, 2022.
Bratlund, B.: A revision of the rarer species from the Ahrensburgian assemblage from Stellmoor, in: The Holocene History of the European Vertebra Fauna. Modern Aspects of Research. Workshop, 6th to 9th April 1998, Berlin, edited by: Benecke, N., Archäologie in Eurasien. Band 6, Marie Leidorf GmbH, Rahden/Westf., 30–42, ISBN 3-89646-255-5, 1999.
Brauer, A., Haug, G. H., Dulski, P., Sigman, D. M., and Negendank, J. F. W.: An abrupt wind shift in western Europe at the onset of the Younger Dryas cold period, Nat. Geosci., 1, 520–523, https://doi.org/10.1038/ngeo263, 2008.
Chandler, B. M., Boston, C. M., and Lukas, S.: A spatially-restricted Younger Dryas plateau icefield in the Gaick, Scotland: reconstruction and palaeoclimatic implications, Quaternary Sci. Rev., 211, 107–135, 2019.
Chapin III, F. S.: The cost of tundra plant structures: evaluation of concepts and currencies, The American Nat., 133, 1–19, 1989.
Cleve, K. V., Barney, R., and Schlentner, R.: Evidence of temperature control of production and nutrient cycling in two interior Alaska black spruce ecosystems, Can. J. Forest Res., 11, 259–274, 1981.
Cleve, K. V., Oechel, W. C., and Hom, J. L.: Response of black spruce (Picea mariana) ecosystems to soil temperature modification in interior Alaska, Can. J. Forest Res., 20, 1530–1535, 1990.
Delisle, G.: Numerical simulation of permafrost growth and decay, J. Quaternary Sci.: Published for the Quaternary Research Association, 13, 325–333, 1998.
Dörfler, W.: Von der Dichte des naturnahen Waldes und den 'Flaschenhälsen' der Wildpopulation, in: Kontrapunkte. Festschrift für Manfred Rösch, edited by: Lechterbeck, J., and Fischer, E., Universitätsforschungen zur prähistorischen Archäologie, Rudolf Habelt Verlag GmbH, Bonn, 95–113, ISBN 978-3-7749-4081-9, 2017.
Dreibrodt, S., Krüger, S., Weber, J., and Feeser, I.: Limnological response to the Laacher See eruption ( LSE) in an annually laminated Allerød sediment sequence from the Nahe palaeolake, northern Germany, Boreas, 50, 167–183, https://doi.org/10.1111/bor.12468, 2020.
Dücker, A. and Maarleveld, G.: Hoch-und spätglaziale äolische Sande in Nordwest-deutschland und in den Niederlanden. Geol. Jb. 73, S. 215—234, 8 Abb., 5 Tab, 1958.
Elkington, T. and Jones, B.: Biomass and primary productivity of birch (Betula pubescens s. lat.) in south-west Greenland, The J. Ecol., 62, 821–830, https://doi.org/10.2307/2258957, 1974.
Feilberg, J.: A phytogeographical study of South Greenland. Vascular plants, Meddelelser om Grønland Bioscience, 15, 1–70, 1984.
Gardiner, B., Berry, P., and Moulia, B.: Wind impacts on plant growth, mechanics and damage, Plant Sci., 245, 94–118, 2016.
Grace, J.: Tree lines, Philosophical Transactions of the Royal Society of London. B, Biol. Sci., 324, 233–245, 1989.
Grace, J. and Norton, D.: Climate and growth of Pinus sylvestris at its upper altitudinal limit in Scotland: evidence from tree growth-rings, The J. Ecol., 78, 601–610, 1990.
Grimm, S. B., Groß, D., Gerken, K., and Weber, M.-J.: On the onset of the Early Mesolithic on the North German Plain Zum Beginn des Frühmesolithikums im norddeutschen Tiefland, in: Vom frühen Präboreal bis zum Subboreal - Aktuelle Forschungen zum Mesolithikum in Europa, edited by: Zander, A. and Gehlen, B., Studien zu Ehren von für Bernhard Gramsch, Edition Mesolithikum/Mesolithic Edition (Kerpen-Loogh 2020), 5, 11–33, 2021a.
Grimm, S. B., Eriksen, B. V., Krüger, S., Reuter, T., Wild, M., and Weber, M.-J.: Late Glacial occupation of northern Germany and adjacent areas. Revisiting the archives., in: The Beef behind all Possible Pasts. The Tandem-Festschrift in Honour of Elaine Turner and Martin Street, 157 ed., edited by: Gaudzinski-Windheuser, S., and Jöris, O., Monographien des RGZM, Mainz, 433–457, https://doi.org/10.11588/propylaeum.950.c12572, 2021b.
Gripp, K.: Die Erdgeschichte von Schleswig-Holstein, Karl Wachholtz Verlag, Neumünster, 411 pp., 1964.
Hoek, W. Z., Bohncke, S. J. P., Ganssen, G. M., and Meijer, T.: Lateglacial environmental changes recorded in calcareous gyttja deposits at Gulickshof, southern Netherlands, Boreas, 28, 416–432, ISSN 0300-9483, 1999.
Hytönen, J., Saramäki, J., and Niemistö, P.: Growth, stem quality and nutritional status of Betula pendula and Betula pubescens in pure stands and mixtures, Scan. J. Forest Res., 29, 1–11, 2014.
Isarin, R. F.: Permafrost distribution and temperatures in Europe during the Younger Dryas, Permafr. Perigl. Process., 8, 313–333, 1997.
Isarin, R. F. B. and Bohncke, S. J. P.: Mean July Temperatures during the Younger Dryas in Northwestern and Central Europe as Inferred from Climate Indicator Plant Species, Quarternary Res., 51, 158–173, 1999.
Janisch, J. and Harmon, M.: Successional changes in live and dead wood carbon stores: implications for net ecosystem productivity, Tree Physiol., 22, 77–89, 2002.
Kaiser, K. and Clausen, I.: Palaeopedology and Stratigraphy of the Late Palaeolithic Alt Duvenstedt Site, Schleswig-Holstein (Northwest Germany), Archäologisches Korrespondenzblatt, 35, 447–466, 2005.
Karlsson, P. and Nordell, K.: Growth of Betula pubescens and Pinus sylvestris seedlings in a subarctic environment, Funct. Ecol., 1, 37–44, 1987.
Karlsson, P. S. and Weih, M.: Relationships between nitrogen economy and performance in the mountain birch Betula pubescens ssp. tortuosa, Ecol. Bull., 45, 71–78, 1996.
Köster, K., Metslaid, M., Engelhart, J., and Köster, E.: Dead wood basic density, and the concentration of carbon and nitrogen for main tree species in managed hemiboreal forests, Forest Ecol. Manage., 354, 35–42, 2015.
Krüger, S.: Of birches, smoke and reindeer dung – Tracing human-environmental interactions palynologically in sediments from the Nahe palaeolake, J. Archaeol. Sci.-Rep., 32, 102370, https://doi.org/10.1016/j.jasrep.2020.102370, 2020.
Krüger, S. and Bogaard, C.: Small shards and long distances – three cryptotephra layers from the Nahe palaeolake including the first discovery of Laacher See Tephra in Schleswig-Holstein (Germany), J. Quaternary Sci., 36, 8–19, https://doi.org/10.1002/jqs.3264, 2020.
Krüger, S., Mortensen, M. F., and Dörfler, W.: Sequence completed – palynological investigations on Lateglacial/Early Holocene environmental changes recorded in sequentially laminated lacustrine sediments of the Nahe palaeolake in Schleswig-Holstein, Germany, Rev. Palaeobot. Palyno., 280, 104271, https://doi.org/10.1016/j.revpalbo.2020.104271, 2020.
Kühn, U.: Biologischer Atlas Schleswig-Holstein Lebensgemeinschaften des Landes, 263 pp., ISBN 3-529-05308-2, 1981.
Kullman, L.: Transplantation experiments with saplings of Betula pubescens ssp. tortuosa near the tree-limit in Central Sweden, Ecography, 7, 289–293, 1984.
Kullman, L.: Tree limit dynamics of Betula pubescens ssp. tortuosa in relation to climate variability: evidence from central Sweden, J. Veg. Sci., 4, 765–772, 1993.
Levanič, T. and Eggertsson, O.: Climatic effects on birch (Betula pubescens Ehrh.) growth in Fnjoskadalur valley, northern Iceland, Dendrochronologia, 25, 135–143, 2008.
Mälkönen, E.: Annual primary production and nutrient cycle in a birch stand, Metsäntutkimuslaitos, 1–35, ISSN: 0026-1610, 1977.
Mauer, O. and Palátová, E.: The role of root system in silver birch (Betula pendula Roth) dieback in the air-polluted area of Krušné hory Mts, J. Forest Sci., 5, 191–199, 2003.
Meadows, J., Robson, H. K., Groß, D., Hegge, C., Lübke, H., Schmölcke, U., Terberger, T., and Gramsch, B.: How fishy was the inland Mesolithic? New data from Friesack, Brandenburg, Germany, Radiocarbon, 60, 1621–1636, 2018.
Moreau, G., Chagnon, C., Auty, D., Caspersen, J., and Achim, A.: Impacts of Climatic Variation on the Growth of Black Spruce Across the Forest-Tundra Ecotone: Positive Effects of Warm Growing Seasons and Heat Waves Are Offset by Late Spring Frosts, Front. Forests Global Change, 145, 1–14, https://doi.org/10.3389/ffgc.2020.613523, 2020.
Mortensen, M. F., Henriksen, P. S., and Bennike, O.: Living on the good soil: relationships between soils, vegetation and human settlement during the late Allerød period in Denmark, Veg. Hist. Archaeobot., 23, 195–205, https://doi.org/10.1007/s00334-014-0433-7, 2014.
Nieuwenhuis, M. and Barrett, F.: The growth potential of downy birch (Betula pubescens (Ehrh.)) in Ireland, Forestry, 75, 75–87, 2002.
O'Connell, M., Atkinson, S., Gamez, K., Pickering, S., and Dutton, J.: Forage preferences of the European beaver Castor fiber: implications for re-introduction, Conserv. Soc., 6, 190–194, 2008.
Overbeck, F. T.: Botanisch-geologische Moorkunde unter besonderer Berucksichtigung der Moore Nordwestdeutschlands als Quellen zur Vegetations-, Klima- und Siedlungsgeschichte Wachholtz, Neumünster, 719 pp., 1975.
Paulsen, J., Weber, U., and Körner, C.: Tree growth near treeline: abrupt or gradual reduction with altitude?, Arct. Antarcti. Alpine Res., 32, 14–20, 2000.
Pawlowski, D.: The usefulness of subfossil Cladocera remains in Younger Dryas climatic reconstructions in central Poland, Acta Geol. Pol., 67, 567–584, 2017.
Pawłowski, D., Płóciennik, M., Brooks, S. J., Luoto, T. P., Milecka, K., Nevalainen, L., Peyron, O., Self, A., and Zieliński, T.): A multiproxy study of Younger Dryas and Early Holocene climatic conditions from the Grabia River paleo-oxbow lake (central Poland), Palaeogeogr. Palaeoclimatol. Palaeoecol., 438, 34–50, 2015.
Pielou, E. C.: A naturalist's guide to the Arctic, University of Chicago Press, 327 pp., ISBN-10: 0-226-66814-2, 2012.
Rach, O., Brauer, A., Wilkes, H., and Sachse, D.: Delayed hydrological response to Greenland cooling at the onset of the Younger Dryas in western Europe, Nat. Geosci., 7, 109–112, 2014.
Rasmussen, S. O., Bigler, M., Blockley, S. P., Blunier, T., Buchardt, S. L., Clausen, H. B., Cvijanovic, I., Dahl-Jensen, D., Johnsen, S. J., Fischer, H., Gkinis, V., Guillevic, M., Hoek, W. Z., Lowe, J. J., Pedro, J. B., Popp, T., Seierstad, I. K., Steffensen, J. P., Svensson, A. M., Vallelonga, P., Vinther, B. M., Walker, M. J. C., Wheatley, J. J., and Winstrup, M.: A stratigraphic framework for abrupt climatic changes during the Last Glacial period based on three synchronized Greenland ice-core records: refining and extending the INTIMATE event stratigraphy, Quaternary Sci. Rev., 106, 14–28, 2014.
Renssen, H.: The climate in The Netherlands during the Younger Dryas and Preboreal: means and extremes obtained with an atmospheric general circulation model, Neth. J. Geosci., 80, 19–30, 2001.
Renssen, H. and Vandenberghe, J.: Investigation of the relationship between permafrost distribution in NW Europe and extensive winter sea-ice cover in the North Atlantic Ocean during the cold phases of the Last Glaciation, Quaternary Sci. Rev., 22, 209–223, 2003.
Ritter, E.: Carbon, nitrogen and phosphorus in volcanic soils following afforestation with native birch (Betula pubescens) and introduced larch (Larix sibirica) in Iceland, Plant Soil, 295, 239–251, 2007.
Rothmaler, W.: Rothmaler – Exkursionsflora von Deutschland. Gefäßpflanzen: Atlasband, 5–822, https://doi.org/10.1007/978-3-662-49710-4, 2017.
Rust, A.: Die alt-und mittelsteinzeitlichen Funde von Stellmoor, K Wachholtz, Neumünster, 240 pp., 1943.
Schütrumpf, R.: Die Paläobotanisch-Pollenanalytische Untersuchung, in: Das Altsteinzeitliche Rentierjägerlager Meiendorf, edited by: Rust, A., Karl Wachholtz Verlag, Neumünster, 11–47, 1937.
Schütrumpf, R.: Die pollenanalytische Untersuchunge der Rentierjägerfundstelle Stellmoor in Holstein, in: Die alt- und mittelsteinzeitlichen Funde von Stellmoor, edited by: Rust, A., Karl Wachholtz Verlag, Neumünster, 6–45, 1943.
Stark, S., Julkunen-Tiitto, R., and Kumpula, J.: Ecological role of reindeer summer browsing in the mountain birch (Betula pubescens ssp. czerepanovii) forests: effects on plant defense, litter decomposition, and soil nutrient cycling, Oecologia, 151, 486–498, 2007.
Theuerkauf, M. and Joosten, H.: Substrate dependency of Lateglacial forests in north-east Germany: untangling vegetation patterns, ecological amplitudes and pollen dispersal in the past by downscaling regional pollen, J. Biogeogr., 36, 942–953, https://doi.org/10.1111/j.1365-2699.2008.02047.x, 2009.
Theuerkauf, M., Couwenberg, J., Kuparinen, A., and Liebscher, V.: A matter of dispersal: REVEALSinR introduces state-of-the-art dispersal models to quantitative vegetation reconstruction, Veg. Hist. Archaeobot., 25, 541–553, 2016.
Tybirk, K., Nilsson, M.-C., Michelsen, A., Kristensen, H. L., Shevtsova, A., Strandberg, M. T., Johansson, M., Nielsen, K. E., Riis-Nielsen, T., and Strandberg, B.: Nordic Empetrum dominated ecosystems: function and susceptibility to environmental changes, AMBIO: A J. Human Environ., 29, 90–97, 2000.
Usinger, H.: Pollenanalytische und stratigraphische Untersuchungen an zwei Spätglazial-Vorkommen in Schleswig-Holstein, Mitteilungen der Arbeitsgemeinschaft Geobotanik in Schleswig-Holstein und Hamburg, Heft 25, 183 pp., 1975.
Usinger, H.: Zur spät-und frühpostglazialen Vegetationsgeschichte der schleswig-holsteinischen Geest nach dem Pollen und Pollendichtediagramm aus dem Esinger Moor, Pollen et Spores, XXIII, 389–433, ISSN 0375-9636, 1981a.
Usinger, H.: Pollen- und Großestanalysen an limnischem Spätglazial aus dem Scharnhagener Moor, Schleswig-Holstein, Schriften Naturwissenschaftlicher Verein Schleswig-Holstein, 51, 85–105, 1981b.
Usinger, H.: Pollenanalytische Datierung spätpaläolithischer Fundschichten bei Ahrenshöft, Kr. Nordfriesland, Archäologische Nachrichten aus Schleswig-Holstein, 8, 24, 1998.
Usinger, H.: Vegetation and climate of the lowlands of northern Central Europe and adjacent areas around the Younger Dryas - Preboreal transition - with special emphasis on the Preboreal oscillation, Workshop of the U.I.S.P.P.-Commission XXXII, Greifswald, 1–26, ISSN: 1434-6427, 2004.
Usinger, H. and Wolf, A.: Zur vegetations- und klimageschichtlichen Gliederung des Alleröds nach Untersuchungen im Blixmoor und Kubitzbergmoor (Schleswig-Holstein), Schriften Naturwissenschaftlicher Verein Schleswig-Holstein, 52, 29–45, 1982.
Vetaas, O. R.: Primary succession of plant assemblages on a glacier foreland-Bodalsbreen, southern Norway, J. Biogeogr., 21, 297–308, 1994.
Wang, A.-F., Roitto, M., Sutinen, S., Lehto, T., Heinonen, J., Zhang, G., and Repo, T.: Waterlogging in late dormancy and the early growth phase affected root and leaf morphology in Betula pendula and Betula pubescens seedlings, Tree Physiol., 36, 86–98, 2016.
Weber, M.-J. and Baales, M.: Reiche Jagdgründe am Ende der Eiszeit, Archäologie in Deutschland, 26–29, ISSN: 0176-8522, 2013.
Weber, M.-J., Grimm, S. B., and Baales, M.: Between warm and cold: Impact of the Younger Dryas on human behavior in Central Europe, Quaternary Int., 242, 277–301, https://doi.org/10.1016/j.quaint.2010.12.002, 2011.
Weih, M. and Karlsson, P. S.: Growth and nitrogen utilization in seedlings of mountain birch (Betula pubescens ssp. tortuosa) as related to plant nitrogen status and temperature: a two-year study, Ecoscience, 4, 365–373, 1997.
Welling, A., Rinne, P., Viherä-Aarnio, A., Kontunen-Soppela, S., Heino, P., and Palva, E. T.: Photoperiod and temperature differentially regulate the expression of two dehydrin genes during overwintering of birch (Betula pubescens Ehrh.), J. Experiment. Botany, 55, 507–516, 2004.
Woldstedt, P.: Erläuterungen zur Geologisch-morphologischen Übersichtskarte des norddeutschen Vereisungsgebietes im Maßstab 1:1500000, Preußisch Geologische Landesanstalt, Berlin, 33 pp., 1935.
Woldstedt, P.: Das Eiszeitalter. Grundlinien einer Geologie des Quartärs, Erster Band. Die Allgemeinen Erscheinungen des Eiszeitalters, Ferdinand Enke Verlag, Stuttgart, 374 pp., 1954.
Salix sp. reaches shares of >10 % when applying REVEALS to the NAH dataset. Populus tremula was only scarcely present in the pollen record and hence excluded from the model. The scarcity could be explained by the bad pollen preservation of samples in the NAH record, whereas Populus pollen is present in the STM pollen record (Usinger unpubl.). Further, a piece of wood identified as Populus tremula from the NAH sediment core has been dated to the Dryas 3 period and, hence, indicates its early presence in the area (Krüger et al., 2020). Macrofossils of Populus tremula are regularly found alongside Betula pubescens during the Dryas 3 period as well as the Preboreal period (see Supplement).